Trans. Nonferrous Met. Soc. China 26(2016) 620-626
Effects of magnetic field and hot rolling on microstructures and properties of cryoECAPed 1050 aluminum alloy during annealing
Yi-heng CAO1, Li-zi HE1, Lin ZHANG2, Yi-zhou ZHOU3, Ping WANG1, Jian-zhong CUI1
1. Key Laboratory of Electromagnetic Processing of Materials, Ministry of Education, Northeastern University, Shenyang 110819, China;
2. School of Materials Science and Engineering, Northeastern University, Shenyang 110819, China;
3. Institute of Metal Research, Chinese Academy of Sciences, Shenyang 110016, China
Received 3 April 2015; accepted 14 September 2015
Abstract: The evolution of hardness and microstructures of 1050 aluminum alloy prepared by hot rolling and subsequent equal- channel angular pressing at cryogenic temperature (cryoECAP) after annealing at 150-400 °C for 1 h without and with magnetic field of 12 T was investigated. The electron back scattering diffraction pattern (EBSD) and transmission electron microscopy (TEM) were utilized to characterize the grain microstructures and dislocations. It is demonstrated that the hot rolling before cryoECAP produces more equiaxed grains with a smaller average size and a higher fraction of high angle boundaries (HABs) in the subsequent cryoECAPed 1050 aluminum alloy, thus accelerating the recovery and recrystallization of cryoECAPed alloy and produces more homogeneous microstructure during annealing. The magnetic field promotes the recovery and recrystallization and leads to much lower hardness at 150-250 °C, while it can suppress the abnormal grain growth and form more homogeneous grain size distributions annealed at 300-400 °C.
Key words: 1050 aluminum alloy; magnetic annealing; hot rolling; cryoECAP; hardness; grain growth
1 Introduction
Considerable attention has been paid on equal- channel angular pressing (ECAP) due to its ability to produce ultrafine-grained bulk samples having improved properties under a fully dense condition without changing the cross-sectional dimensions. It is well established now that, for large strains, continual plasticity plays a key role in the microstructural evolution and should be carefully evaluated [1-4]. In the last decades, the researches of ECAP have mostly been focused on the effects of the number of pressing, extrusion route and die shape, etc. [5-7], while the reports on the microstructures and properties during heat treatment are limited [8-11]. HASEGAWA et al [8] discovered that the ultrafine grain sizes introduced by ECAP were not stable at temperatures higher than 200 °C in pure Al and Al-Mg alloys, but fine-grained structures were retained in Al-Zr alloy up to 300 °C. VALIEV et al [9] found out that the severely plastic deformation (SPD) followed by heating made materials have new unusual properties. Aluminum alloy 6061 exhibited obvious high hardness after pre-ECAP solid-solution treatment combined with post-ECAP aging treatment [10]. The microstructures and textures of Al-0.5Cu alloy after ECAP exerted profound influences on the texture evolution during the subsequent annealing at 150-300 °C for 1 h [11].
The magnetic field was found to have great influences on the grain boundary-related phenomena such as grain boundary migration, texture formation, recrystallization, phase precipitation and transformation in ferromagnetic and non-ferromagnetic (i.e., paramagnetic and diamagnetic) materials [12-16]. HARADA et al [12] indicated that a magnetic field enhanced the grain growth at the early stage of annealing and produced a homogeneous grain boundary microstructure in nanocrystalline nickel. Sharp textures of {100} and {110} were generated when Fe-6.5%Si (mass fraction) alloy was annealed at 1100 °C without and with a DC magnetic field of 6 T, respectively [13,14]. The magnetic annealing could be a powerful tool to alleviate the grain boundary segregation and segregation- induced brittleness in iron alloys [15,16]. TSUREKAWA et al [16] demonstrated that magnetic annealing decreased tin concentration at random boundaries in Fe-0.8%Sn (mole fraction) alloy at 973 K for 6 h with a magnetic field up to 6 T. However, few researches are available for the effects of hot rolling before ECAP and magnetic field on the microstructures and properties of annealed ECAPed 1050 aluminum alloys. In the present work, the hardness, grain structures and dislocations of 1050 aluminum alloy prepared by hot rolling and subsequent equal-channel angular pressing at cryogenic temperature (cryoECAP) were investigated in detail after annealing at various temperatures under a magnetic field of 12 T.
2 Experimental
The material used was 1050 aluminum alloy. The as-cast alloy was hot-rolled to 15 mm with a reduction of 62.5% before cryoECAP. The ECAP die has a channel with dimensions of 10 mm × 10 mm. The channel intersection angle
is 90° and the curvature on the outer side of channel intersection (Ψ) is 20°. The samples were pressed through the die 8 times via route BC at a speed of 7 mm/s, and cooled to -150 °C in liquid nitrogen for more than 1 h before each pressing cycle between consecutive passes. The samples after 8 ECAP passes were then heat-treated at 150, 200, 250, 300, 350 and 400 °C for 1 h without and with magnetic field of 12 T, respectively. A stable magnetic intensity of 12 T was applied during annealing.
The Vickers hardness of sample under each condition was measured on the longitudinal section (plane Z) with a load of 30 N for 15 s, and averaged by 10 measurements. The microstructures were characterized by an LEO 1530 field emission-scanning electron microscope (FE-SEM) with an Oxford-INCA electron back scattering diffraction pattern (EBSD) system on the longitudinal section paralleling to the pressing direction. The samples for EBSD analysis were prepared by standard metallographic methods and electropolished in a solution of 20 mL HClO4 and 190 mL CH3OH at 20 °C for 20 s. The boundaries with mis-orientations less than 15° are defined as low angle boundaries (LABs) and those with mis-orientation larger than 15° are defined as high angle boundaries (HABs). The characteristics of dislocations and substructures were examined using a FEI TECNAI G220 type transmission electron microscope (TEM). The TEM foils were prepared by twin-jet thinning electrolytically in a solution of 30% nitric acid and 70% methanol (volume fraction) at -25 °C.
3 Results
The hardness evolution of as-rolled cryoECAPed (RECAPed) samples during annealing without and with magnetic field is presented in Fig. 1. The hardness of RECAPed sample annealed without magnetic field decreases slightly at temperatures lower than 200 °C, and then decreases sharply between 200-300 °C, and finally reaches a saturate value at temperatures higher than 300 °C. Similar tendency can be found for the sample annealed with magnetic field except that the sharp decrement occurs between 150-300 °C. Compared with that of sample annealed without magnetic field, the hardness of sample annealed with magnetic field is lower at 150-300 °C and higher at temperatures higher than 300 °C.
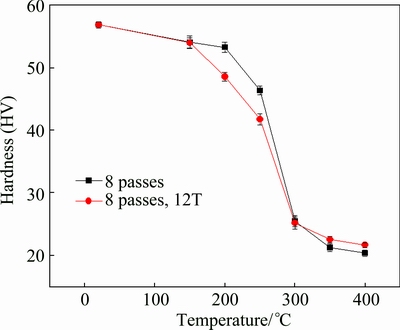
Fig. 1 Hardness of as-rolled cryoECAPed samples annealed at different temperatures without and with magnetic field
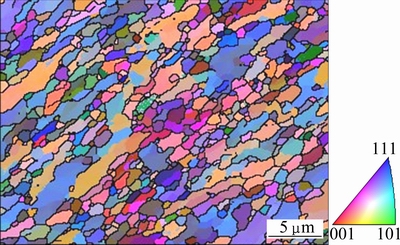
Fig. 2 Initial grain distribution of as-rolled cryoECAPed sample
The colored map of grain size distribution along with the [001] inverse pole figure of RECAPed sample is illustrated in Fig. 2. The accepted grain tolerance angle is 2°. Plenty of equiaxed subgrains and elongated subgrains with similar color are observed in deformation bands paralleling to the pressing direction, and the average grain size is about 0.6 μm. The fraction of HABs in RECAPed sample is 0.5.
Figure 3 demonstrates the grain microstructures of samples annealed at 150-250 °C for 1 h without and with magnetic field. A mixture of fine equiaxed and elongated grains is observed in the samples annealed without and with magnetic field at 150 °C, and the sample annealed with magnetic field has more fraction of equiaxed grains (Figs. 3(a) and (b)). When annealed at 250 °C, the number of equiaxed grains increases significantly (Figs. 3(c) and (d)), and larger grain sizes are found in the sample annealed with magnetic field.
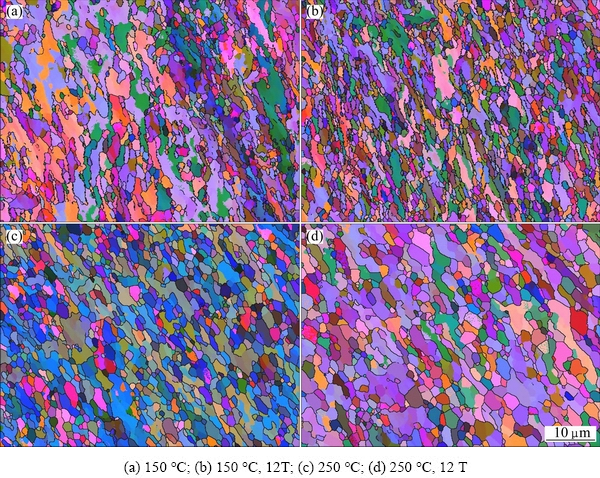
Fig. 3 Grain structures of samples annealed at 150-250 °C for 1 h
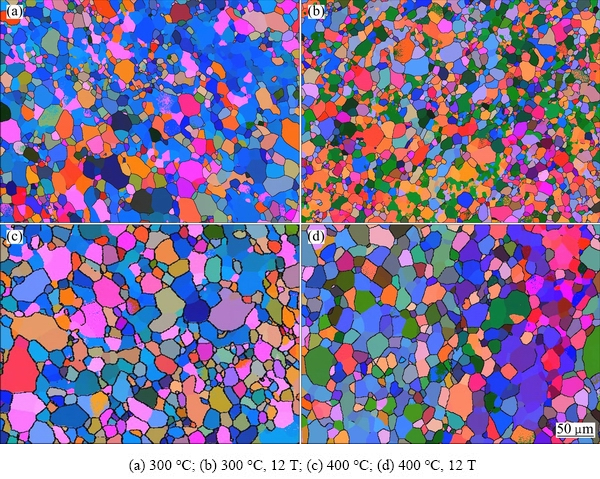
Fig. 4 Grain structures of samples annealed at 300-400 °C for 1 h
Figure 4 presents the grain microstructures of RECAPed samples annealed at 300-400 °C for 1 h without and with magnetic field. Obviously, abnormal grain growth occurs in the sample annealed at 300 °C without magnetic field (Fig. 4(a)), and typical bimodal grain size distributions with small grain size of about 12 μm and large grain size of about 50 μm are formed in the sample annealed at 400 °C (Fig. 4(c)). With the application of magnetic field, the grain size distributions are more homogeneous and no abnormal grain growth is observed (Figs. 4(b) and (d)).
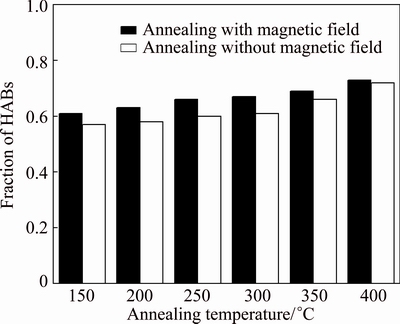
Fig. 5 Changes of fraction of HABs with annealing temperature in as-rolled cryoECAPed samples without and with magnetic field
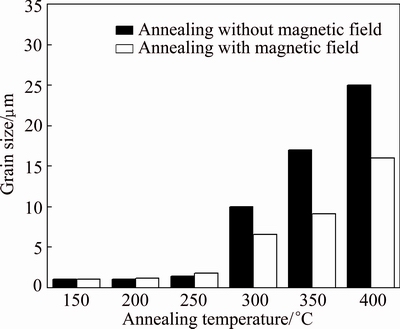
Fig. 6 Changes of grain size with temperature in as-rolled cryoECAPed samples without and with magnetic field
Figure 5 shows the evolution of fraction of HABs in RECAPed samples annealed at 150-400 °C for 1 h without and with magnetic field. The amount of HABs increases with increasing the temperature in the samples without and with magnetic field. In addition, the application of magnetic field enhances the formation of HABs, but the deviation in the fraction of HABs decreases when the temperature is higher than 300 °C.
Figure 6 illustrates the evolution of grain size of RECAPed samples annealed at 150-400 °C for 1 h without and with magnetic field. The grain growth can be divided into low temperature region at 150-250 °C and high temperature region at 300-400 °C. In low temperature region, slow grain growth is found and the average grain sizes are 1.04-1.4 μm in the samples annealed without magnetic field. Magnetic field slightly increases the grain growth at low temperature region and produces the average grain sizes of 1.06-1.8 μm in the samples annealed with magnetic field. In high temperature region, the grain sizes increase significantly and are 10-25 μm for the samples annealed without magnetic field and 6.6-16 μm for the samples annealed with magnetic field.
Figure 7 demonstrates the morphologies of grains and dislocations of RECAPed samples annealed at 150-300 °C for 1 h without and with magnetic field. Plenty of elongated subgrains are visible in shear bands, and the tangled dislocations locate at grain boundaries when the alloy is annealed at 150 °C without magnetic field (Fig. 7(a)). At 200 °C, the deformation bands disappear, the dislocation density decreases further (Fig. 7(c)). At 300 °C, the recrystallized grains grow rapidly, and the abnormal grain growth can be observed (Fig. 7(e)). With the application of magnetic field (Figs. 7(b), (d) and (f)), the dislocation density decreases.
4 Discussion
4.1 Effect of hot rolling on microstructural evolution of cryoECAPed 1050 aluminum alloy during annealing
The microstructural characterizations of RECAPed 1050 aluminum alloy annealed at different temperatures are investigated. It can be seen that when the alloy is annealed at 150-250 °C (Fig. 3), the elongated grains grow into the equiaxed grains and the grain sizes increase slowly. The TEM images of RECAPed samples (Fig. 7) display that plenty of elongated subgrains still remain, and the tangled dislocations segregate at the grain boundaries at 150 °C, and the dislocation density decreases further at 200 °C, and the recrystallized grains grow up rapidly finally at 300 °C.
According to the EBSD results, more equiaxed grains with a smaller average size (0.6 μm) and a higher fraction of HABs (0.5) are observed in the RECAPed sample (Fig. 2) than those in the as-cast ECAPed (CECAPed) sample (with an average grain size of 0.7 μm and a fraction of HABs of 0.36) reported in our previous work [17]. This is mainly because the hot- rolled samples have a larger deformation than the as-cast samples, and then the higher deformation storage energy in hot-rolled samples increases the driving force for the nucleation and growth of grain, thus resulting in an accelerated recrystallization in the RECAPed sample. In addition, higher fraction of grain boundaries in the hot-rolled sample with smaller grain size provides more nuclei, thus accelerating the recrystallization. It is known that HABs have a higher surface energy and are more likely to migrate and eliminate the dislocations in their migration route during moving, and then produce a perfect fully recrystallized structure. Higher fraction of HABs generates higher recrystallization rate in the RECAPed sample annealed at temperatures lower than 250 °C (Fig. 3), thus, more fully recrystallized grains and more homogeneous grain size distributions are detected in the RECAPed samples annealed at temperatures higher than 300 °C (Fig. 4) than those in the CECAPed samples [17].
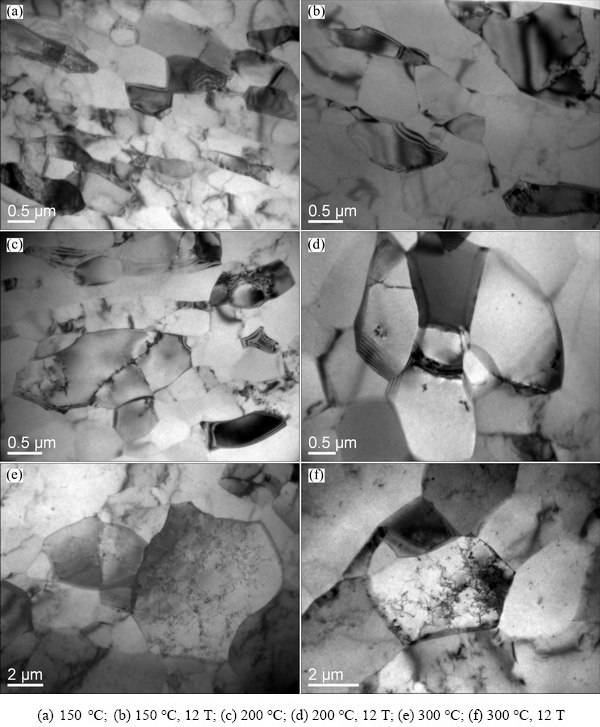
Fig. 7 TEM images of grains and substructures of as-rolled cryoECAPed samples annealed at 150 -300 °C for 1 h
4.2 Effects of magnetic field on microstructural and property evolution of cryoECAPed 1050 aluminum alloy during annealing
With the application of magnetic field, more equiaxed grains can be seen in the sample annealed at 150 °C (Fig. 3(b)), and the grain sizes of sample annealed at 250 °C are slightly larger (Fig. 3(d)), compared with those in RECAPed samples annealed without high magnetic field (Figs. 3(a) and (c)). This indicates that magnetic field can enhance the recovery and accelerate the subsequent recrystallization, and promote the grain growth at low temperatures. Moreover, a lower hardness is observed in the RECAPed samples annealed with magnetic field in low temperature region, showing that quicker recovery and recrystallization occur in the RECAPed samples with the application of magnetic field. The hardness reaches a saturate value at temperatures higher that 300 °C in RECAPed samples (Fig. 1).
The process of recovery in an ECAPed material is based on the interactions between dislocations, and the deformed structures will disappear gradually. Thermally activated motions of dislocations, such as the cross slipping of screw dislocations and climbing of edge dislocations, will lead to their rearrangement and mutual annihilation, and result in a lower dislocation density observed in the samples annealed with magnetic field at 150 °C (Fig. 6(b)). It is known that a magnetic field can influence the motion of dislocations without any external mechanical stress, which is attributed to the spin- dependent interactions between dislocations and paramagnetic defects in the crystal structure, and the interactions result in the release of dislocations from their pinning centers [18]. ALSHITS et al [19] point out that the magnetic field is not to exert an additional force on dislocations to promote their thermally activated depinning from local obstacles, but to break the local barriers due to specific spin processes in a dislocation– paramagnetic center system. The enhanced dislocation motion by magnetic field due to the above mechanism can accelerate the recovery in deformed structure, as shown in our present work.
It has been revealed that under a directed external field, the anisotropy will generate a free energy difference between adjacent grains to provide a driving force for boundary displacement [20]. This driving force does not depend on the boundary properties and moves a boundary from the grain with lower free energy towards the one with higher free energy. Since the motion of grain boundaries constitutes the fundamental process of recrystallization and grain growth, we assume that the application of magnetic field in the RECAPed samples enhances the recrystallization and grain growth due to the increased grain boundary motion.
When annealed at 300-400 °C (Fig. 4), the abnormal grain growth occurs, the grains grow rapidly and typical bimodal grain size distributions appear. It can be seen that a more homogeneous structure and lower grain sizes were produced when annealed with magnetic field at high temperatures (Figs. 4(b) and (d)). This indicates that magnetic field can suppress the abnormal grain growth and form a homogeneous grain structure. Because the high distortion energy in RECAPed samples is consumed quickly during recovery in the samples annealed with magnetic field, no enough energy is provided for the grain boundary migration, thus a more homogeneous structure with lower grain sizes is produced. HARADA et al [12] discovered that the application of magnetic field enhanced the grain growth at the early stage of annealing and finally resulted in a homogeneous grain-boundary microstructure in nanocrystalline nickel when the samples were annealed at 300 °C for 120-1800 s under a direct current magnetic field of 1.2 MA/m.
5 Conclusions
1) Annealing at 200 and 250 °C with magnetic field obviously lower the hardness of RECAPed samples, due to the accelerated recovery and recrystallization of RECAPed samples in magnetic field.
2) The hot rolling before cryoECAP produces more equiaxed grains with a smaller average size and a higher fraction of HABs, resulting in an accelerated recrystallization at temperatures lower than 250 °C and more fully recrystallized grains and more homogeneous grain size distributions at temperatures higher than 300 °C.
3) The magnetic field promotes the mobility of dislocations and grain boundaries, thus accelerating the recovery and recrystallization of RECAPed samples at 150-250 °C. In addition, the magnetic field inhibits the grain coarsening and produces a more homogeneous microstructure at 300-400 °C due to the rapid consumption of stored distortion energy during recovery.
References
[1] STOLYAROV V V, ZHU Y T, ALEXANDROV I V, LOWE T C, VALIEV R Z. Influence of ECAP routes on the microstructure and properties of pure Ti [J]. Materials Science and Engineering A, 2001, 299(1-2): 59-67.
[2] LIU M P, JIANG T H, WANG J, LIU Q, WU Z J, YU Y D, SKARET P S, ROVEN H J. Aging behavior and mechanical properties of 6013 aluminum alloy processed by severe plastic deformation [J]. Transactions of Nonferrous Metals Society of China, 2014, 24(12): 3858-3865.
[3] SHAERI M H, SHAERI M, SALEHI M T, SEYYEDEIN S H, DJAVANROODI F. Microstructure and texture evolution of Al-7075 alloy processed by equal channel angular pressing [J]. Transactions of Nonferrous Metals Society of China, 2015, 25(5): 1367-1375.
[4] SHOKUHFAR A, NEJADSEYFI O. The influence of friction on the processing of ultrafine-grained/nanostructured materials by equal-channel angular pressing [J]. Journal of Materials Engineering and Performance, 2014, 23(3): 1038-1048.
[5] OHISHI K, HORITA Z, FURUKAWA M, NEMOTO M, LANGDON T G. Optimizing the rotation conditions for grain refinement in equal-channel angular pressing [J]. Metallurgical and Materials Transactions A, 1998, 29(9): 2011-2013.
[6] Furukawa M, Iwahashi Y, Horita Z N, Langdon T G. Effect of processing routes on microstructure and mechanical properties of 2014 Al alloy processed by equal channel angular pressing [J]. Transactions of Nonferrous Metals Society of China, 2010, 20(10): 1822-1828.
[7] QIAN Chen-hao, LI Ping, XUE Ke-min. Influence of deformation passes on interface of SiCp/Al composites consolidated by equal channel angular pressing and torsion [J]. Transactions of Nonferrous Metals Society of China, 2015, 25(5): 1376-1382.
[8] HASEGAWA H, KOMURA S, UTSUNOMIYA A, HORITA Z, FURUKAWA M, NEMOTO M, LANGDON T G. Thermal stability of ultrafine-grained aluminum in the presence of Mg and Zr additions [J]. Materials Science and Engineering A, 1999, 265(1-2): 188-196.
[9] VALIEV R Z, ISLAMGALIEV R K, ALEXANDROV I V. Bulk nanostructured materials from severe plastic deformation [J]. Progress in Materials Science, 2000, 45(2): 103-189.
[10] KIM J K, JEONG H G, HONG S I, KIM Y S, KIM W J. Effect of aging treatment on heavily deformed microstructure of a 6061 aluminum alloy after equal channel angular pressing [J]. Scripta Materialia, 2001, 45(8): 901-907.
[11] FERRASSE S, SEGAL V M, ALFORD F. Texture evolution during equal channel angular extrusion (ECAE) part II. An effect of post-deformation annealing [J]. Materials Science and Engineering A, 2004, 372(1-2): 235-244.
[12] HARADA K, TSUREKAWA S, WATANABE T, PALUMBO G. Enhancement of homogeneity of grain boundary microstructure by magnetic annealing of electrodeposited nanocrystalline nickel [J]. Scripta Materialia, 2003, 49(5): 367-372.
[13] WATANABE T, FUJII H, OIKAWA H, ARAI K I. Grain boundaries in rapidly solidified and annealed Fe-6.5 mass% Si polycrystalline ribbons with high ductility [J]. Acta Metallurgica, 1989, 37(3): 941-952.
[14] WATANABE T, TSUREKAWA S, FUJII H, KANNO T. The control of texture and grain boundary microstructure by magnetic annealing [J]. Materials Science Forum, 2005, 495-497: 1151-1158.
[15] TSUREKAWA S, KAWAHARA K, OKAMOTO K, WATANABE T, FAULKNER R. Application of magnetic field to the control of grain boundary segregation in iron [J]. Materials Science and Engineering A, 2004, 387-389: 442-446.
[16] TSUREKAWA S, OKAMOTO K, KAWAHARA K, WATANABE T. The control of grain boundary segregation and segregation-induced brittleness in iron by the application of a magnetic field [J]. Journal of Materials Science, 2005, 40(4): 895-901.
[17] HE L Z, CAO Y H, LIU X T, ZHANG H T, WANG P, LU C, GUO Y P, CUI J Z. Influences of magnetic annealing on the grain growth in a cryoECAPed 1050 aluminum alloy [J]. Materials Characterization, 2013, 84(10): 188-195.
[18] MORGUNOV P B. Spin micromechanics in the physics of plasticity [J]. Physics Uspekhi, 2004, 47(2): 125-147.
[19] ALSHITS V I, DARINSKAYA E V, KOLDAEVA M V, PETRZHIK E A. Magnetoplastic effect: Basic properties and physical mechanisms [J]. Crystallography Reports, 2003, 48(5): 768-795.
[20] MOLODOV D A, GOTTSTEIN G, HERINGHAUS F, SHVINDLERMAN L S. Motion of planar grain boundaries in bismuth-bicrystals driven by a magnetic field [J]. Scripta Materialia, 1997, 37(8): 1207-1213.
强磁场和热轧对低温等径角挤压1050铝合金组织和性能的影响
曹以恒1,何立子1,张 林2,周亦胄3,王 平1,崔建忠1
1. 东北大学 材料电磁过程研究教育部重点实验室,沈阳 110819;
2. 东北大学 材料科学与工程学院,沈阳 110819;
3. 中国科学院 金属研究所,沈阳 110016
摘 要:研究在不施加和施加12 T强磁场条件下,于150~400 °C下退火1 h后,经热轧和低温等径角挤压 (cryoECAP)制备的1050铝合金的硬度和显微组织的演化规律。通过背散射电子衍射(EBSD)和透射电子显微镜(TEM)表征晶粒的显微结构和位错。研究表明:在cryoECAP变形前进行热轧可使cryoECAP制备的1050铝合金产生更多更小尺寸的等轴晶以及更多的大角度晶界(HABs),这些都能在随后的退火过程中促进合金的回复和再结晶且使合金组织变得更加均匀。在150~250 °C施加强磁场退火能促进回复和再结晶过程,并且使硬度值明显降低。在300~400 °C退火过程中施加磁场能够抑制晶粒的异常长大,促使晶粒尺寸分布更加均匀。
关键词:1050铝合金;磁场退火;热轧;低温等径角挤压;硬度;晶粒长大
(Edited by Mu-lan QIN)
Foundation item: Project (2011CB606403) supported by the State Basic Research Development Program of China; Projects (51171044, 51174058) supported by the National Natural Science Foundation of China; Project (2012CB723307) supported by the State Basic Research Development Program of China
Corresponding author: Li-zi HE; Tel: +86-24-83681760; E-mail: helizi@epm.neu.edu.cn
DOI: 10.1016/S1003-6326(16)64150-X