
Effect of 5%Cr on oxidation of Ni-10Al in
0.1MPa O2 at 900 ℃ and 1 000 ℃
ZHANG Xue-jun(张学军)1, 2, GAO Chun-xiang(高春香)1, NIU Yan(牛 焱)2
1. College of Applied Chemistry, Shenyang Institute of Chemical Technology, Shenyang 110142, China;
2. State Key Laboratory for Corrosion and Protection, Institute of Metal Research, Chinese Academy of Sciences, Shenyang 110016, China
Received 28 July 2006; accepted 15 September 2006
Abstract: The effect of 5%Cr(mole fraction) on the scaling behavior of Ni-10Al was investigated at 900 ℃ and 1 000 ℃ with the aim to discuss the third element effect(TEE). The oxidation rate of the ternary alloy containing Cr is much slower than the corresponding binary alloys at both temperatures. A dense external scale of NiO overlying a zone of internal oxide precipitates forms on Ni-10Al, while Ni-5Cr-10Al develops a protective layer. So the addition of Cr promotes the formation of alumina scale. The possible mechanism of Cr on the oxidation of Ni-Al alloy is discussed.
Key words: Ni-Cr-Al alloy; Ni-10Al alloy; oxidation; third-element effect
1 Introduction
Binary and ternary Ni-based alloys are often used as model systems to study the processes occurring in the oxidation of complex multi-component Ni-based superalloys. The minimum aluminum content needed to develop protective Al2O3 scales on Ni-based binary alloys was estimated to range between 8% and 17%(mass fraction) (15.9% to 30.8% in mole fraction, correspondingly)[1-4]. Unfortunately, these aluminum levels in binary alloys often result in unacceptable mechanical properties. The addition of chromium to Ni-Al alloys can reduce the critical Al content to form Al2O3 scales, providing a case of the so-called third-element effect (TEE), which is of considerable interest for optimizing the composition of commercial alloys. The classical interpretation, initially proposed by WAGNER[5], assumes that the third element can reduce the flux of oxygen into the alloy, favoring the formation of external scales of the most reactive component. However, the precise role of the third element is not yet very clear. One of other possible mechanisms advanced later[6], originally proposed by GIGGINS and PETTIT[7], is based on the idea that the simultaneous internal precipitation of the oxides of the two most reactive components produces an overall volume fraction of internal oxide sufficient for the transition to an external scale according to a criterion defined for binary alloys by WAGNER[8] under reduced contents of the most reactive component than in binary alloys. Thus, the scaling behavior of Ni-5Cr-10Al was studied with the purpose to interpret the Cr effect in the oxidation of Ni-Al alloy.
2 Experimental
The alloy ingots were prepared from high-purity metals by arc-melting under a Ti-gettered argon atmosphere using non-consumable tungsten electrodes from appropriate amounts of pure nickel (99.99%), aluminum (99.999%) and Cr (99.99%). The alloy ingots were subsequently annealed in argon atmosphere at
1 200 ℃ for 24 h to remove the residual mechanical stresses to achieve a better equilibration of the alloy phases. The nominal compositions (mole fraction, %) of the alloys studied here are Ni-10Al and Ni-5Cr-10Al, and the corresponding actual average compositions are Ni-10.27Al and Ni-5.22Cr-10.41Al, respectively.
Flat specimens with a surface area of about 2 cm2 were cut from the ingots by a diamond wheel saw, ground down to 1200 emery paper, washed in water, alcohol and acetone and then dried immediately before use. Oxidation tests were carried out in 0.1 MPa of pure oxygen at 1 373 K for 24 h with continuous mass gain measurement using a microbalance. The microstructures of the alloys and the oxide scales were characterized with scanning electron microscope(SEM) and an energy- dispersive X-ray spectrometer(EDAX) attached to the SEM to establish the nature, composition, and spatial distribution of the compounds formed by oxidation.
3 Results and discussion
3.1 Scaling kinetics
The kinetics curves of the two alloys oxidized at 900 and 1 000 ℃ are shown in Fig.1(a) and Fig.2(a) as normal plots in Fig.1(b) and Fig.2(b) as parabolic plots. In general, the oxidation rate of the ternary alloy containing Cr is much slower than the corresponding binary alloy at both temperatures. At 900 ℃, the oxidation kinetics of the present alloys Ni-10Al and Ni-5Cr-10Al obeyed the parabolic rate law for the whole duration of the test with rate constants of 2.0×10-11 and 1.8×10-12 g2?cm-4?s-1, respectively. At 1 000℃, the kinetics of Ni-10Al obeyed the parabolic rate law for the whole test duration with a rate constant of 1.5×10-10 g2?cm-4?s-1, while that of Ni-5Cr-10Al decreased from 2.1×10-11 g2?cm-4?s-1 for the first 4 h to 1.1×10-11 g2?cm-4?s-1 from 4 to 24 h.
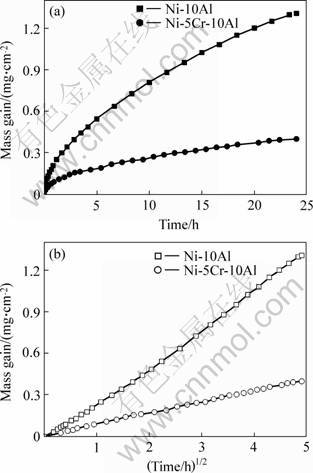
Fig.1 Scaling kinetics of Ni-10Al and Ni-5Cr-10Al alloys oxidized at 900 ℃ in 0.1 MPa O2: (a) Normal plots; (b) Parabolic plots
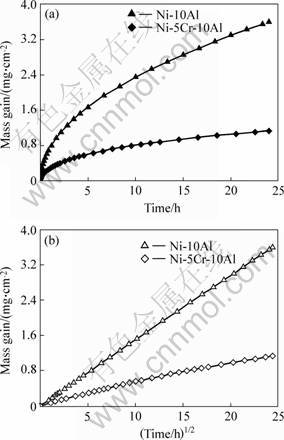
Fig.2 Scaling kinetics of Ni-10Al and Ni-5Cr-10Al alloys oxidized at 1 000℃ in 0.1 MPa O2: (a) Normal plots; (b) Parabolic plots
3.2 Scale microstructure and composition
At 900 ℃ (Fig.3(a)), Ni-10Al formed a dense external scale of NiO about 8 mm thick containing some Ni-Al spinel particles in the inner region corresponding approximately to the alloy consumption zone, followed by a zone of internal oxidation about 20 mm deep, where the size of the internal oxide particles increased with depth. After 24 h oxidation at 900 ℃ the surface scale grown on the surface of Ni-5Cr-10Al was strongly non-uniform (Fig.3(b)). A fraction of the alloy surface formed an external NiO followed by an irregular zone of internal oxidation of aluminum and chromium, but still thinner than those formed on Ni-10Al, while the remaining fraction developed an Al2O3 layer surmounted by a very thin and discontinuous NiO layer. Oxidation of Ni-10Al at 1 000 ℃ (Fig.3(c)) formed a thicker layer of NiO (about 20 mm) and a deeper zone of internal oxidation (about 45 mm), where the Al2O3 particles had a smaller size and formed bands parallel to the alloy surface. After 24 h oxidation at 1 000 ℃ the same alloy presented a very thin layer of Al2O3 surmounted by a few particles of NiO (Fig.3(d)).
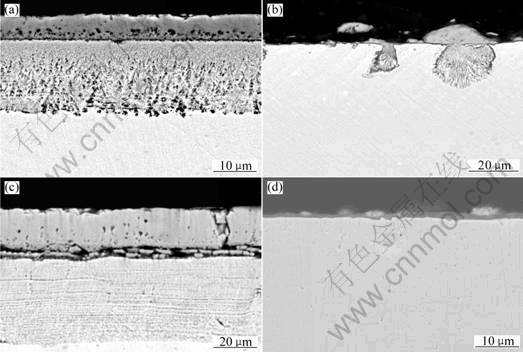
Fig.3 Cross sectional images(SEM/BEI) of Ni-10Al at 900 ℃ (a) and 1 000 ℃ (c), and Ni-5Cr-10Al at 900 ℃ (b) and 1 000 ℃ (d) after 24 oxidation in 0.1 MPa O2
3.3 Discussion
It is only briefly recalled that the minimum concentration of aluminum needed to avoid the internal oxidation of Al on Ni-Al alloys is between 8% and 11.7% Al(mass fraction), corresponding to 15.9% and 22.4% Al(mole fraction), at 900 ℃ and between 5.4% and 8.8% Al(mass fraction), corresponding to 11.1% and 17.4% Al(mole fraction), at 1 000 ℃. Moreover, the critical Al content needed to form stable external alumina scales on the same alloys ranges from 14.1% to 17.0% Al(mass fraction), corresponding to 26.3% and 30.8% Al(mole fraction), at both 900 and 1 000 ℃[2]. A systematic study of the oxidation of ternary Ni-Cr-Al alloys at 1 000-1 200 ℃ carried out by GIGGINS and PETTIT[7] identified three groups of alloys. Alloys of group I formed external NiO scales associated with an internal oxidation of chromium plus aluminium. Alloys of group II formed external chromia scales coupled to an internal oxidation of aluminium. Finally, alloys of group III formed exclusive external alumina scales. In addition, it has been found that the addition of chromium reduces the critical aluminum content needed to form alumina scales with respect to binary Ni-Al alloys[7].
The exclusive growth of external Al2O3 scales in the oxidation of ternary Ni-Cr-Al alloys requires to avoid two different kinds of internal oxidation: 1) the coupled internal oxidation of Al and Cr beneath external NiO scales and 2) the internal oxidation of Al beneath external chromia scales. The critical Al contents needed for the transitions from these forms of internal oxidation to the external oxidation of Al were calculated in our previous publications[10, 11]. It is concluded that the critical Al content needed to avoid the coupled internal oxidation of Cr+Al beneath external NiO scales, xAlo*(t,NiO), decreases almost linearly with an increase of the Cr content of the alloy. In particular, at 900 ℃ xAlo*(t,NiO) decreases from the value typical of binary Ni-Al alloys, xAlo*(Ni-Al), equal to 11.6%(mole fraction), in the absence of Cr, down to zero for the binary Ni-Cr alloys, for which the critical Cr content needed to avoid the internal oxidation of Cr, xCro*(Ni-Cr), is equal to 8.3%(mole fraction)[10,11]. A second critical Al content needed for the transition between the internal oxidation beneath Cr2O3 scale and the formation of external alumina scales, xAlo*(t,Cr2O3), which is again a function of the chromium content in the bulk alloy, xCro. At 900 ℃ this analysis yielded a value of xAlo*(t,Cr2O3) independent of the Cr content in the alloy in the range of compositions and equal to 1.7%(mole fraction). According to the theoretical oxidation map[10,11], the Ni-5Cr-10Al falls into the zone of forming alumina protective scale. The present experimental results agree with the theoretical analysis[10,11] and with the oxidation map obtained by experiment[7].
4 Conclusions
1)At 900 ℃ nd 1 000 ℃, the oxidation rate of Ni- 5Cr-10Al alloy was much slower than that of the Ni-10Al.
2)Ni-10Al alloy formed external NiO scales followed by a zone of internal oxidation of Al. Conversely, the scale formed on Ni-5Cr-10Al alloy at 1 000 ℃ was protective.
3)A Cr addition of 5%(mole fraction) to Ni-10Al was sufficient to prevent the internal oxidation of Al and to induce the formation of external alumina scales, providing an example of third-element effect.
References
[1] WOOD G C, STOTT F H. Establishment and breakdown of α-Al2O3 scale on Ni-Al alloys at high temperatures[J]. Br Corros J, 1971, 6: 247-256.
[2] PETTIT F S. Oxidation mechanism for nickel-aluminium alloys at temperatures between 900 ℃ and 1 300 ℃[J]. Trans Metall Soc AIME, 1967, 239: 1296-1305.
[3] SMIALEK J L. Oxide morphology and spalling model for Ni-Al[J]. Met Trans, 1978, 9A: 309-320.
[4] HINDAM H M, SMELTZER W W. Growth and microstructure of coal α-Al2O3 on Ni-Al alloys: internal precipitation and transition to external scale[J] J Electrochem Soc, 1980, 127: 1622-1630.
[5] WAGNER C. Passivity and inhibition during the oxidation of metals at elevated temperatures[J]. Corros Sci, 1965, 5: 751-764.
[6] STOTT F H, WOOD G C, STRINGER J. The influence of alloying elements on the development and maintenance protective scales[J]. Oxid Met, 1995, 44: 113-145
[7] GIGGINS C S, PETTIT F S. Oxidation of Ni-Cr-Al alloy between
1 000 ℃-1 200 ℃[J]. J Electrochem Soc, 1971,118: 1782-1790.
[8] WAGNER C. Types of reaction in the oxidation of alloys[J]. Z Elektrochem, 1959, 63: 772-782.
[9] NIU Y, GESMUNDO F. The internal oxidation of ternary alloys VIII: the transition from the internal to the external oxidation under high oxidant pressure( and the third-element effect)[J]. Oxid Met, 2007, in press.
[10] ZHANG Xue-jun, GESMUNDO F, NIU Y. The third-element effect on the oxidation of Ni-xCr-7Al alloys (x = 0, 5 10 at.%) at 1173K[J]. High Temp Mater & Proc, 2005, 24(6): 369-374.
[11] NIU Yan, ZHANG Xue-jun, WU Ying, GESMUNDO F. The third-element effect in the oxidation of Ni-xCr-7Al (x = 0, 5, 10, 15 at.%) alloys in 1 atm O2 at 900-1 000℃[J]. Corros Sci, 2007, in press.
(Edited by PENG Chao-qun)
Foundation item: Project(200603206) supported by the Shenyang Institute of Chemical Technology for PhD
Corresponding author: ZHANG Xue-jun; Tel: +86-24-89383297; E-mail: xjzhang_syict@163.com