
Electrochemical synthesis and characterization of tantalum alkoxides
YANG Sheng-hai(杨声海)1, CAI Ya-nan(蔡亚楠)2, YANG Hai-ping(杨海平)1, JIN Sheng-ming(金胜明)2
1. School of Metallurgical Science and Engineering, Central South University, Changsha 410083, China;
2. School of Minerals Processing and Bioengineering, Central South University, Changsha 410083, China
Received 10 August 2009; accepted 15 September 2009
Abstract: Tantalum(Ⅴ) propoxide (Ta(OPrn)5), isopropoxide (Ta(OPri)5) and butoxide(Ta(OBun)5) were synthesized by electro- chemical reactions of corresponding alcohol at sacrificial tantalum anode in the presence of tetraethylammonium chloride as a conductive additive. The pure products were isolated by reduced pressure distillation under 5 kPa. The crystal of Ta(OPri)5 was obtained by recrystallization from hexane at -10 ℃. These samples were characterized by Fourier transform infrared spectra (FT-IR), Raman spectra, nuclear magnetic resonance spectra (NMR), TG/DTA and ICP-MS. The results show that direct electrochemical synthesis of metal alkoxides has a high current efficiency and electrolysis yield. These alkoxides have a high purity of 99.97% and can be directly used as the precursor of Ta2O5 films.
Key words: electrochemical synthesis; tantalum alkoxides; spectral characterization; thermal analysis
1 Introduction
Tantalum oxide, Ta2O5, has important applications as a dielectric material in dynamic random access memory (DRAM), ultra-large scale integrated technology (ULSI)[1], and electroluminescent devices; and other applications include being used as corrosion- resistant materials, integrated optical device and thin film capacitors, high-temperature ceramics, antireflection coatings for solar cells, optical waveguides, and solid- state O2 sensors[2]. Traditional film fabrication techniques include thermal oxidation, reactive sputtering, ion plating, sol-gel processing[3-4], and chemical vapor deposition (CVD)[5]. Tantalum alkoxides are mainly used for the production of Ta2O5.
Most tantalum alkoxides such as tantalum(V) ethoxide have binuclear structures, [Ta2(OR)8(μ-OR)2], with two bridging alkoxide ligands[6]. In order for the tantalum(V) centers to attain octahedral coordination, evaporation occurs only after dissociation to monomers [Ta(OR)5]. It was considered that if the carbochain gets long or branched chain exists, the steric hindrance effect might improve the volatility of the alkoxides. So, a useful new class of precursors for CVD of tantalum oxides is necessary. On the other hand, longer carbochain or branched chain will make the precursor not so sensitive to moisture as tantalum ethoxide[7].
Tantalum pentachloride is usually employed as the raw material for synthesis of tantalum alkoxide which is the product of the reaction between tantalum pentachloride and alcohol in diluted solvent of benzene or toluene[8]. As metal halide reacts with alcohol only at partial replacement, it is necessary to add some reagents like ammonia or pyridine to impel the reaction to complete. In order to remove the trace halide solved in alcohol and tantalum alkoxides, alkali metals are added for the formation of metal halides[9]. This preparation method has some disadvantages, such as restricted requirements on equipments, bad work condition, low recovery rate and toxic diluted solvent pollution. Significant progress was made in the electrochemical synthesis of metalorganic complexes in last several decades. Many similar complexes of reactive metals, such as iron, cobalt, nickel and copper, have been produced by this technique[10]. In contrast to conventional methods, the electrochemical synthetic process is more productive and inexpensive with regards to apparatus and raw materials[11-12]. Furthermore, it is more friendly to the environment.
In the present work, Ta(OPrn)5, Ta(OPri)5 and Ta(OBun)5 were directly prepared with tantalum plate (industrial grade) as a sacrificial anode in absolute alcohol. The condensed products were isolated by reduced pressure distillation under 3-5 kPa. Samples were characterized by FT-IR, Raman spectra, nuclear magnetic resonance (NMR), thermal analysis and ICP-MS.
2 Experimental
2.1 Materials and equipments
Anhydrous propanol and isopropanol, butanol(AR grade) were purchased from Tianjin Hengxing Chemical Preparation Co. Ltd., China. Tetraethylammonium chloride (TEAC) (AR grade) was purchased from Jintan Huadong Chemical Research Institute of Jiangsu Province, China. All reagents were used without further purification.
A stainless steel plate was used as cathode, and its working area was 19.0 cm×12.0 cm. A tantalum plate (3.0 kg), purchased from Zhuzhou Cemented Carbide Group Co. Ltd., China, was employed as the anode, and its working area was 18.0 cm×10.0 cm. The tantalum plates were made from tantalum powders (metallurgical grade) by self-resistance sintering, electron bombardment and rolling treatment. The chemical compositions of the tantalum plate are listed in Table 1. A DC electrical source (WYJ 15A-60V) was supplied by Shanghai Wenkai Power Equipment Co. Ltd., China.
Table 1 Chemical compositions of tantalum anode (mass fraction, %)
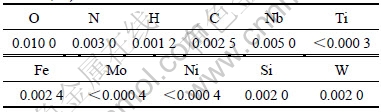
2.2 Synthesis and isolation
Electrochemical synthesis was performed in a plastic cell with undivided cathodic and anodic spaces, equipped with a condenser. Solutions of TEAC (0.04 mol/L) in the corresponding alcohol were used as electrolytes. The two electrodes were polished with a series of emery papers, degreased in acetone, rinsed in deionized water and corresponding alcohol. The process was performed under direct current with refluxing of the electrolyte and hydrogen evolution at the cathode. Voltage of 60 V, and process duration of 24-48 h were applied depending on the yield wanted.
Firstly, distillation was performed under ambient pressure to separate redundant alcohol from mixed solutions of alcohols and tantalum alkoxides at temperature above the boiling point of corresponding alcohol. Then, the distillation temperature was raised to 150 ℃ gradually, and a little amount of ester was removed. Finally, the crude tantalum alkoxide solutions were distilled at pressure of 5 kPa and oil bath temperature of 210-230 ℃. The crystal of Ta(OPri)5 was obtained by recrystallization from hexane at -10 ℃. The condensate was preserved in a dry nitrogen-sealed glass bottle to keep it from moisture. We found that the products Ta(OPrn)5, Ta(OPri)5 and Ta(OBun)5 are less air/moisture sensitive. For example, Ta(OBun)5 would be stable even if exposed to air for several hours. This characteristic makes them handy to use in solution-based CVD.
2.3 Characterization
FT-IR spectra were measured with a Nicolet Avatar 360 IR spectrometer operating in the region of 4000–400 cm-1. Raman spectra were recorded using a Laboram-010 Laser Raman spectrometer with 632.8 nm He-Ne laser. The sampling time was 1 s and samples were sealed in a glass tube in inert gas ambient. The 1H-NMR spectrum was recorded by an Inova-400(Varian) nuclear magnetic resonance spectroscope, and chloroform-d was adopted as dilute reagent. Thermogravimetric measurements were carried out in nitrogen atmosphere using a SDT Q600 V8.0 Build 95 thermoanalytical equipment (flux rate 100 cm3/min, heating rate 10 K/min, temperature interval 25-800 ℃, sample mass 10-40 mg, surface area of TG crucible 0.227 cm2). The contents of impurity metals of the hydrolyzate Ta2O5 were determined by ICP-MS Agilent 7500A.
3 Results and discussion
3.1 Structure characterization
Fig.1 shows the FT-IR spectra of three kinds of tantalum alkoxides. The several peaks between 2 970 cm-1 and 2 850 cm-1 are due to δ(C—H) stretching vibration of alkoxy groups. Peaks at around 2 962 cm-1 and 2 872 cm-1 are ascribed to asymmetric and symmetric stretching vibration, respectively.
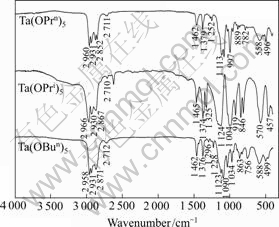
Fig.1 FT-IR spectra of Ta(OPrn)5, Ta(OPri)5 and Ta(OBun)5 samples
The sharp bands around 1 460 and 1 375 cm-1 correspond to the δ(C—H) bending vibrations. Peaks at 1 462 and 1 465 cm-1 are attributed to scissoring vibration of methylene. Peaks at around 1 375 cm-1 are attributed to symmetric bending vibration of methyl groups. Bands at 1 200-1 000 cm-1 correspond to the C—O vibrations of alkoxy groups bound to Ta. In the spectrum of Ta(OPri)5, the peak at 919 cm-1 is assigned to in-plane rocking vibration of methyl. The broad envelopes of bands below 600 cm-1 are due to the Ta—O stretching modes occurring along with bending and torsional modes of the ligands.
There are no bands between 3 600 and 3 100 cm-1 in Fig.1, showing that the samples were well preserved and the partial hydrolyzation has not occurred. It is also observed a medium peak existing at 2 621 cm-1 in the spectrum of Ta(OPri)5, which is consistent with result in Ref.[11].
The Raman spectra of the three tantalum alkoxides are shown in Fig.2. The peaks below and at 600 cm-1 could be assigned to Ta—O vibrations, and the bands around 1 080 cm-1 are attributed to Ta(—O—C) stretching vibrations[13]. Among the three spectra, the peaks at 1 050 cm-1 are attributed to C—C vibrations, and the peaks between 2 800 and 2 950 cm-1 are ascribed to C—H vibrations of the alkyl groups. They are in good agreement with the results of IR spectra.
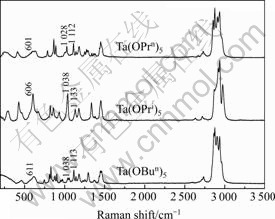
Fig.2 Raman spectra of Ta(OPrn)5, Ta(OPri)5 and Ta(OBun)5 samples
Nuclear magnetic resonance studies on tantalum alkoxides have been very useful in understanding and assigning the structure of these species. Fig.3 shows the observed 1H NMR spectra of Ta(OPrn)5, Ta(OPri)5 and Ta(OBun)5 in CDCl3 with respect to tetramethylsilane. Solvent peaks are observed at (7.26-7.28)×10-6. Peak assignments in Fig.3(a) are as follows: the signals at 4.35×10-6 and 4.18×10-6 are assigned to HA. The major peak at 1.73×10-6 is due to HB and peak at 0.90×10-6 is assigned to HC, namely H atom of the terminal methyl. The proportion of spectral integral area is 1.00?1.06?1.46, conforming to the stoichiometry of the compound Ta(OPrn)5.
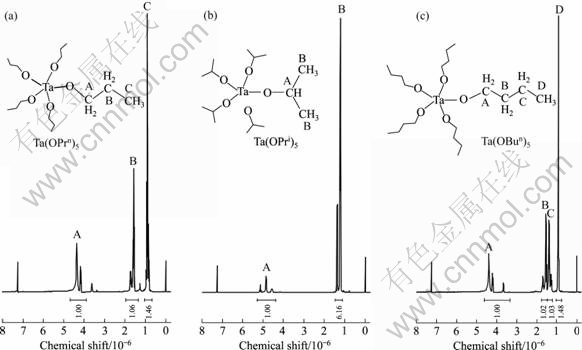
Fig.3 1H NMR spectra of Ta(OPrn)5, Ta(OPri)5 and Ta(OBun)5 samples (A, B and C representing serial number of H atoms)
As shown in the spectrum of Ta(OPri)5, the peaks around 4.87×10-6 are due to HA. Compared with Ta(OPrn)5, the peak of H atom in this position moves to high field because of shielding effect of methyl branched chain. Tantalum isopropoxide gave a complex spectrum (α-protons) containing at least 19 peaks clearly due to three overlapping septets. There is a monomer-dimer equilibrium for Ta(OPri)5 due to the steric hindrance of chain-branching of the alkyl group. The signals between 1.21 and 1.38 are assigned to two —CH3 groups. The proportion of spectral integral area is 6.16, indicating that the proportion of the two kinds of atoms is 6, too.
In the spectrum of tantalum butoxide, it is clear to comprehend the assignment of the hydrogen atoms at a glance. As shown in the plot, the chemical shift of HA is about 4.39, but chemical shift of HB and HC are very contiguous since electron-attracting effect of the oxygen atom is weak and it is difficult to have large impact on the distribution of electron cloud. The chemical shift of terminal —CH3 is equal to that of tantalum propoxide because they are both normal alkoxides. The normal alkoxides of tantalum were dimeric, so the double-bridged edge-shared bioctahedral structure was proposed[14].
3.2 Thermal analysis
Thermal property is of prime consideration in assessing the feasibility of a metallorganic compound as a precursor in chemical vapor deposition, demonstrating the conditions required to transport material from its source container to the deposition zone[15]. TG gives an indication of the thermal stability by monitoring the rate of mass loss for a sample as temperature is increased.
The thermogravimetric/differential thermogravimetric (TG/DTG) plots for Ta(OPrn)5, Ta(OPri)5 and Ta(OBun)5 samples are shown in Fig.4. The TG curve of Ta(OPrn)5 displays a single mass loss in the temperature range of 200-295 ℃. On the DTG plot, the rate of mass loss shows a rapid increase after reaching 230 ℃. The mass loss rate continues to increase after reaching the maximum rate at the DTG peak temperature of 277 ℃. According to a total mass loss of 95%, it is concluded that tantalum propoxide has almost completely evaporated. Mass loss of Ta(OPri)5 in two distinct tempetature stages can be seen from TG plot. DTG curves of Ta(OPri)5 are very complicated that the rate of mass loss has three peaks which may result from crystal transfer and structural damage. The TG/DTG curves of Ta(OBun)5 are similar to those of Ta(OPrn)5 but the remanent mass of Ta(OBun)5 is higher and the rate of mass loss is lower than that of Ta(OPrn)5.
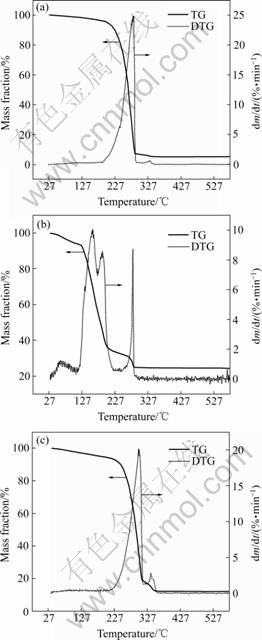
Fig.4 TG/DTG plots for Ta(OPrn)5(a), Ta(OPri)5(b) and Ta(OBun)5(c) samples
3.3 Chemical composition
Tantalum alkoxides are useful as starting materials for oxides for dielectrics. When such an oxide is used as an electronic material for dielectrics, it is required to reduce the quantity of impurities such as transition metals typified by iron, alkali metals (e.g. sodium), alkaline earth metals (e.g. calcium), as well as uranium and thorium as far as possible. These impurity elements cause various disorders such as the reduction in the durability of electronic devices, the increase in the leakage current and software errors[16]. The impurity elements were classified as Nb, Al, main group metals(such as As, Pb and Sn), mobile metals(such as Ba, Ca, Li, Mg, K, Na and Sr) and transition metals(such as Cr, Co, Cu, Fe, Mn, Mo, Ni, Ti, V and Zn). The impurities of tantalum oxides were converted into tantalum alkoxides. The concentrations of impurity elements in the samples are shown in Table 2. According to Table 2, the contents of total impurity elements are within the limit of 0.0015%-0.0030%. The content of Nb in Ta(OBun)5 is 0.002%, a little higher than that of other two compounds. Niobium alkoxides have similar vapor pressure with tantalum alkoxides at distillation temperature so that it is difficult to separate them. Thereby, it is necessary to rectify tantalum alkoxides if it is necessary to remove these impurities. Therefore, the purity of tantalum alkoxides prepared by this method can come up to 99.997% and can meet the demand of dielectric materials in electronic devices.
Table 2 Impurity contents in tantalum alkoxides (mass fraction, 10-6)
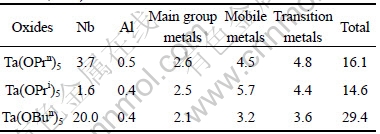
4 Conclusions
1) Ta(OR)5 of the aliphatic series can be prepared by electrochemical synthesis. Electrochemistry method appears to be rather simple and productive in comparison with the chemical methods. Large-scale preparation of these compounds is possible. These alkoxides are relatively stable and do not fume with moist air.
2) The products were characterized by IR, Raman and NMR spectra, demonstrating that the samples are corresponding tantalum alkoxides. Thermal analysis confirms that Ta(OPrn)5 and Ta(OBun)5 are suited for chemical vapor deposition of tantalum oxides films. From the results of ICP-MS, the impurities contents of these tantalum alkoxides are low enough and they are applicable to be deposited as dielectric materials in electronic devices.
References
[1] CHANELIERE C, AUTRAN J L, DEVINE R A B, BALLAND B. Tantalum pentoxide (Ta2O5) thin films for advanced dielectric applications [J]. Mater Sci Eng, 2009, 22(6): 269-322.
[2] DONGJOON M, SUNGHO P, BUM-SEOK S, CHOI S, LEE N, LEE J H. Low temperature crystallization of high permittivity Ta oxide using an Nb oxide thin film for metal/insulator/metal capacitors in dynamic random access memory applications [J]. Journal of Vacuum Science and Technology B, 2005, 23(1): 80-83.
[3] OZER N, LAMPERT C M. Structural and optical properties of sol-gel deposited proton conducting Ta2O5 films [J]. Journal of Sol-Gel Technology, 1997, 8(1/3): 703-709.
[4] YANG Sheng-hai, WANG Yi-nan, HE Jing, TANG Mo-tang, QIU Guan-zhou. Preparation of high-purity tantalum oxide nanoparticles [J]. Rare Metal Materials and Engineering, 2007, 36(2): 282-286. (in Chinese)
[5] MIKKO R, KAUPO K, ANTTI R, RAISANEN P I, LESKELA M, SAJAVAARA T, KEINONEN J. Atomic layer deposition of oxide thin films with metal alkoxides as oxygen sources [J]. Science, 2000, 288(5464): 319-321.
[6] ABRAHAMS I, BRADLEY D C, CHUDZYNSKA H, MOTEBALLI M, O’SHAUGHNESSY P. Polynuclear tantalum oxoalkoxides. Crystal structures of [Ta8O10(OEt)20], [Ta7O9(OPri)17] and [Ta5O7(OBut)11]·C6H5Me [J]. J Chem Soc, Dalton Trans, 2000, 24(16): 2685-2691.
[7] YANG Sheng-hai, CHEN Yong-ming, YANG Hai-ping, LIU Yin-yuan, TANG Mo-tang, QIU Guan-zhou. Preparation of high-purity tantalum ethoxide by vacuum distillation [J]. Trans Nonferrous Met Soc China, 2008, 18(4): 196-201.
[8] MEHROTRA R C. Synthesis and reactions of metal alkoxides [J]. Journal of Non-crystalline Solids, 1988, 100(1/3): 1-15.
[9] FRIEDRICH Z. Process for preparing tantalum alkoxides and niobium alkoxides: US 6548685 [P]. 2003-04-15.
[10] VECCHIO-SADUS A M. The electrochemical synthesis of inorganic and organometallic complexes in non-aqueous media [J]. Journal of Applied Electrochemistry, 1993, 23(5): 401-416.
[11] TUROVA N Y, KOROLEV A V, TCHEBUKOV D E, BELOKON A I, YANOVSKY A I, STRUCHKOV Y T. Tantalum(Ⅴ) alkoxides: Electrochemical synthesis, mass-spectral investigation and oxoalkoxocomplexes [J]. Polyhedron, 1996, 15(21): 3869-3880.
[12] YANG Sheng-hai, PAN Ze-qiang, LI Zhao-hui, TANG Mo-tang, QIU Guan-zhou. Electrochemical synthesis of tantalum ethoxide using sacrificing anode [J]. Rare Metal Materials and Engineering, 2006, 35(4): 625-628. (in Chinese)
[13] DOLLISH F R, FATELEY W G, BENTLEY F F. Characteristic Raman frequencies of organic compounds [M] New York: Wiley, 1974: 218.
[14] BRADLEY D C, HOLLOWAY C E. Nuclear magnetic resonance studies on niobium and tantalum penta-alkoxides [J]. J Chem Soc A, 1968: 219-223.
[15] BRADLEY D C. Metal alkoxides as precursors for electronic and ceramic materials [J]. Chemical Reviews, 1989, 89(6): 1317-1322.
[16] HOCHIDO Y, KADOKURA H, TANABE S, KOBAYASHI R. Process for purifying niobium alkoxides and tantalum alkoxides: US 5919963 [P]. 1999-07-06.
Foundation item: Project(2007AA03Z425) supported by the Hi-tech Research and Development Program of China; Project(50404011) supported by the National Natural Science Foundation of China
Corresponding author: YANG Sheng-hai; Tel: +86-731-88830470; E-mail: 75894838@163.com
DOI: 10.1016/S1003-6326(09)60060-1
(Edited by YANG Hua)