
J. Cent. South Univ. (2021) 28: 2463-2471
DOI: https://doi.org/10.1007/s11771-021-4739-1

Periodic electromagnetic signals as potential precursor for seismic activity
YONG Shan-shan(雍珊珊)1, WANG Xin-an(王新安)1, ZHANG Xing(张兴)2, GUO Qin-meng(郭琴梦)1, WANG Jing(王晶)1, YANG Chao(杨超)1, JIANG Bing-hui(蒋冰慧)1
1. Key Laboratory of Integrated Micro-Systems Science and Engineering Applications, Peking University Shenzhen Graduate School, Shenzhen 518055, China;
2. School of Electronics Engineering and Computer Science, Peking University, Beijing 100871, China
Central South University Press and Springer-Verlag GmbH Germany, part of Springer Nature 2021
Abstract: Electromagnetic signals may be a promising precursor to seismic activity which has been observed in many case studies in past decades. However, the correlation and causation between the electromagnetic signals and the seismic activity are still unclear without intensive observation network. In order to find seismoelectromagnetic phenomenon, we deployed AETA (acoustic and electromagnetic testing all-in-one system), a high-density multi-component seismic monitoring system in the China Earthquake Science Experiment site (CESE, in Sichuan Province and Yunnan Province, China) and the capital circle (areas with a distance which is ≤200 km from Beijing), to record electromagnetic and geo-acoustic data across 0.1 Hz-10 kHz. In the course of data collection, we discovered an electromagnetic waveform that occurs on a daily basis. Because the signal generally coincides with sunrise and sunset, we named this phenomenon the SRSS (Sunrise-Sunset) waveform. After conducting three statistical tests based on seismicity and SRSS, we determined that the SRSS waveform is roughly correlated with the onset of seismic activity. It generally occurs at the regions where seismicity occurs. This discovery might have significant implications with respect to the future of earthquake prediction.
Key words: seismic precursor; periodic electromagnetic signal; Sunrise-Sunset (SRSS) waveform
Cite this article as: YONG Shan-shan, WANG Xin-an, ZHANG Xing, GUO Qin-meng, WANG Jing, YANG Chao, JIANG Bing-hui. Periodic electromagnetic signals as potential precursor for seismic activity [J]. Journal of Central South University, 2021, 28(8): 2463-2471. DOI: https://doi.org/10.1007/s11771-021-4739-1.
1 Introduction
Without prior warning, earthquakes, especially those with magnitudes larger than 6.0, can be immensely destructive [1-3]. Scientists have used various instruments to record anomalies prior to earthquakes, and electromagnetic disturbance is one of promising precursors which have been intensively observed and studied during past several decades [4-9]. Both in rock fracture experiments and in field observations, electromagnetic emission and anomalies have been observed [10-21]. According to these case studies, we found that these anomalies appeared from a few months before to a few days after the considered earthquake and they were all imminent anomalies. However, we also found the complexity of electromagnetic disturbance phenomenon before earthquake. Because each abnormal phenomenon before earthquake will be different which results in the difficulties to make accurate earthquake prediction.So, until now, to prove correlation and causation between the electromagnetic signals and the seismic activity is still problematic [22].
In the hope that more data will allow the community to elucidate the relationship between electromagnetic signals and earthquakes, we developed AETA (acoustic and electromagnetic testing all-in-one), a multi-component seismic monitoring system that monitors both electromagnetic disturbances and geo-acoustic signals [23, 24]. AETA consists of an electromagnetic (EM) probe [25], a geo-acoustic (GA) probe [26], a data pre-processing terminal, and an uplink to a cloud server (Figure 1). Data from the two probes are relayed to the cloud server in real time. We also developed a website (www.aeta.cn) and a companion piece of software in order to make the data available to relevant researchers. The parameters of the AETA EM probe are as follows: 0.1 Hz-10 kHz, 0.1-1000 nT electromagnetic signal, sensitivity of >20 mV/nT@ 0.1 Hz-10 kHz, 18-bit resolution, and sampling rates of 500 Hz at low frequency (≤200 Hz) and 30 kHz at full frequency (≤10 kHz) [25].
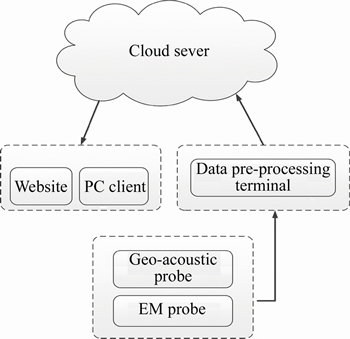
Figure 1 Components of AETA seismic monitoring system
To determine if these electromagnetic disturbance signals and geo-acoustic signals are reliable precursors for earthquakes, we deployed AETA stations in two regions: the China Earthquake Science Experiment site (CESE [27]) and the capital circle (areas with a distance which is ≤200 km from Beijing, China). Earthquakes are common in these two regions; CESE is located in the Sichuan and Yunnan Provinces, China, which are high seismicity areas, and in the capital circle, the 1975 Haicheng ML7.3 earthquake and the 1976 Tangshan ML7.8 earthquake occurred and brought great losses. We placed 152 AETA stations at CESE, and 29 stations throughout capital circle. We also have stations at other regions, like Guangdong Province, Taiwan, Shanxi Province, Gansu Province, Tibet, China, etc. The total number of stations in China is 221.
With the support of the China Earthquake Administration and other local earthquake administrative bodies, we have been generating high-quality electromagnetic and geo-acoustic data since June, 2017. In this paper we will discuss what we found in electromagnetic data.
2 Data
The electromagnetic data (EMD, V) recorded at the AETA stations are available to registered users at www.aeta.cn. This work was conducted at the Research Center of Earthquake Monitoring and Forecasting Technology and the Key Laboratory of Integrated Micro-Systems Science and Engineering Applications at Peking University’s Shenzhen Graduate School in Shenzhen, China (http://web.pkusz.edu.cn/ims).
2.1 Periodic EMD waveform
Since June, 2017, we have recorded EMD for approximately 18 months. Most of the previous studies concerning EMD data have occurred over the course of several months or years [4-6]. However, our data, which are over a much shorter time-span, are much higher in resolution. Figure 2 shows examples of the EMD periodicity at four different stations: LTNC (Hebei Province, China), DJY (Sichuan Province, China), LJHP (Yunnan Province, China), and GLTD (Taiwan Region, China). All these data changes day-repeatedly.
Figure 2(a) shows the daily periodical signal in LTNC station (117.2°E 39.32°N) from June 17, 2018 to June 23, 2018. Figure 2(b) shows the daily periodical signal in DJY station (103.65°E, 30.98°N) from June 17, 2018 to June 23, 2018. Figure 2(c) shows the daily periodical signal in LJHP station (101.26°E, 26.63°N) from June 17, 2018 to June 23, 2018. Figure 2(d) shows the daily periodical signal in GLTD station (121.15°E, 22.75°N) from June 17, 2018 to June 23, 2018.
2.2 SRSS waveform
At some stations (10% of total number of AETA stations, most of them distribute in Sichuan and Yunan Provinces, China, shown in Table 1), we recorded period waveforms, like those shown in Figure 2(c), with peaks that occur within one hour of both sunrise and sunset. We refer to this phenomenon as the SRSS (Sunrise-Sunset) waveform. Examples of the SRSS waveform are shown in Figure 3, where the red and green dashed lines refer to the local sunrise and sunset time at each station, respectively. We can tell that rise time and local sunset time are quite close, fall time & local sunset time are the same. Figure 3(a) shows the SRSS in TSLN station (118.66°E, 39.51°N) from July 10, 2017 to July 16, 2017. Figure 3(b) shows the SRSS in GZ station (99.99°E, 31.62°N) from June 17, 2018 to June 23, 2018. Figure 3(c) shows the SRSS in LJHP station (101.26°E, 26.63°N) from June 17, 2018 to June 23, 2018. Figure 3(d) shows the SRSS in FT station (114.04°E, 22.58°N) from October 29, 2017 to November 4, 2017.
This synchronous phenomenon with sun’s activity caught our attention. We analyzed and compared all periods with SRSS in AETA stations and drew the figure and features of it.
Table 1 List of AETA stations with SRSS more than 15 d from January 1, 2019 to November 7, 2019
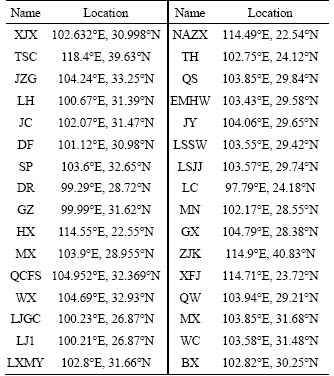
A diagram of the SRSS waveform structure is shown in Figure 4. The periodicity of the SRSS waveform is about 24 h. At sunset (±1 h), the amplitude of the SRSS waveform starts to increase sharply, and at sunrise (±1 h), the amplitude decreases abruptly. The SRSS often takes the form of a square wave. The average value of the amplitude peak (VH) is significantly larger than the average value of the amplitude trough (VL). The exact magnitudes of VH and VL vary throughout the day which is much less than VH-VL.
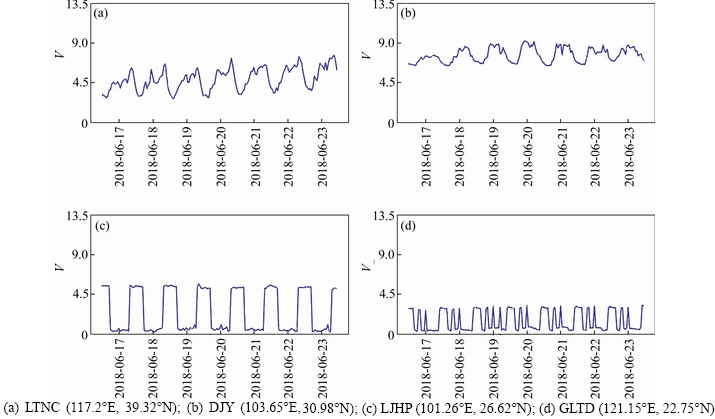
Figure 2 Examples of EMD waveforms with daily periodicity:
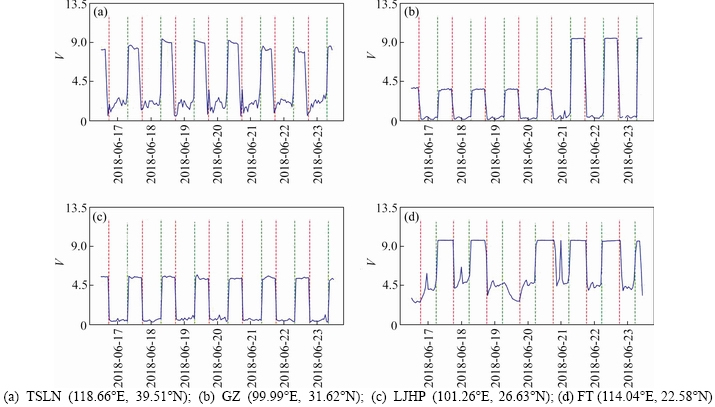
Figure 3 Examples of SRSS:
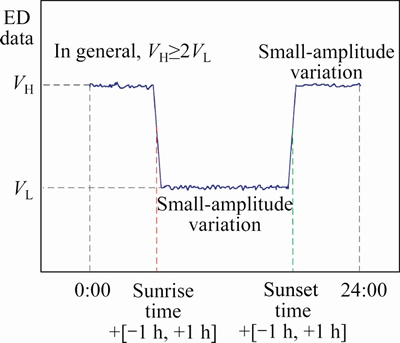
Figure 4 Diagram of typical SRSS waveform
While the SRSS waveform can persist for days to months, then the signal may disappear for days to months. Despite the transient nature of the SRSS signal, we observe similar SRSS signals at multiple stations. For example, on October 22, 2018, we recorded SRSS signals at 11 stations (9 in CESE and 2 in capital circle). On June 17, 2019, we recorded SRSS signals at 16 stations (15 in CESE and 1 in capital circle).
To figure out why this SRSS occurs and is it just a kind of instrument or environmental noise, we go back to the original signals of EMD which is 500 Hz sampled in low frequency band which is ≤200 Hz. There are two kinds of original EMD signals and are different in frequency range. They are 0.1-200 Hz and 0.1 Hz-10 kHz. We found that, for most of stations with SRSS, despite the power frequency signal (50 Hz) and its odd harmonic signal (150 Hz), other frequency signals also change synchronously with sunrise and sunset time. Take the EMD data on NAZX station (114.49°E, 22.54°N) from March 1, 2019 to March 7, 2019 as example, the EMD signal at 50 Hz doesn’t change synchronously with sunset and sunrise, while the 150 Hz EMD signal and others are both synchronous changed with sunset and sunrise. We can tell that this SRSS phenomenon isn’t subjected to environmental or power frequency noise.
It is like there is an unknown field in the space modulate all electromagnetic signals in ≤200 Hz frequency range. And this unknown field does not exist at any time and any place like the sun activity. Although we do not know what it is, we might find its worth through the correlation analysis between it and seismicity according to EMD data.
2.3 Spatio-temporal occurrence of SRSS waveforms
As mentioned previously, we do not observe the SRSS waveforms consistently through time. We choose 13 AETA stations in CESE with SRSS during July 1, 2017 to March 30, 2019. At station QCFS, we observed the SRSS waveform on a nearly daily basis from November 2017 to November 2018. At the other 12 stations, however, the SRSS waveforms occur more infrequently. We infer that these waveforms can be highly localized because we only recorded SRSS waveforms at specific stations, rather than across our entire densely spaced array at any given time. For example, of the 152 AETA stations in the Sichuan and Yunnan provinces, we only recorded SRSS waveforms at 15 of those stations on June 17, 2019. They are JZG(104.24°E, 33.25°N), GZ(99.99°E, 31.62°N), LH(100.67°E, 31.39°N), XJX(102.632°E, 30.998°N), BX(102.82°E, 30.25°N), LSJJ(103.57°E, 29.74°N), QS(103.85°E, 29.84°N), EMHW (103.43°E, 29.58°N), QW(103.94°E, 29.21°N), JY(104.06°E, 29.65°N), DR(99.29°E 28.72°N), MN(102.17°E, 28.55°N), QJ(102.94°E, 26.9°N), LJLD1(100.21°E, 26.87°N), DLHDQ(100.09°E, 25.9°N). Meanwhile, in the duration of SRSS appearance, there is a high frequency of earthquakes appeared and the epicentral distance is less than 100 km as Figure 5 displays. Black stars mark these earthquakes. We will discuss the correlation between SRSS and earthquakes later.
3 Analysis and results
To quantify the correlation between the SRSS waveform and nearby seismic activity, we conduct analyses on both the SRSS waveforms and several recorded seismic signals. When we matched a recorded SRSS waveform with a specific seismic event, we confined our search to earthquakes that occurred within 100 km and 20 d of a specific station’s SRSS signal because we think anomalies in a closer distance and shorter time span contribute more to a coming earthquake than others anomalies which precede the time span and exceed the area range. It is also in accordance with our earthquake prediction aim which is to predict an impeding earthquake within next 20 d for a specific area within 100 km radius.
3.1 SRSS waveforms and seismic activity at QCFS
The Qingchuan Fangshi AETA station (QCFS, 104.96°E, 32.37°N) is located in the CESE study area. Several earthquakes occurred within a 100 km radius of QCFS between November 6, 2017 and December 8, 2018 (Table 2). There are eight earthquakes with a SRSS mutation 0.5 to 19 d ahead. Only 2 earthquakes occurred without SRSS mutation. By our observation, once the SRSS signal has mutated, there is quite possible that an earthquake will occur in the following 20 d. There are eight times of SRSS mutations, each of them followed with an earthquake. The mutation of SRSS means no amplitude change during sunrise and sunset time in one or several days. If the SRSS waveform persists, the likelihood of a nearby seismic event occurring in the next 20 d is very low. Only 2 earthquakes occurred without SRSS mutations ahead. The earthquakes recorded at QCFS over this time interval have a linear spatial trend, with QCFS located at the center of the pattern of earthquake epicenters. There are two main fault zones named Pingwu-Qingchuan fault zone and Yingxiu-Beichuan-Qingchuan fault zone across this region. Whether there is a correlation between these fault zones and earthquakes remains unknown. However, it is possible that the QCFS station was sensitive to the activities of these two fault zones from November 6, 2017 to December 8, 2018. The stable and continuous SRSS waveform indicates their connection. Any change of the fault zone implies a pending earthquake, which was captured by QCFS in the form of an SRSS mutation. In general, when the stable SRSS disappeared and was not observed for a long time, the connection between the QCFS station and the fault zone was dismissed.
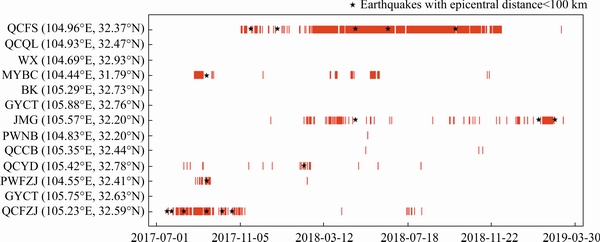
Figure 5 Temporal occurrences of SRSS waveforms at 13 AETA stations
Table 2 List of earthquakes within 100 km of AETA station QCFS (from November 6, 2017 to December 8, 2018) and the occurrence of SRSS waveform mutation
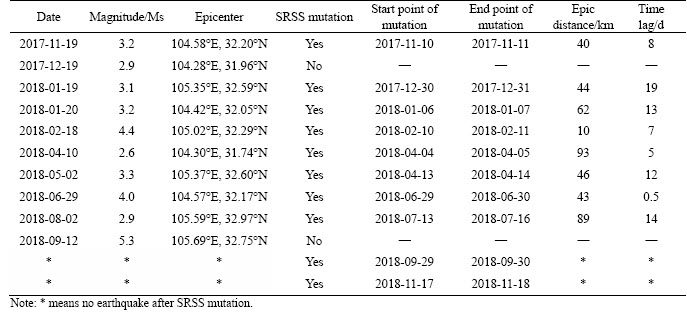
3.2 SRSS waveforms and seismic activity in Sichuan province
We tried to find whether there was a stronger connection with SRSS occurrence and seismicity. So, we conducted analysis between the recorded SRSS signals and the 35 Ms 3.0+ earthquakes (except for the Jiuzhaigou earthquake) that occurred in the Sichuan Province from January 1, 2017 to December 31, 2017. We found that SRSS waveforms were recorded at specific AETA stations as long as these stations were within 100 km of the earthquake epicenters. Of the 35 earthquakes investigated, each earthquake was within 100 km of at least one AETA station, while eight earthquakes occurred within 100 km of one or more AETA stations (Table 3).
3.3 SRSS waveforms and Jiuzhaigou earthquake
The Ms 7 Jiuzhaigou earthquake (103.82°E, 33.20°N) occurred on August 8, 2017 in the Jiuzhaigou County of Sichuan Province. EMD data was obtained at stations located near the earthquake’s epicenter, just prior to the onset of seismic activity. Because of the earthquake’s intensity, we discovered SRSS signals at four AETA stations, some of which had epicentral distances greater than 100 km (Table 4). Before Jiuzhaigou earthquake, there were 36 AETA station in Sichuan Province. In area of a distance of 200 km from epicenter, there were eight AETA stations. Four of them had SRSS. They are JZG, SP, MX and QC stations. According to LU et al [28], an anomaly band was found at JZG station (104.24°E, 33.25°N) during August 3, 2017 to August 23, 2017 based on PCA analysis on SRSS. The epic distance of JZG station is only 40 km. So, the occurrence of SRSS and anomalies distracted from SRSS analysis are very important phenomenon for earthquake risk estimation and prediction.
3.4 Relationship between SRSS waveforms and seismic activity
Based on the above analyses, we can start to define the relationship between the SRSS waveforms and the onset of seismic activity. Firstly,the absence of seismicity within 100 km of an AETA station yields a similar lack of recorded SRSS waveforms. Secondly, it is the mutation of the SRSS waveform, rather than the appearance of the signal itself, that indicates that there is a high probability of an earthquake occurring within 100 km of that station in the following 20 d. Thirdly, SRSS waveforms occurring at multiple nearby stations may be a precursor to a high magnitude earthquake. In the future, more densely spaced AETA stations may be used to capture the SRSS waveform mutation and pinpoint the oncoming earthquake’s epicenter in real time.
Table 3 Eight ≥Ms 3.0 earthquakes in Sichuan Province in 2017 and AETA stations with SRSS in a distance less than 100 km from epicenter
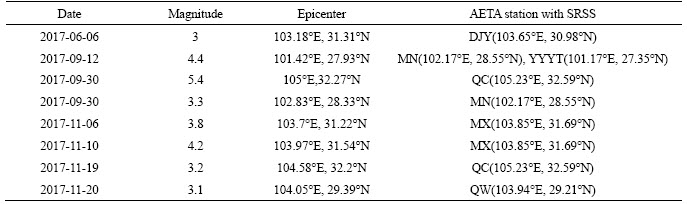
Table 4 AETA stations located within 200 km of Ms 7 Jiuzhaigou earthquake
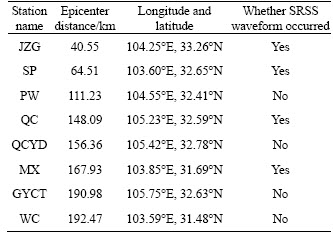
4 Conclusions
In this study, we identified an EMD signal with a daily periodicity that coincides with sunrise and sunset. Based on three case studies, we determined that this SRSS waveform is both spatially and temporally related to the onset of seismic activity. Compared with previous studies, we not only proved that electromagnetic disturbance is a promising precursor, but also found a specific waveform of seismoelectromagnetic disturbance which can be applied earthquake prediction model to get better accuracy of prediction.
While our findings are valuable, we hope to deploy more AETA stations in the future, which will allow us to gather more SRSS waveforms and seismic data. With a larger data set, in conjunction with local geological data that may provide insight into the origin of this EMD signal, we hope to more robustly quantify the connection between the SRSS waveforms and the onset of seismic activity.
Acknowledgements
We appreciate the support and assistance of the China Earthquake Administration, the Sichuan Earthquake Administration (and its subordinate earthquake bureaus), the Yunnan Earthquake Administration (and its subordinate earthquake bureaus), and the Hebei Earthquake Administration (and its subordinate earthquake bureaus), China. We are grateful for the kind support and guidance of YANG Fu-qing and WANG Yang-yuan. We would also like to thank Shenzhen Valley Venture for their service and improvement upon the AETA instrumentation.
Contributors
YONG Shan-shan conceptualized the problem, wrote the main manuscript text, and contributed to some figure preparation. WANG Xin-an contributed to the manuscript framework and reviewed the manuscript. GUO Qin-meng, WANG Jing, YANG Chao and JIANG Bing-hui wrote part of the manuscript text and contributed to some data and figure preparation. ZHANG Xing reviewed the manuscript.
Conflict of interest
YONG Shan-shan, WANG Xin-an, ZHANG Xing, GUO Qin-meng, WANG Jing, YANG Chao, JIANG Bing-hui declare that they have no conflict of interest.
References
[1] PARSONS T, JI Chen, KIRBY E. Stress changes from the 2008 Wenchuan earthquake and increased hazard in the Sichuan basin [J]. Nature, 2008, 454(7203): 509-510. DOI: 10.1038/nature07177.
[2] YUE H, LAY T. Inversion of high-rate (1 sps) GPS data for rupture process of the 11 March 2011 Tohoku earthquake (Mw9.1) [J]. Geophysical Research Letters, 2011, 38(7): 752-767. DOI: 10.1029/2011gl048700.
[3] LI Rui-hao, FU Zhao-zhu. Local gravity variations before and after the Tangshan earthquake (M=7.8) and the dilatation process [J]. Tectonophysics, 1983, 97(1-4): 159-169. DOI: 10.1016/0040-1951(83)90143-9.
[4] HAN Peng, ZHUANG Jian-cang, HATTORI K, CHEN C H, FEBRIANI F, CHEN Hong-yan, YOSHINO C, YOSHIDA S. Assessing the potential earthquake precursory information in ULF magnetic data recorded in Kanto, Japan during 2000–2010: Distance and magnitude dependences [J]. Entropy, 2020, 22(8): 859. DOI: 10.3390/e22080859.
[5] QIU Zhi-cheng, YONG Shan-shan, WANG Xi-nan. On possible electromagnetic precursors to a significant earthquake (Mw=7.0) occurred in Jiuzhaigou (China) on 8 August 2017 [C]//Proceedings of the 2020 The 3rd International Conference on Information Science and System. Cambridge, United Kingdom, New York, NY, USA: ACM, 2020: 229–234. DOI: 10.1145/3388176.3388202.
[6] HELMAN D S. Seismic electric signals (SES) and earthquakes: A review of an updated VAN method and competing hypotheses for SES generation and earthquake triggering [J]. Physics of the Earth and Planetary Interiors, 2020, 302: 106484. DOI: 10.1016/j.pepi.2020.106484.
[7] FLORIOS K, CONTOPOULOS I, CHRISTOFILAKIS V, TATSIS G, CHRONOPOULOS S, REPAPIS C, TRITAKIS V. Pre-seismic electromagnetic perturbations in two earthquakes in northern Greece [J]. Pure and Applied Geophysics, 2020, 177(2): 787-799. DOI: 10.1007/s00024- 019-02362-6.
[8] NIKOLOPOULOS D P E. Electromagnetic pre-earthquake precursors: Mechanisms, data and models-A review [J]. Journal of Earth Science & Climatic Change, 2015, 6(1): 250. DOI: 10.4172/2157-7617.1000250.
[9] HAYAKAWA M, HOBARA Y, OHTA K, HATTORI K. The ultra-low-frequency magnetic disturbances associated with earthquakes [J]. Earthquake Science, 2011, 24(6): 523-534. DOI: 10.1007/s11589-011-0814-2.
[10] FRASER-SMITH A C, BERNARDI A, MCGILL P R, LADD M E, HELLIWELL R A, VILLARD O G Jr. Low-frequency magnetic field measurements near the epicenter of the Ms 7.1 Loma Prieta Earthquake [J]. Geophysical Research Letters, 1990, 17(9): 1465-1468. DOI: 10.1029/GL017i009p01465.
[11] POTIRAKIS S M, SCHEKOTOV A, ASANO T, HAYAKAWA M. Natural time analysis on the ultra-low frequency magnetic field variations prior to the 2016 Kumamoto (Japan) earthquakes [J]. Journal of Asian Earth Sciences, 2018, 154: 419-427. DOI: 10.1016/j.jseaes. 2017.12.036.
[12] SURKOV V V, MOLCHANOV O A, HAYAKAWA M. Pre-earthquake ULF electromagnetic perturbations as a result of inductive seismomagnetic phenomena during microfracturing [J]. Journal of Atmospheric and Solar-Terrestrial Physics, 2003, 65(1): 31-46. DOI: 10.1016/S1364-6826(02)00117-7.
[13] HAYAKAWA M, PULINETS S, PARROT M, MOLCHANOV O A. Recent progress in seismo electromagnetics and related phenomena [J]. Physics and Chemistry of the Earth, Parts A/B/C, 2006, 31(4-9): 129-131. DOI: 10.1016/j.pce.2006.05.001.
[14] CHEN Hua-ran, YANG Dong-mei, LI Qi, ZHU Rong, JIANG Chun, WANG Jian-guo. Observation and research on seismic precursor information of electromagnetic emissions [J]. Earthquake Research in China, 2008, 24(2): 180-186. (in Chinese)
[15] YAMADA I, MASUDA K, MIZUTANI H. Electromagnetic and acoustic emission associated with rock fracture [J]. Physics of the Earth and Planetary Interiors, 1989, 57(1, 2): 157-168. DOI: 10.1016/0031-9201(89)90225-2.
[16] ZHU Tao, ZHOU Jian-guo, WANG Hong-qiang. Electromagnetic emissions during dilating fracture of a rock [J]. Journal of Asian Earth Sciences, 2013, 73: 252-262. DOI: 10.1016/j.jseaes.2013.05.004.
[17] ZHOU Z L, LI X B, WAN G X. The relation between the frequency of electromagnetic radiation (EMR) induced by rock fracture and attribute parameters of rock masses [J]. Chinese Journal of Geophysics, 2009, 52(1): 259-265. (in Chinese)
[18] MA Q Z, FANG G Q, LI W, ZHOU J N. Electromagnetic anomalies before the 2013 Lushan M_S7.0 earthquake [J]. Acta Seismologica Sinica, 2013, 35(5): 717-730. DOI: 10.3969/j.issn.0253-3782.2013.05.010.
[19] XIE T, LIU L, LU J, LI M, YAO L, WANG Y L, YU C. Retrospective analysis on electromagnetic anomalies observed by ground fixed station before the 2008 Wenchuan M_S8.0 earthquake [J]. Chinese Journal of Geophysics, 2018, 65(7): 1922-1937. (in Chinese)
[20] HATTORI K. ULF geomagnetic changes associated with large earthquakes [J]. Terrestrial, Atmospheric and Oceanic Sciences, 2004, 15(3): 329. DOI: 10.3319/tao.2004.15.3. 329(ep).
[21] LIU J Y, CHEN Y I, CHUO Y J, TSAI H F. Variations of ionospheric total electron content during the Chi-Chi Earthquake [J]. Geophysical Research Letters, 2001, 28(7): 1383-1386. DOI: 10.1029/2000GL012511.
[22] HUANG Qing-hua. One possible generation mechanism of co-seismic electric signals [J]. Proceedings of the Japan Academy Ser B: Physical and Biological Sciences, 2002, 78(7): 173-178. DOI: 10.2183/pjab.78.173.
[23] WANG X A, YONG S S, XU B X, LIANG Y W, BAI Z Q, AN H Y, ZHANG X, HUANG J P, XIE Z, LIN K, HE C J, LI Q P. Research and implementation of multi-component seismicmonitoring system AETA [J]. Acta Scientiarum Naturelium Universitatis Pekinensis, 2018, 54: 487-494. (in Chinese)
[24] WANG Xin-an, YONG Shan-shan, HUANG Ji-pan, LU Ya-xuan, ZHANG Xing, LIANG Yi-wen. Earthquake prediction research based on data of ETA [J]. Acta Scientiarum Naturalium Universitatis Pekinensis, 2019, 55(2): 209-214. DOI: 10.13209/j.0479-8023.2019.007. (in Chinese)
[25] YONG Shan-shan, WANG Xin-an, PANG Rui-tao, JIN Xiu-ru, ZENG Jing-wu, HAN Chao-xiang, XU Bo-xing. Development of inductive magnetic sensor for Multi-component seismic monitoring system AETA [J]. Acta Scientiarum Naturelium Universitatis Pekinensis, 2018, 54: 495-501. (in Chinese)
[26] HUANG Ji-pan, YONG Shan-shan, WANG Xin-an, PANG Rui-tao, ZENG Jing-wu. A geo-acoustic sensing probe for seismic monitoring [J]. Acta Scientiarum Naturalium Universitatis Pekinensis, 2020, 56(2): 193-198. (in Chinese)
[27] KE Y L, LIU Y W, ZHANG L, LI Y, CHEN Z, BAO C, LIANG H, CHEN X F, YAO Y. Establishment and analysis of the high-precision hydrogen observation array in China earthquake science experiment site [J]. Earthquake, 2018, 38: 35-48. (in Chinese)
[28] LU Ya-xuan, WANG Xin-an, HUANG Ji-pan, YONG Shan-shan. Research on Jiuzhaigou Ms7.0 earthquake based on earthquake based on AETA electromagnatic disturbance data [J]. Acta Scientiarum Naturalium Universitatis Pekinensis, 2019, 55(6): 1007-1013. DOI: 10.13209/ j.0479-8023.2019.108. (in Chinese)
(Edited by ZHENG Yu-tong)
中文导读
一种反应地震前兆活动的周期性电磁信号
摘要:电磁信号是反应地震前兆活动的重要信号,在过去几十年里有很多观测震例和研究实验。但是,由于没有密集的观测网获取大量的真实数据,电磁信号和地震活动之间的相关性尚不清楚。为了找到地震强相关的电磁现象,在川滇地区和首都圈布设了高密度的多分量地震监测系统(AETA),来观测0.1 Hz~10 kHz的电磁和地声数据。在数据的采集中,发现了电磁信号的波形呈现日周期的特性。该信号的变化与日升日落同步,因此命名为日升日落波SRSS(Sunrise-Sunset)。本文通过三次震例分析,发现SRSS波与地震事件具有强相关性,该信号通常出现在地震活跃的区域。SRSS波与地震相关性对地震预测意义重大。
关键词:地震前兆;周期性电磁信号;SRSS波
Foundation item: Projects(KJYY20170721151955849, JCYJ20190808161401653) supported by Fundamental Research Grant from Shenzhen Science & Technology, China
Received date: 2020-07-07; Accepted date: 2020-11-13
Corresponding author: YONG Shan-shan, PhD, Senior Engineer, Research Assitant; Tel: +86-755-6033003; E-mail: yongshanshan@ pku.edu.cn; ORCID: https://orcid.org/0000-0002-8700-3196