
J. Cent. South Univ. (2018) 25: 141-150
DOI: https://doi.org/10.1007/s11771-018-3724-9

Simulation of long-distance pipeline transportation properties of whole-tailings paste with high sliming
WU Ai-xiang(吴爱祥)1, 2, RUAN Zhu-en(阮竹恩)1, 2, WANG Yi-ming(王贻明)1, 2, YIN Sheng-hua(尹升华)1, 2,
WANG Shao-yong(王少勇)1, 2, WANG Yong(王勇)1, 2, WANG Jian-dong(王建栋)1, 2
1. School of Civil & Resources Engineering, University of Science & Technology Beijing,Beijing 100083, China;
2. Key Laboratory of High-Efficient Mining and Safety of Metal Mines of the Ministry of Education (University of Science & Technology Beijing), Beijing 100083, China
Central South University Press and Springer-Verlag GmbH Germany, part of Springer Nature 2018
Abstract: Based on Bingham rheological model, a three-dimensional numerical simulation model for long-distance pipeline transportation is established by computational fluid dynamics (CFD) to study the pipeline transportation properties of high sliming paste from a copper mine in China. Based on the rheological properties test, the pressure and velocity of pipeline and elbow are simulated by CFD under different mass concentrations and different stowing capacities. The results show that the pipeline resistance of whole-tailings paste with high sliming while without pumping agent is much higher than that with high sliming and pumping agent at the same mass concentration, and the pipeline resistance of whole-tailings paste with high sliming while without pumping agent is much higher than that with low sliming while without pumping agent. It is very important to add pumping agent to whole-tailings paste with high sliming, and the resistance changes with mass concentration and stowing capacity at the same cement-to-sand ratio of 1:5 and tailings-to-waste ratio of 6:1. However, the change is just limited, that is to say, the paste transportation system is of good stability. Furthermore, at the elbow, the maximum pressure and velocity transfer to the outside of the pipe from the inside. However, lubricating layer is formed at the pipe wall because of high content of fine particles in whole-tailings paste, which will protect the elbow from abrasion. CFD provides an intuitive and accurate basis for pipeline transportation study, and would have a wider application space in the study of paste rheological properties and resistance reduction methods.
Key words: high sliming; whole-tailings paste; long-distance transportation; computational fluid dynamics
Cite this article as: WU Ai-xiang, RUAN Zhu-en, WANG Yi-ming, YIN Sheng-hua, WANG Shao-yong, WANG Yong, WANG Jian-dong. Simulation of long-distance pipeline transportation properties of whole-tailings paste with high sliming [J]. Journal of Central South University, 2018, 25(1): 141–150. DOI: https://doi.org/10.1007/s11771- 018-3724-9.
1 Introduction
Cemented paste backfill has been widely approved and used all around the world with the advantages of environment protecting, energy saving, emission reduction, safety and high efficiency. It represents the development trend of mine filling technology, which is regarded as the new technology of green mining in the 21st century [1]. With the increase of the mining depth, the pipeline filling line is gradually developing to the super long and super deep [2]. The first long- distance mineral slurry pipeline in the world was built in Tasmania, Australia in 1967 for the Savage River magnetite mine [3]. Pipeline transportation is one of the key technologies of mine filling, which directly affects the operation state and efficiency of the filling system [4].
Different from the traditional low concentration filling slurry [5], paste is structural flow [6], resulting in the two-phase flow theory cannot be directly used to calculate pipeline resistance of paste. Based on the study of pipeline resistance of two-phase flow and rheological model such as Bingham, many experimental researches in rheological properties and pipeline resistance of structural flow have been carried out. SONG et al [7] and YOU et al [8] investigated rheological properties of cement paste containing ultrafine blast furnace slag. ZHANG et al [9] and WEI et al [10] used viscometer tests to analyze the rheological characteristics and shear thinning phenomena of cemented paste. The effects of superplasticizer on the rheological properties of cemented paste were investigated [11–14]. WANG et al [15] built a new pipe loop test platform to study the influence factors and levels of resistance in tailings slurry pipe transportation, and the influences of pipe inner-diameter, flow velocity, slurry concentration and particle size on pipeline resistance were investigated. YU et al [16] investigated the influencing factors of rheological model and rheological properties of high concentration cemented backfilling slurry. SENAPATI et al [17] studied the rheological and pipeline flow characteristics of copper tailings samples at the mass concentration of 65%–72%, which indicated that non-Newtonian behavior at these high concentrations and the rheological data were best fitted by Bingham plastic model. YAO [18] found that the structural flow of whole-tailings paste slurry has many advantages, such as no segregation, no dehydration, small pipeline resistance, and little abrasion to the pipeline. WU et al [19] analyzed the resistance characteristics of unclassified tailings slurry in pipeline transportation based on structural dynamic theory and Bingham rheological model, and then built a pipeline resistance model to research the resistance behavior of paste backfill pipeline considering the effect of wall slip [20]. LIU et al [4] provided an empirical model for calculating the pipeline resistance of high concentration cemented classified tailing slurry by loop testing and data analysis. IHLE [21] analyzed the efficient combined use of water and energy in long-distance slurry pipelines in light of the total cost function resulting from an energy and mass balance.
With the development of computer technology, computational fluid dynamics (CFD) has gradually been applied to study the transportation properties of paste or paste-like slurry. CFD can quantitatively describe the numerical solution of flow field in time and space and solve the limitation of equipment and cost used in traditional fluid research [22]. WANG et al [23] studied the pipeline transportation properties of paste-like slurry in deep backfilling system by Fluent. Nevertheless, the simulation model was a two-dimensional dynamic model. DENG et al [24] used 2D-double-precision solver Fluent2ddp to analyze the properties of slurry with different concentrations in long-distance pipeline, such as flow pressure, flow rate and deflection characteristic. WU et al [25] established a three- dimensional(3D) model of real pipeline to analyze the resistance of the pipeline and the force condition of the elbow based on solid–liquid two-phase flow theory and CFD. KAUSHAL et al [26] used Eulerian two-phase model to simulate the pipeline slurry flow of mono-dispersed fine particles at high concentration with velocity and slip-velocity distributions being predicted. CHEN et al [27] used Eulerian multiphase model to study constituent particle concentration distribution and velocity distribution as well as pressure gradients of coal-water slurries in horizontal pipelines based on kinetic theory of granular flow. CHEN et al [2] developed a 3D model, W type and long-distance backfilling pipeline transportation model to study the pressure and velocity of pipeline and elbow by CFD. CHEN et al [28] constructed a hydraulic gradient model to calculate the hydraulic gradient of paste-like crude tailings backfill slurry transported by a pipeline system in a mine based on rheological model derivation, numerical simulation, and regression analysis. BANDYOPADHYAY et al [29] analyzed the resistance across the horizontal pipeline and different piping components using CFD, proving that the CFD application is an effective tool for design in the pipeline and piping components. Although CFD is widely used in numerical simulation of pipeline transportation of paste or paste-like slurry,but most of the researches are based on multiphase flow model,which cannot reveal the rheological properties of paste slurry in pipeline thoroughly.
Based on the above analyses, a three- dimensional numerical simulation model for long- distance pipeline transportation is established by CFD and Bingham rheological model, to study the pipeline transportation properties of whole-tailings paste with high sliming from a copper mine in China. According to the actual situation of mine paste filling system, the cement-to-sand ratio of paste and tailings-to-waste ratio are 1:5 and 6:1, respectively at the mass concentration range of 73%–76%.
2 Materials and methods
2.1 Rheological properties of whole-tailings paste with high sliming
2.1.1 Basic properties
Whole-tailings and waste rock from a copper mine in China are the main filling materials, the basic properties of which are shown in Table 1. The particle size distribution curves of whole-tailings and waste rock are shown in Figure 1.
The content of fine particles (<20 μm) of whole-tailings is 29.8%, and the average diameter of whole-tailings is 58.3 μm. The content of mud is high because the whole-tailing is extremely sliming [30]. The coefficient of uniformity(Cu) and coefficient of curvature(Cc) for whole-tailings are 18.36 and 1.62, respectively, which indicates that the whole-tailing has a wide range and a good continuity of particle size distribution. They are shape parameters and calculated using the following equations [31].
(1)
(2)
where D60 is grain diameter at 60% passing, D30 is grain diameter at 30% passing and D10 is grain diameter at 10% passing.
2.1.2 Rheological properties
Characterized with high mass concentration, low moisture content, homogeneity and stability, the shear stress of the whole-tailing paste is linear with shear ratio, indicating that the paste is Bingham fluid. The Rheological model of paste is Bingham model as follows.
(3)
where τ is shear stress, τ0 is yield stress, η is plastic viscosity, and γ is shear ratio.
According to the proportioning scheme shown in Table 2, whole-tailing paste was prepared at 4 different mass concentrations (Case 1–4). Another whole-tailing with low sliming from a lead-zinc mine in China was used as a control (Case 5). Then the rheological parameters were measured by Brookfield R/S+ Rheometer. Without pumping agent, the yield stress of paste with high sliming (Case 1–4) is much higher than that of paste with low sliming (Case 5), and yield stress of paste with high sliming increases with mass concentration.
Table 1 Basic parameters of whole-tailings and waste rock

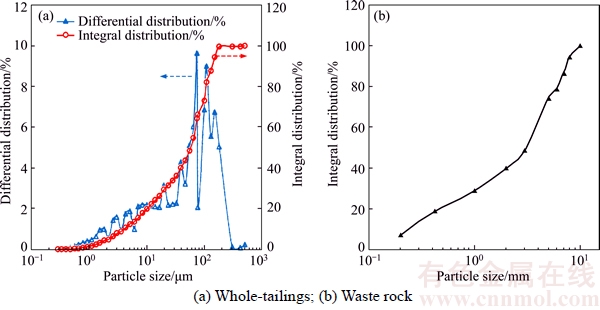
Figure 1 Particle size distribution curve:
Table 2 Proportioning scheme and rheological parameters of whole-tailings paste
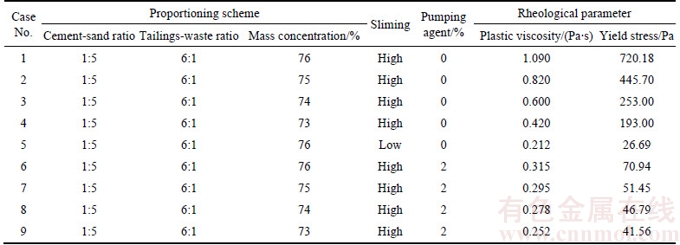
In engineering application, the yield stress of paste should not be more than 200 Pa [32]. However, the yield stress of paste at the lowest mass concentration (73%) is up to 193 Pa. To meet the requirements of the mass concentration of the copper mine (>73%), the pumping agent JK-5 was used to improve the fluidity and pumpability of the paste, and the rheological parameters of paste with 2% pumping agent were measured (Case 6–9). Obviously, the plastic viscosity and yield stress decline greatly while the fluidity is improved with pumping agent added.
2.2 CFD simulation of long-distance pipeline transportation
2.2.1 Physical model
A 3D model of real pipeline from a copper mine in China was established using the preprocessor Gambit (as shown in Figure 2). The inner diameter of filling pipeline is 0.178 m, and the length of filling pipeline is 2599 m, of which the length of vertical pipeline is 360 m and the length of horizontal pipeline is 2239 m. The stowing gradient is 7.22. The four straight pipelines are connected with three circular arc elbows with 90°, the radius of which is 0.5 m. The center angle of the first elbow is slightly larger than 90°. Then the mesh was generated using Gambit, and the grid number 4773753 are used for the simulation.
2.2.2 Mathematical model
2.2.2.1 Inlet velocity
The inlet velocity is calculated by the following equation.
(4)
where Q is inlet flow, D is inner diameter of inlet, and u is inlet velocity.
As the designed stowing capacity is 70–90 m3/h and the inner diameter of the pipeline is 0.178 m, the inlet velocity can be calculated as 1 m/s approximately.
2.2.2.2 Reynolds number
The whole-tailings paste with high sliming is non-Newtonian fluid, and the Reynolds number was calculated by the following equation, which is defined by plastic viscosity.
(5)
where Re is Reynolds number, ρ is the density of whole-tailings paste, u is velocity of whole-tailing paste, D is inner diameter of inlet, and μ is viscosity.
According to the rheological parameters and velocity, the Reynolds number is less than 2300, indicating that the motion state of whole-tailing paste is laminar flow [33].
2.2.2.3 Boundary conditions
The boundary conditions are as follows [34]: standard boundary conditions, velocity inlet boundary conditions and pressure outlet boundary conditions.
Material properties are defined using User- Defined Fan Model in Fluent based on the rheological parameters and Bingham rheological model.
2.2.2.4 Govern equations
Under pump pressure and self-weight, whole-tailing paste flows in pipeline with resistance. Continuity of Eq. (6), momentum of Eq. (7) and pressure of Eq. (8) are used in CFD to satisfy the boundary conditions.
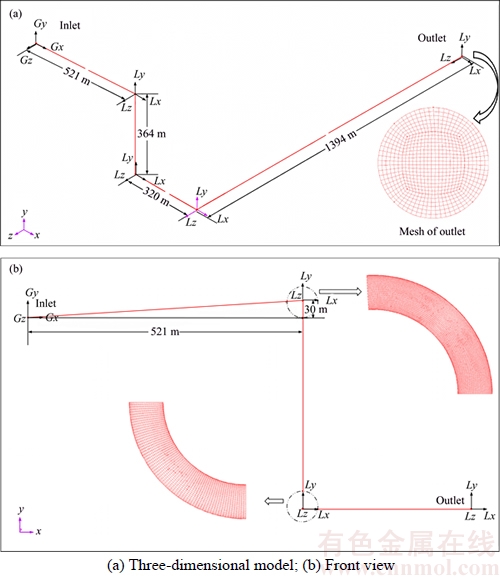
Figure 2 Chart of backfilling pipeline and gridding of elbow:
(6)
(7)
(8)
where t is time, ρ is density of whole-tailings paste, u is velocity of whole-tailings paste, Fx, Fy and Fz are surface force in each direction of micro fluid element, F is force on the micro fluid element, μ is viscosity, P1 and P2 are pressures at corresponding positions, g is gravity acceleration, hp is resistance from position z1 to position z2, and γ is unit weight of whole-tailings paste.
2.2.3 Basic assumptions
The following basic assumptions are necessary for the simulation due to the limitation of the software [35]: 1) The plastic viscosity of whole- tailings paste does not change with temperature and time, that is to say, it is constant; 2) The paste is a homogeneous and incompressible flow; 3) Heat exchange during transporting process is neglected; 4) Effects of vibration and ground pressure wave during the transporting process are also ignored.
3 Results and discussion
3.1 Pipeline resistance
Resistance is a main parameter in the design of paste slurry pipeline transportation system, and accurate prediction of resistance is an important work [36]. The resistance of pipeline under different conditions is shown in Figure 3.
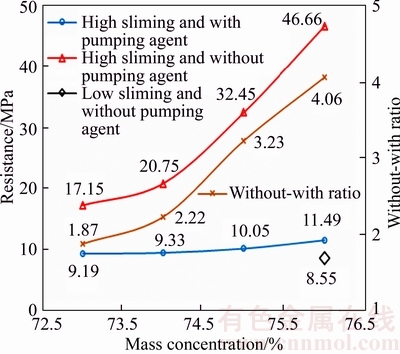
Figure 3 Resistance of different studied cases (Without- with ratio is the ratio of resistance without pumping agent to resistance with pumping agent)
The resistance increases with the mass concentration whether the pumping agent added or not because the yield stress increases with the mass concentration. And the increasing rate also increases with the mass concentration at the same time. When the mass concentration increases by 1% (from 75% to 76%), the increase amount of increasing rate is almost the same as the decrease amount when the mass concentration decreases by 2% (from 75% to 73%) that is the “plus one and minus two” law of precise control of paste mass concentration.
Under the same proportioning scheme, the resistance of the paste without pumping agent is 2–4 times of that with pumping agent. The resistance is as high as 46.66 MPa at the mass concentration of 76%, and the resistance reaches to 17.15 MPa even at the mass concentration of 73%, which is much higher than the rated delivery pressure (16 MPa) of common pump [37] and the resistance (8.55 MPa) of the control case (Case 5). It is important to add pumping agent to the whole-tailings paste to reduce the resistance. With pumping agent of 2%, the resistance of paste at the mass concentration of 76% is only 11.4 MPa. However, even with pumping agent, the resistance of the paste with high sliming at the mass concentration of 73% is higher than that with low sliming at the mass concentration of 76%. That is because the high content of fine particles (<20 μm) of whole-tailings paste, which increases the viscosity, resulting in resistance increase.
At the same time, the resistance of whole- tailings paste changes little with mass concentration after pumping agent is added. The resistance is stable at around 10 MPa.
The resistance under the stowing capacity range of 70–110 m3/h (the velocity range of 0.7– 1.1 m/s) was investigated. The results are shown in Figure 4, from which it can be seen that the resistance increases with velocity. However, it is stable at around 11 MPa.
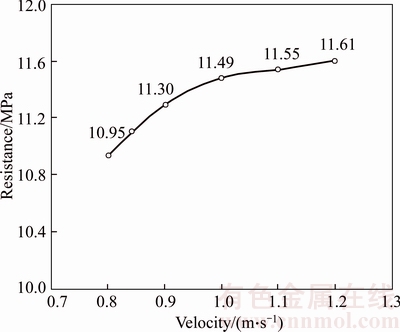
Figure 4 Resistance under different velocities
3.2 Velocity of paste in pipeline
When mass concentration is the highest and pumping agent is added, it is taken as the research object (Case 6). The flow velocity in the 4 straight pipelines is analyzed. It can be seen from Figure 5 that the velocity at the center of the pipeline is the largest and stable, and the flow velocity at the pipe wall decreases to 0 rapidly. The motion state of whole-tailing paste in straight pipeline is “plunger flow”, and the central zone where the velocity changes a little is flow core zone.
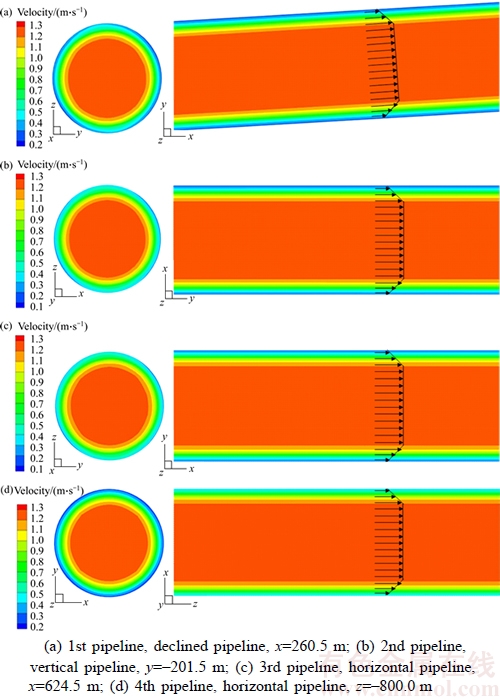
Figure 5 Velocity contours in 4 pipelines in case 6:
To ensure the stability of the paste in pipeline transportation, the content of fine particles (<20 μm) should not be less than 15% [38]. In this work, the content of fine particles is 29.8%, resulting in a lubricating layer with very low viscosity produced. As a result, the resistance of the whole-tailings paste decreased and the transport performance improved to some extent.
3.3 Velocity and pressure of paste in elbow
For case 6, the pressure and velocity in the 3 elbows are analyzed.
Velocity contours in 3 elbows in case 6 are shown in Figures 6(a), (c) and (e). It can be seen that the motion state of whole-tailings paste in straight pipeline is not “plunger flow” anymore, and the velocity increases rapidly from the inside to the outside, resulting in the increase of dynamic pressure. The paste impacts the wall heavily and the resistance increases, which may cause wall abrasion. However, a lubricating layer with very low viscosity is produced because of high content of fine particles. The velocity and viscosity in lubricating layer are very low, which protects the wall from abrasion and is quite different from serious abrasion of common pipeline transportation [3, 24–25].
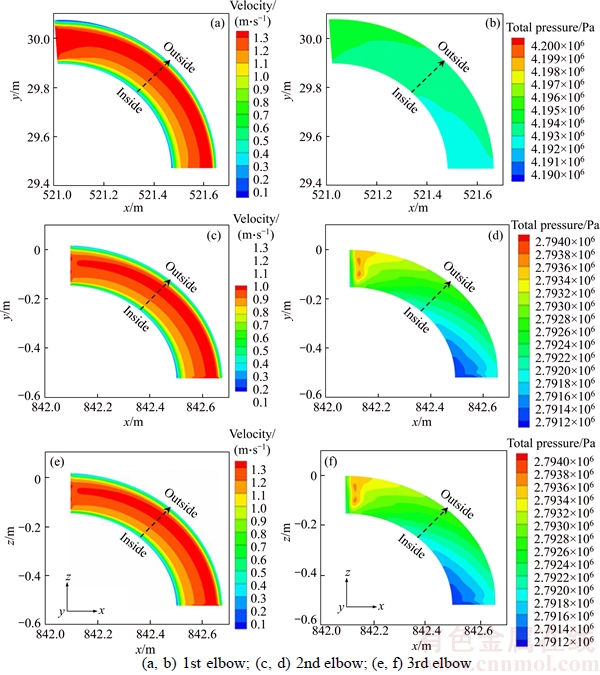
Figure 6 Velocity contours (a, c, e) and pressure contours (b, d, f) in 3 elbows in case 6:
Pressure contours in 3 elbows in case 6 are shown in Figures 6(b), (d) and (f). Because of inertia force, there is a big differential between the pressure at the inlet and that at the outlet of the elbow, indicating that there is big resistance of paste at the elbow. The pressure at the outside of the elbow is larger than that at the inside, resulting in heavier wall abrasion at the outside.
4 Conclusions
1) CFD is used to study the pipeline transportation properties of whole-tailings paste with high sliming. The resistance of the paste without pumping agent is 2–4 times of that with pumping agent under the same proportioning scheme and is much higher than the rated delivery pressure (16 MPa) of common pump. The content of fine particles (<20 μm) of whole-tailings paste is so high that the viscosity and resistance increase, and the resistance of the paste with high sliming is much higher than that with low sliming. That is because the high content of fine particles (<20 μm) of whole-tailings paste increases the viscosity, resulting in resistance increase. It is especially important to add pumping agent to the whole-tailings paste with high sliming to reduce the resistance and improve the fluidity.
2) The resistance increases with the mass concentration and stowing capacity. However, it changes little. The paste transportation system is of good stability at the cement-to-sand ratio of 1:5, the tailings-to-waste ratio of 6:1, the mass concentration range of 73%–76% and the stowing capacity range of 70–110 m3/h (the velocity range of 0.7–1.1 m/s).
3) The motion state of whole-tailings paste in straight pipeline is "plunger flow" while that in elbow is not. Furthermore, at the elbow, the maximum pressure and velocity transfer to the outside of the elbow from the inside. However, lubricating layer with low viscosity and velocity is formed at the pipe wall because of high content of fine particles in whole-tailings paste, which will protect the elbow from abrasion.
4) The study of the pipeline transportation properties of whole-tailings paste with high sliming based on experiment and CFD simulation provides a new method for the research and design of the pipeline transportation of paste. CFD provides an intuitive and accurate basis for pipeline transportation study, and would have a wider application space in the study of paste rheological properties and resistance reduction methods.
References
[1] WU Ai-xiang, WANG Yong, WANG Hong-jiang. Status and prospects of the paste backfill technology [J]. Metal Mine, 2016, 45(7): 1–9. (in Chinese)
[2] CHEN Xin, SHI Xiu-zhi, ZHOU Jian, QIU Xian-yang, LIU Xue-fei. CFD simulation of transportation properties of W type and long distance pipeline for foam slurry backfilling [J]. Chinese Journal of Nonferrous Metals, 2016, 26(8): 1782–1793. (in Chinese)
[3] COWPER S N T, COWPER J N T, THOMAS A D. Slurry Pipelines: Past, present and future [J]. Australian Journal of Multi-Disciplinary Engineering, 2009, 7(2): 189–196.
[4] LIU Xiao-hui, WANG Guo-li, ZHAO Zhan-bin, WANG Shao-yong. Study on the flow resistance characteristics of structure fluid backfilling slurry based on loop pipe testing [J]. China Molybdenum Industry, 2016, 40(5): 20–23. (in Chinese)
[5] ZHANG Xiu-xiang, QIAO Deng-pan. Simulation and experiment of pipeline transportation of high density filling slurry with coarse aggregates [J]. Chinese Journal of Nonferrous Metals, 2015, 25(1): 258–267. (in Chinese)
[6] WU Ai-xiang, LIU Xiao-hui, WANG Hong-jiang, WANG Yi-ming, JIAO Hua-zhe, LIU Shi-zhong. Resistance characteristics of structure fluid backfilling slurry in pipeline transport [J]. Journal of Central South University: Science and Technology, 2014, 45(12): 4325–4330. (in Chinese)
[7] SONG J T, SHIN J H. Rheological properties of cement pastes containing mineral admixtures and superplasticizer [J]. Journal of the Korean Ceramic Society, 2005, 42(11): 737–742.
[8] YOU C D, BYUN S H, SONG J T. Rheological properties of cement paste containing ultrafine blast furnace slag [J]. Journal of the Korean Ceramic Society, 2007, 44(8): 430–436.
[9] ZHANG Qin-li, CHEN Qiu-song, WANG Xin-min. Cemented backfilling technology of paste-like based on aeolian sand and tailings [J]. Minerals, 2016, 6(4): 132–141.
[10] WEI Chong, WANG Xin-min, ZHANG Yun-hai, ZHANG Qin-li. Paste-like cemented backfilling technology and rheological characteristics analysis based on jigging sands [J]. Journal of Central South University, 2017, 24(1): 155-167.
[11] MIKANOVIC N, JOLICOEUR C. Influence of super plasticizers on the rheology and stability of limestone and cement pastes [J]. Cement & Concrete Research, 2008, 38(7): 907–919.
[12] JAYASREE C, KRISHNAN J M, GETTU R. Influence of superplasticizer on the non-Newtonian characteristics of cement paste [J]. Materials and Structures, 2011, 44(5): 929–942.
[13] OUATTARA D, YAHIA A, MBONIMPA M, BELEM T. Effects of superplasticizer on rheological properties of cemented paste backfills [J]. International Journal of Mineral Processing, 2017, 161(4): 28–40.
[14] PAPO A, PIANI L, RICCERI R. Rheological properties of very high-strength portland cement pastes: Influence of very effective superplasticizers [EB/OL]. [2010-01-25] International Journal of Chemical Engineering, http://dx.doi. org/10.1155/2010/ 682914.
[15] WANG Shao-yong, WU Ai-xiang, YIN Sheng-hua, HAN Bin, WANG Hong-jiang. Influence factors of pressure loss in pipeline transportation of paste slurry [J]. Chinese Journal of Engineering, 2015,37(1): 7–12. (in Chinese)
[16] YU Yue, CHENG Kun, PANG Bo. Rheological properties of high concentration cemented backfilling slurry [J]. Electronic Journal of Geotechnical Engineering, 2016, 21(7): 1611–1620.
[17] SENAPATI P K, MISHRA B K. Feasibility studies on pipeline disposal of concentrated copper tailings slurry for waste minimization [J]. Journal of the Institution of Engineers, 2016: 1–7.
[18] YAO Zhong-liang. Application and prospect of structural fluid cemented filling technology [J]. Mining Research and Development, 2016(10): 39–45. (in Chinese)
[19] WU Ai-xiang, LIU Xiao-hui, WANG Hong-jiang, WANG Yi-ming, JIAO Hua-zhe, LIU Shi-zhong. Resistance characteristics of structure fluid backfilling slurry in pipeline transport [J]. Journal of Central South University (Science and Technology), 2014, 45(12): 4325–4330. (in Chinese)
[20] WU Ai-xiang, CHENG Hai-yong, WANG Yi-ming, WANG Hong-jiang, LIU Xiao-hui, LI Gong-cheng. Transport resistance characteristic of paste pipeline considering effect of wall slip [J]. The Chinese Journal of Nonferrous Metals, 2016, 26(1): 180–187. (in Chinese)
[21] IHLE C F. The least energy and water cost condition for turbulent, homogeneous pipeline slurry transport [J]. International Journal of Mineral Processing, 2016, 148: 59–64.
[22] HURTADO J P,
E I. CFD analysis of 58 Adit main fans parallel installation for the 2015–2019 underground developments of the new level mine project [J]. Applied Thermal Engineering, 2015, 90(4): 1109–1118.
[23] WANG Xin-min, ZHAO Jian-wen, XUE Jun-hua, YU Guo-feng. Features of pipe transportation of paste-like backfilling in deep mine [J]. Journal of Central South University of Technology, 2011, 18(5): 1413–1417.
[24] DENG Dai-qiang, ZHU Yong-jian, WANG Fa-zhi, WANG Li, YU Wei-jian. Transportation numerical simulation of filling slurry in long distance pipeline [J]. Journal of Anhui University (Nature Science Edition), 2012, 36(6): 36–43. (in Chinese)
[25] WU Di, CAI Si-jing, YANG Wei, WANG Wen-xiao, WANG Zhang. Simulation and experiment of backfilling pipeline transportation of solid-liquid two-phase flow based on CFD [J]. Chinese Journal of Nonferrous Metals, 2012, 22(7): 2133–2140. (in Chinese)
[26] KAUSHAL D R, THINGLAS T, TOMITA Y, KUCHII S, TSUKAMOTO H. CFD modeling for pipeline flow of fine particles at high concentration [J]. International Journal of Multiphase Flow, 2012, 43: 85–100.
[27] CHEN Liang-yong, DUAN Yu-feng, PU Wen-hao, ZHAO Chang-sui. CFD simulation of coal-water slurry flowing in horizontal pipelines [J]. Korean Journal of Chemical Engineering, 2009, 26(4): 1144–1154.
[28] CHEN Qiu-song, ZHANG Qin-li, WANG Xin-min, XIAO Chong-chun, HU Qing. A hydraulic gradient model of paste-like crude tailings backfill slurry transported by a pipeline system [J]. Environmental Earth Sciences, 2016, 75(14): 1099.
[29] BANDYOPADHYAY T K, DAS S K. Non-Newtonian liquid flow through small diameter piping components: CFD analysis [J]. Journal of the Institution of Engineers, 2016(5): 1–11.
[30] XING Peng, YANG Xi-xiang, WANG Hong-jiang, ZHOU Fa-lu, WANG Shao-yong, CHU Yu-sha. Research on flocs selection of tailings with high sliming in Baishitamu copper mine [J]. Modern Mining, 2016(3): 49–52. (in Chinese)
[31] POPESCU M E. An introduction to geotechnical engineering [J]. Engineering Geology, 1981, 22(4): 377–377.
[32] CLAYTON S, GRICE T G, BOGER D V. Analysis of the slump test for on-site yield stress measurement of mineral suspensions [J]. International Journal of Mineral Processing, 2003, 70(1–4): 3–21.
[33] ZHANG Qin-li, LIU Qi, ZHAO Jian-wen, LIU Jian-gang. Pipeline transportation characteristics of filling paste-like slurry pipeline in deep mine [J]. The Chinese Journal of Nonferrous Metals, 2015, 25(11): 3190-3195. (in Chinese)
[34] KURNIA J C, SASMITO A P, MUJUMDAR A S. CFD simulation of methane dispersion and innovative methane management in underground mining faces [J]. Applied Mathematical Modelling, 2014, 38(14): 3467–3484.
[35] WANG Xin-min,DING De-qiang, WU Ya-bing, ZHANG Qin-li, LU Yang-zhe.Numerical simulation and analysis of paste backfilling with piping transport [J]. China Mining Magazine, 2006, 15(7): 57–59. (in Chinese)
[36] MA Shuai, WANG Xiao-dong, WU Jian-de, LENG Ting-ting, MA Jun, JIANG Di. Study on resistance loss characteristics of iron concentrate pipeline transportation [C]// Control and Decision Conference. IEEE, 2015: 2037–2041.
[37] WU Ai-xiang, WANG Hong-jiang. Theory and technology of metal cemented paste backfill [M]. Beijing, Science Press, 2015: 331–347. (in Chinese)
[38] LANDRIAULT D. Paste backfill mix design for Canadian underground hard rock mining [C]// 97th Annual General Meeting of CIM, Rock Mechanics and Strata Control Session. Halifax, Nova Scotia, 1995: 229–238.
(Edited by FANG Jing-hua)
中文导读
高含泥全尾砂膏体长距离输送CFD模拟研究
摘要:针对高含泥膏体管道输送特性研究问题,以国内某铜矿为研究对象,基于宾汉姆流变模型,运用计算流体力学(CFD)建立高含泥膏体长距离管道输送性能研究的3D数值分析模型。在室内流变特性试验的基础上,对不同浓度、不同充填能力料浆输送中管道和弯管处压力、速度进行CFD模拟分析。结果表明:不添加泵送剂的高含泥膏体的管道输送阻力损失远高于添加泵送剂的高含泥膏体阻力损失和不添加泵送剂的低含泥膏体阻力损失,这对于高含泥膏体添加泵送剂尤为重要。当灰砂比为1:5、尾废比为6:1、浓度为73%–76%时充填能力为70–110 m3/h,阻力损失变化不大,输送系统相对稳定。通过弯管处压力、速度模拟可得,管道内压力和速度最大值点由管道中心线向管道内下侧方偏移。但是由于细粒级颗粒含量高,在管壁处形成润滑层,对弯管有一定的保护作用。CFD能够为膏体管道输送研究提供直观准确的依据,在膏体流变特性和降阻方法创新方面还有更为广阔的应用空间。
关键词:高含泥;全尾砂膏体;长距离输送;计算流体动力学
Foundation item: Project(2016YFC0600709) supported by the National Key R&D Program of China; Project(51574013) supported by the National Natural Science Foundation of China; Project(FRF-TP-17-024A1) supported by the Fundamental Research Funds for the Central Universities, China
Received date: 2017–03-27; Accepted date: 2017-07-21
Corresponding author: RUAN Zhu-en; Tel: +86-10–62334680; E-mail: ziyuan0902rze@163.com