
Transient oxidation behavior of nanocrystalline CoCrAlY coating at 1 050 ℃
LIU Guang-ming(刘光明)1, 2, LI Mei-shuan(李美栓)1, MA Jing-hui(马敬翙)2,
DU Nan(杜 楠)2, ZHOU Yan-chun(周延春)1
1. Shenyang National Laboratory for Materials Science, Institute of Metal Research, Chinese Academy of Sciences, Shenyang 110016, China;
2. School of Materials Science and Engineering, Nanchang Institute of Aeronautical Technology,
Nanchang 330034, China
Received 23 October 2006; accepted 2 February 2007
Abstract: Nanocrystalline CoCrAlY overlay coating was prepared on M38G superalloy by magnetron sputtering deposition. To investigate the oxidation behavior and phase transformation of alumina during oxidation, the oxidation experiments were conducted at 1 050 ℃ for various time in the range of 5-180 min. The phase compositions of the oxide scales were investigated by using glancing angle X-ray diffraction(XRD). The microstructure analysis of oxide scales was carried out by means of scanning electron microscopy(SEM). The growth process of metastable alumina at the grain boundaries and transformation to stable alumina were discussed. The results show that at the initial oxidation stage the mixture of δ-Al
2O
3,
g-Al
2O
3 and α-Al
2O
3 is formed on the sample surface rapidly. Especially, δ-Al
2O
3 and g-Al
2O
3 prefer growing at the grain boundaries of CoCrAlY coating. With increasing oxidation time, δ-Al
2O
3 and g-Al
2O
3 transform to θ-Al
2O
3, afterwards θ-Al
2O
3 transforms to α-Al
2O
3 gradually. After 180 min oxidation, θ-Al
2O
3 transforms into α-Al
2O
3 completely.
Key words: CoCrAlY coating; oxidation; Al2O3; phase transformation
1 Introduction
MCrAlY (M=Co, Ni, Fe or their combination) overlay coatings are widely used in superalloy components for high temperature applications in gas turbine technology to protect the blade surfaces against oxidation and hot corrosion[1-2]. In addition, this kind of coatings can serve as bond layers for thermal barrier coatings on superalloy substrates[3-4]. It was reported that during oxidation only alumina layer formed on nanocrystalline CoCrAlY coatings with fine grains, however, on these alloys with the same composition but with grain sizes of several microns formed a mixture scale of chromium oxide, spinels and alumina[5]. Because of the formation of a continuous, stable and slow growing protective alumina layer, fine-grain sputtered coatings possess the improved oxidation resistance[6-7].
However, it is usually found that g-, θ-alumina grains form on superalloys or coatings of MCrAlY, especially β-NiAl coatings when oxidized at tempera- tures below 1 000 ℃, and the presence of metastable alumina, such as g-Al2O3 and θ-Al2O3, causes fast oxidation kinetics[8-12]. Metastable alumina phases also appear at higher temperatures for short time oxidation[10]. Similar phase transformation of metastable alumina to stable alumina was observed during oxidation of nanocrystalline CoCrAlY coatings at high temperature[10]. Hence, the alumina phase transformation may play a crucial role for high temperature oxidation resistance of these technologically important nanocrystalline CoCrAlY coatings.
The oxidation behaviors of nanocrystalline CoCrAlY coatings were studied by PENG et al[10]. However, more details about the formation and phase transformation of the metastable alumina on the coatings at the initial oxidation stage are not available. In the present work, the formation of alumina and phase transformation of metastable phase to α-Al2O3 during transient oxidation of nanocrystalline CoCrAlY coatings were investigated by means of glancing angle X-ray diffraction(XRD) and scanning electron microscopy (SEM).
2 Experimental
The Ni-based superalloy M38G was used as substrate material, and its nominal compositions (mass fraction, %) are as follows: Ni bal; Cr 16.0; Al 4.0; Co 8.5; W 2.0; Ti 3.8; Mo 1.7; Nb 0.7; Ta 1.7; C 0.17; B 0.01; P <0.001. Rectangular specimens with dimen- sions of 10 mm×20 mm×2.5 mm were cut by the electrical-discharge method. The surfaces were polished down to 800# SiC paper. The specimens were cleaned in acetone, ethanol and distilled water with an ultrasonic bath before magnetron sputtering deposition.
Magnetron sputtering deposition was used to prepare Co-30Cr-6Al-0.2Y (mass fraction, %) coating on M38G superalloy. The process was described in detail in Ref.[13]. The average grain size of the coating was less than 100 nm.
The isothermal oxidation experiments were conducted at 1 050 ℃ in air for 5, 10, 20, 40, 90 and 180 min, respectively. The oxide scales formed on the samples were very thin at the early oxidation stage. In order to examine the phase compositions of these thin films, glancing angle (0.5? incident angle) X-ray diffraction(XRD) was employed. The microstructures of surface morphologies were observed by using scanning electron microscope(SEM) equipped with an energy dispersive spectroscope(EDS).
3 Results and discussion
3.1 Oxidation kinetics
Fig.1(a) shows the kinetics curve of the as-prepared sample as a function of time during oxidation at 1 050 ℃. In order to probe the oxidation mechanism of nanocrystalline CoCrAlY coating, the quartic curve of mass gain per unit area (Δm)4 as a function of time was plotted in Fig.1(b). It is seen that the initial oxidation kinetics at 1 050 ℃ is in quite good agreement with quartic rate equation:
(Δm)4=kt (t<180 min) (1)
where k=8.89×10-5 mg4×cm-8×min-1.
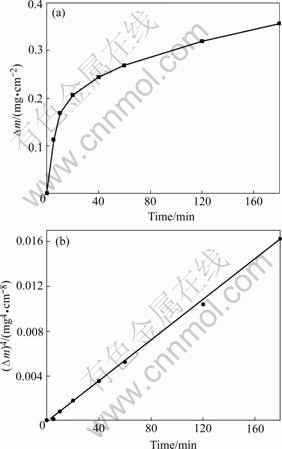
Fig.1 Oxidation kinetics curve (a) and quartic curve of mass gain per unit area (b) of as-prepared sample at 1 050 ℃ as function of time
Generally, during high temperature oxidation, the formation of a dense oxide scale on the metal surface separates substrate alloy and the oxygen gas. If sufficient oxygen partial pressure was available on the oxide surface, the oxidation rate of these alloys at high temperatures would be limited by solid-state diffusional transport of the reactants or electrons through the scale. Under this condition, the oxidation kinetics obeyed parabolic rate law[14]. However, when nanocrystalline CoCrAlY coating was oxidized, oxidation kinetics followed quartic rate equation. The phenomenon was related to the special structure of this kind of nanocrystalline coating. Because a large amount of grain boundaries existed in the coating, these grain boundaries could serve as short-cut diffusion path of metal ions. Al ions could diffuse outward very fast through grain boundaries and form a dense oxide film at the early oxidation stage. Under this condition, the ion diffusion coefficient in the alumina film decreased, which led to oxidation rate to decrease. Therefore, the oxidation kinetic obeyed quartic rate equation[15].
3.2 Phase composition of oxidation scale
Fig.2 shows XRD patterns of the Co-30Cr-6Al- 0.2Y coating isothermally oxidized for 5, 10, 20, 40, 90 and 180 min, respectively. Based on XRD analysis, even after shot term oxidation (t=5 min) sharp characteristic peaks of α-Al2O3 are observed, however, some very weak characteristic peaks of d-Al2O3 and g-Al2O3 are recognized after oxidation for 5, 10 and 20 min, which indicates that α-Al2O3 and trace amount of d-Al2O3 and g-Al2O3 are formed on the sample surface. After 40 min oxidation, characteristic peaks of θ-Al2O3 are observed. After 180 min oxidation, all characteristic peaks of metastable alumina disappear, which indicates that metastable alumina transforms to α-Al2O3 at this stage.
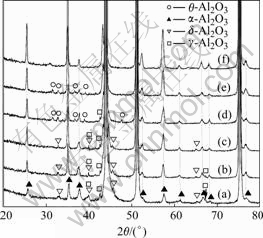
Fig.2 XRD patterns of Co-30Cr-6Al-0.2Y coating isothermally oxidized for various time: (a) 5 min; (b) 10 min; (c) 20 min; (d) 40 min; (e) 90 min; (f) 180 min
Depended upon the reaction temperature the alumina is formed in different modifications: below 900-950 ℃ the alumina is generally formed as g-Al2O3 and at higher temperatures as α-Al2O3. δ-Al2O3 is also identified in scales formed at 900 ℃. Metastable alumina transforms to α-Al2O3 on heating, and the transform follows the following order[16]:

Actually, in this experiment, when the as-prepared samples are oxidized at 1 050 ℃, some metastable alumina, for instance, g-, δ- and θ-Al2O3 are formed at the initial oxidation stage. With increasing oxidation time metastable alumina transformed according to the following order:
(γ+δ)-Al2O3→θ-Al2O3→α-Al2O3 (3)
XRD results show that no other oxides (chromium oxide, spinel, or cobalt oxide) are formed during the oxidation. This phenomenon might be related to the following two reasons: first, aluminum exhibits higher affinity to oxygen than chromium and cobalt at 1 050 ℃ [17]:
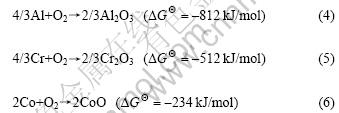
Therefore, the formation of Al2O3 is favored by thermodynamics. The second main reason is related to the microstructure of nanocrystalline CoCrAlY coating. After nanocrystallization, the grain boundary concentration increases, which increases the aluminium diffusivity and reduces the aluminium critical concentration necessary to form a unitary Al2O3 external or continuous inner scale and therefore, promotes the selective oxidation of aluminium[5].
3.3 Microstructures of oxidation production
Fig.3 shows surface morphologies of the as-prepared samples oxidized for 5, 10, 20, 40, 90 and 180 min, respectively. After 5 and 10 min oxidation, some large grain boundaries of the coating can be observed, although the oxide scales are formed on the sample surface. Some small oxide grains are detected on the coating surface, however, a lot of much larger oxide grains are observed at the coating grain boundaries. After 20 min oxidation, the larger oxide grains at the coating grain boundaries grow up preferentially, which results in appearance of the annular oxide products and encircled coating grains. With increasing oxidation time, some needle-like oxides (marked as N) are observed at the coating grain boundaries (see Fig.3(d)). When combining the results of XRD patterns and SEM observation, it can be concluded that these needle-like oxide grains are θ-Al2O3. Fig.3(d) shows that a lot of needle like θ-Al2O3 grains are formed at the coating grain boundaries, which is transformed from g-Al2O3 and d-Al2O3 grains. It is noteworthy that these needle like θ-Al2O3 grains are rich mainly on the coating grain boundaries, therefore, at the initial oxidation stage, the metastable alumina (g-Al2O3 and δ-Al2O3) prefer to grow at the coating grain boundaries, whereas, the stable alumina (α-Al2O3) is formed on the coating grains. After 90 min oxidation, part of needle like θ-Al2O3 grains are transformed to α-Al2O3. After 180 min oxidation, no needle like θ-Al2O3 grains are detected on the sample surface, because no characteristic peak of metastable alumina is observed at this stage, therefore it can reasonably be concluded that θ-Al2O3 grains are completely transformed to α-Al2O3.
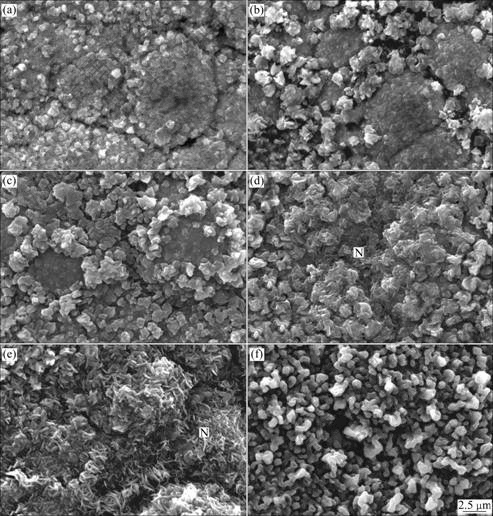
Fig.3 Surface morphologies of as-prepared samples oxidized for various time at 1 050 ℃: (a) 5 min; (b) 10 min; (c) 20 min; (d) 40 min; (e) 90 min; (f) 180 min
As mentioned above, the metastable alumina prefers growing at the grain boundaries of CoCrAlY coating. PINT[18] demonstrated that the formation of metastable or stable alumina was related to aluminum concentration in the substrate alloy or coating. When β-NiAl coating was oxidized at 950 ℃ the formation of stable alumina (α-Al2O3) was favored in aluminum depletion location, however, the metastable phase preferred growing in the Al enrichment region. Because nanocrystalline coating had a large amount of grain boundaries and acted as fast diffusion paths for metal ions, Al could diffuse outwardly through these grain boundaries, which led to higher Al concentration at the coating grain boundaries during oxidation[5]. Under this condition, the formation of metastable alumina was favored at the grain boundaries, whereas the stable alumina mainly formed beyond the coating grain boundaries.
It was reported that at the initial oxidation stage, fast growing of metastable alumina corresponded to the fast oxidation kinetics[8-9], which implied that the formation of metastable and stable alumina had different mechanisms. During oxidation α-Al2O3 grew mainly through oxygen inward diffusion[8,18], whereas metastable alumina (θ-Al2O3) grew through Al ion outward diffusion. In this experiment, protuberant oxide was formed at coating grain boundaries, judged from the topographies. It is intuitive to suppose that metastable alumina (g-Al2O3, δ-Al2O3 and θ-Al2O3) grains grew outward from the original coating surface, which agreed with the growth mechanism of θ-Al2O3 proposed by BRUMM et al[9] and PINT et al[18-19].
4 Conclusions
1) When nanocrystalline CoCrAlY coating is oxidized at 1050℃, the oxidation kinetics follows quartic rate equation at the initial oxidation stage.
2) At the early oxidation stage, metastable alumina (g-Al2O3 and δ-Al2O3) is formed on the sample surface. With increasing oxidation time, g-Al2O3 and δ-Al2O3 grains transform to θ-Al2O3, and totally transform to α-Al2O3 after 180 min oxidation.
3) The formation of metastable alumina grains is favored at the coating grain boundaries, which corresponds to Al-rich regions.
References
[1] FRITSCHER K. Eutectic structures in the Ni-Co-Cr-Al system obtained by plasma spraying and by Bridgman growth [J]. Journal of Crystal Growth, 2003, 250: 546-557.
[2] MOBARRA R, JAFARI A H , KARAMINEZHAD M. Hot corrosion behavior of MCrAlY coatings on IN738LC [J]. Surface and Coatings Technology, 2006, 216: 2202-2207.
[3] BAUFELD B, BARTSCH M, BRO? P, SCHMUCKER M. Microstructural changes as postmortem temperature indicator in Ni-Co-Cr-Al-Y oxidation protection coatings [J]. Mater Sci Eng A, 2004, A384: 162-171.
[4] GUO M H, WANG Q M, KE P L, GONG J, SUN C, HUANG R F, WEN L S. The preparation and hot corrosion resistance of gradient NiCoCrAlYSiB coatings [J]. Surface and Coatings Technology, 2006, 200: 3942-3949.
[5] WANG F, GENG S. High temperature oxidation and corrosion resistant nanocrystalline coatings [J]. Surface Engineering, 2003, 19(1): 32-36.
[6] NIJDAM T J, JEURGENS L P H, SLOOF W G. Promoting exclusive α-Al2O3 growth upon high-temperature oxidation of NiCrAl alloys: Experiment versus model predictions [J]. Acta Materialia, 2005, 53: 1643-1653.
[7] CHEN G, RUHLEC M. Transient alumina transformation on a sputtered K38G nanocrystalline coating [J]. Surface and Coatings Technology, 2005, 191: 263-266.
[8] GRABKE H J. Oxidation of NiAl and FeAl [J]. Intermetallics, 1999, 7: 1153-1158.
[9] BRUMM M W, BRABKE H J. The oxidation behaviour of NiAl—Ⅰphase transformations in the alumina scale during oxidation of NiAl and NiAl-Cr alloys [J]. Corr Sci, 1992, 33(11): 1677-1690.
[10] PENG X, CLARKE D R, WANG F. Transient alumina transformations during the oxidation of magnetron-sputtered CoCrAl nanocrystalline coatings [J]. Oxid Met, 2003, 60(3/4): 225-240.
[11] AN T F, GUAN H R, SUN X D, HU Z Q. Effect of the Al2O3 transformation in scales on the oxidation behavior of a nickel-base superalloy with an aluminide diffusion coating [J]. Oxid Met, 2000, 54(3/4): 301-316.
[12] RYBICKI G C, SMIALEK J L. Effect of the θ→α-Al2O3 transformation on the oxidation behavior of β-NiAl+Zr [J]. Oxid Met, 1989, 31(3/4): 275-304.
[13] WANG F, LOU H. Oxidation behaviour and scale morphology of normal-grained CoCrAl alloy and its sputtered microcrystalline coating [J]. Mater Sci Eng A, 1990, A129(2): 279-285.
[14] KOFSTAD P. High Temperature Corrosion [M]. London and New York: Elsevier Applied Science Publishers Ltd, 1988.
[15] LI T F. High Temperature Oxidation and Hot Corrosion of Metals [M]. Beijing: Chemical Industry Press, 2003. (in Chinese)
[16] ZHANG Z H, LI N. Effect of polymorphism of Al2O3 on the synthesis of magnesium aluminate [J]. Ceram Inter, 2005, 31: 583- 589.
[17] TURKDOGAN E T. Physical Chemistry of High Temperature Technology [M]. New York: Academic Press, 1980.
[18] PINT B A. Experimental observations in support of the dynamic-segregation theory to explain the reactive-element effect [J]. Oxid Met, 1996, 45(1/2): 1-37.
[19] PINT B A, MARTIN J R, HOBBS L W. The oxidation mechanism of θ-Al2O3 scales [J]. Solid State Ionics, 1995, 78: 99-107.
Foundation item: Project(50371095) supported by the National Natural Science Foundation of China
Corresponding author: LIU Guang-ming; Tel:+86-791-3863026; E-mail: tisic@126.com
(Edited by CHEN Wei-ping)