Trans. Nonferrous Met. Soc. China 23(2013) 1033-1038
Preparation and electrochemical performance of nano-scale Ni(OH)2 doped with zinc
Li ZHAO, Zhi-hao LIU, Lei JIN
School of Chemical Engineering and Technology, Harbin Institute of Technology, Harbin 150001, China
Received 20 February 2012; accepted 11 June 2012
Abstract: Nano-scale Ni(OH)2 doped with Zn was prepared by precipitation transformation method and characterized by XRD and TEM. The electrochemical performance was investigated by cyclic voltammetry (CV) and constant current technology. The measurement results indicate that the lattice parameters of nano-scale Ni(OH)2 are changed and the agglomeration of particles becomes obvious with the increased Zn-doped content. Compared with un-doped one, the discharge specific capacities of nano-scale Ni(OH)2 doped with 10% Zn are enhanced by 8% and 6%, respectively, at the discharge rate of 0.2C and 3C. After 110 cycles, the discharge specific capacity of the sample doped with 10% zinc is still above 85% of its initial capacity discharged at 0.2C. Therefore, a suitable Zn-doped content is beneficial to improving the discharge performance of nano-scale Ni(OH)2.
Key words: nano-materials; nickel hydroxide; Zn doping; electrochemical performance
1 Introduction
The increasing concerns over air pollution and depletion of natural petroleum reserves have spurred renewed interest in electric vehicles (EV), where high power batteries play an important role. Due to the high power and low cost, Ni-MH batteries are considered to be one of the most promising choices for EV application [1].
As the positive material of MH/Ni battery, Ni(OH)2 is a kind of p-type semiconductor, and its properties are one of the most important factors to influence the performance of battery. Doping such metal cations as Co and Al is an effective way to improve the electrochemical performance of Ni(OH)2. Nano-scale Ni(OH)2 is a latent positive material for MH/Ni batteries with superior electrochemical performance [2,3]. It has been reported that nickel hydroxide with a smaller crystalline size shows better charge–discharge cyclic characteristics and a higher proton diffusion coefficient, and the capacity of Ni(OH)2 electrode can be increased by mixing nano-phase Ni(OH)2 with spherical Ni(OH)2 [4]. In order to further enhance the electrochemical performance of nano-scale Ni(OH)2, many elements have been doped into nano-scale Ni(OH)2. Among them, cobalt doping significantly improves high-current discharge capacity and cycle performance of Ni(OH)2 [5,6]; Cu-doped amorphous nano-Ni(OH)2 power material has higher charge efficiency and better discharge performance [7,8]; nano-scale Ni(OH)2 doped with Al exhibits a better electrochemical cycle performance [9]; Mn-doped Ni(OH)2 shows higher oxygen evolution overpotential and proton diffusion rate [10]; Mg doping and Y doping enhance the high rate discharge capacity and cyclic performance of nano-scale Ni(OH)2 [11-13].
As reported, doping Zn [14,15] into micron-scale Ni(OH)2 can improve the reversibility and oxygen evolution overpotential of Ni(OH)2 electrode and inhibit the expansion of Ni(OH)2 electrode, but the effects of doping Zn on the performance of nano-scale Ni(OH)2 have not been well investigated so far. The effects of doping Zn on the crystalline parameters, surface morphology, and proton diffusion coefficient of nano-scale Ni(OH)2 have not be reported yet. Therefore, In this work, Zn-doped nano-scale Ni(OH)2 was prepared by solution method, and the effects of doping amount of Zn on the crystal structure, surface morphology and electrochemical performance of nano-scale Ni(OH)2 were investigated.
2 Experimental
2.1 Preparation of Zn-doped nano-scale Ni(OH)2
0.2 mol/L Ni(NO3)2 and 0.2 mol/L Zn(NO3)2 were dropped into 0.1 mol/L Na2C2O4 solution at the same speed and stirred to generate precipitate of oxalate. After 10 min, tween-80 was added into the reactor slowly. After stirring for 1 h, the obtained mixed-solution was pumped into base NaOH solution (50 °C, pH=11.0), and at the same time, 0.2 mol/L NaOH was also dropped into the base NaOH solution at a suitable rate to keep pH unchanged. The reaction system was stirred for 5 h, so that the precipitation reaction was fully completed. Precipitate was separated by centrifuge and washed with distilled water, and then was oven-dried at 70 °C for 12 h. After being pulverized with an agate mortar, nano-scale Ni(OH)2 doped with Zn was obtained. In this experiment, the mass fraction of doped Zn was controlled at 0, 5%, 10%, and 15%.
2.2 Characterization of Zn-doped nano-scale Ni(OH)2
TEM observation was performed at a voltage of 20 kV using a TEM-1200EX scanning electron microscope made by JEOL. A D/MAX-rB diffractometer which was produced by Rigaku Corporation was used to measure the XRD spectra of the samples (voltage 45 kV, Cu Kα, scan rate 5 (°)/min).
2.3 Electrochemical measurement of Zn-doped nano- scale Ni(OH)2
Cyclic voltammetric measurement was performed by a CHI630A electrochemical workstation. A powder microelectrode was used as working electrode in a three-electrode system, a Hg/HgO electrode was used as reference electrode and a hydrogen-storage alloy electrode was used as counter electrode. The electrolyte was 7 mol/L KOH solution with 15 g/L LiOH. The scanning range was 0-0.70 V and scanning rate was 1-9 mV/s.
Discharge performance was conducted by test cells. Zn-doped nano-scale Ni(OH)2 was mixed with 5% (mass fraction) cobalt, 5% (mass fraction) nickel and 80% (mass fraction) sphere nickel hydroxide powders, and then appropriate mount binder (m(CMC):m(PTFE)= 1.5%:5%) was dropped into the mixed powder to obtain a paste. The paste was filled into foam nickel substrate with dimensions of 1 cm×1 cm, then was dried at 80 °C for 2 h. The dried electrode was pressed to obtain a nickel hydroxide electrode. A single Ni(OH)2 electrode was sandwiched between two hydrogen-storage alloy electrodes and then were immersed in the electrolyte consisting of 7 mol/L KOH and 15 g/L LiOH. The test cells were conducted with BTS series battery performance-testing instrument produced by Neware Company.
3 Results and discussion
3.1 XRD analysis of Zn-doped nano-scale Ni(OH)2
Figure 1 shows the XRD patterns of nano-scale samples doped with different amounts of Zn. It can be seen from Fig. 1 that all characteristic diffraction peaks are well consistent with the hexagonal β-Ni(OH)2 (2θ= 19°, 33°, 38°). This result indicates that all samples are typically β-Ni(OH)2. The low and broad peaks at (001) and (102) lattice plane indicate the refinement of crystal grains.
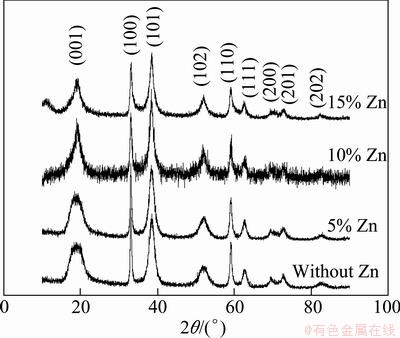
Fig. 1 XRD patterns of nano-scale Ni(OH)2 doped with different amounts of Zn
With the increased zinc-doped content, the crystallographic ability of material becomes bad. Especially for the sample doped with 10% Zn, many small peaks on XRD patterns indicate that there are abundant defects in the sample, which is helpful in improving the electrochemical performance of Ni(OH)2.
Table 1 shows the lattice parameters of nano-scale Ni(OH)2 doped with different amounts of zinc, which are calculated according to Scherrer Formula [16]. It can be seen from Table 1 that Zn-doping exerts only a slight influence on parameter a and parameter b, but parameter c is changed obviously. With the increased Zn-doped amount, parameter c increases firstly, and then decreases. When the doped amount of Zn is 5%, parameter c achieves its maximum value. The changing trends of parameter a and parameter b are the same as parameter c, but parameter a and parameter b reach their maximum at the point of 10% Zn doping. As we known, Ni(OH)2 is a kind of stratified structure material, and the increase of parameter c means the increase of interlayer spacing and the decrease of proton diffusion in the reacting processes of Ni(OH)2, which is helpful to improving the electrochemical performance of Ni(OH)2.
Table 1 Effect of Zn-doped content on lattice parameters of samples
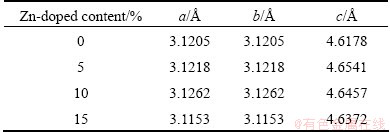
3.2 Morphology analysis of Zn-doped nano-scale Ni(OH)2
The TEM images of nano-scale Ni(OH)2 doped with different contents of Zn are given in Fig. 2. It can be seen from Fig. 2 that the un-doped sample consists of many small thin slices and a spot of acicular crystals, and the particles are in a well-distributed state. With the increase of Zn-doped content, the agglomeration of particles becomes significantly serious. Doping zinc into the lattices of nano-scale Ni(OH)2 not only affects the lattice parameters, but also changes ζ potential and thereby changes the interaction force between particles [17]. According to the results of TEM measurements, it can be concluded that the repulsive interaction of particles is reduced with the increased zinc doping content. Due to high surface free energy, nano-materials are unstable and easy to agglomerate. The decrease of ζ potential further leads to agglomeration of particles. The suitable agglomeration of nano-scale Ni(OH)2 can enhance its taping-density and improve its volume specific capacity, but too serious agglomeration is harmful to high rate discharge performance of nano-scale Ni(OH)2.
3.3 Cyclic voltammetry behaviour of samples
Figure 3 shows the cyclic voltammetry curves of nano-scale Ni(OH)2 doped with different contents of Zn. It is obvious that the oxidation peaks of all samples appear at the potential about 0.5 V, so it can be concluded that doping zinc does not affect the oxidation potential of nano-scale Ni(OH)2 evidently. With the increased Zn-doping amount, the potential of reduction peak increases firstly and then decreases. When the Zn-doped contents are 5% and 10%, the reduction peak potential approximates to 0.4 V which is higher than others. The higher reduction peak potential is desirable for Ni(OH)2 because it will heighten the discharge plateau of Ni(OH)2 electrode and improve the discharge performance of batteries.
For a nano-scale Ni(OH)2 micro-electrode system, peak current and scanning rate fit to the following formula:
(1)
where IP is the peak current; n is the number of transfer electrons; A is the area of electrode; D is the proton diffusion coefficient; v is the scanning rate; C0 is the initial concentration of Ni(OH)2.
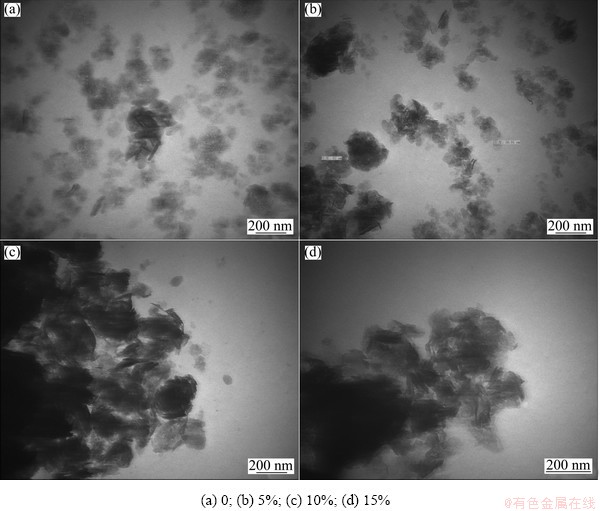
Fig. 2 TEM images of nano-scale Ni(OH)2 doped with different contents of Zn
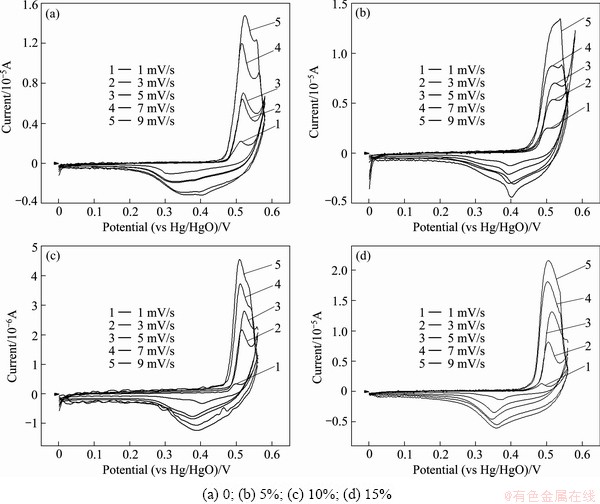
Fig. 3 CV curves of samples doped with different contents of Zn
The oxidation peak current IP and the square root of scanning rate v1/2 are used to fit a line, and then the slope of line and the real superficial area A of the powder microelectrode are taken into Formula (1) to calculate proton diffusion coefficient D of nano-scale Ni(OH)2.
As shown in Table 2, the proton diffusion coefficient (D) of nano-scale Ni(OH)2 firstly increases and then decreases with the increased Zn-doped contents. The maximum D is obtained when the doping content of Zn is 10%. The change trend of D is similar to that of parameter c shown in Table 1, which proves that raising layer distance is beneficial to increasing D value. It can also be seen from Table 2 that the proton diffusion coefficient of nano-scale Ni(OH)2 doped with Zn is bigger than that of the un-doped one. Certainly, the proton diffusion coefficient is not the unique determinant factor to the electrochemical performance of Ni(OH)2. In some cases, the charge transfer step could also affect the electrochemical performance, but the proton diffusion coefficient exerts a greater effect on the electrochemical performance of Ni(OH)2.
Table 2 Proton diffusion coefficient of samples doped with different contents of Zn

3.4 Discharge performance of samples
Figure 4 shows the discharge curves of nano-scale Ni(OH)2 doped with different contents of Zn. As described in Fig. 4, the discharge specific capacity of samples increases gradually at first and then decreases with the increased Zn-doping contents. In the case of 0.2C discharging, the discharge specific capacity of nano-scale Ni(OH)2 doped with 10% Zn is about 285 mA·h/g, which is higher than that (about 264 mA·h/g) of the un-doped nano-scale Ni(OH)2 by 8%, and its discharge plateau is also the highest. When being discharged at 3C, the discharge specific capacity of the sample doped with 10% Zn is 6% higher than that of the un-doped one. And the sample doped with 15% Zn also shows a higher discharge specific capacity, but its discharge plateau is lower than that of other samples when discharge rate is 3C. So, it can be concluded that 10% is a suitable Zn-doped content, which coincides with the result of cyclic voltammetry measurement. That is to say, doping 10% zinc in nano-scale Ni(OH)2 can preferably change the lattice parameters, increase proton diffusion coefficient, and improve discharge performance of nano-scale Ni(OH)2.
The effect of Zn-doped nano-scale Ni(OH)2 on the discharge performance of nickel hydroxide electrode can be explained as follows. Usually, common micrometer nickel hydroxide is spherical with an average size of 10-15 μm, and void volume of granules can still reach above 0.5 μm after being compacted. Nano-scale Ni(OH)2 has at least one physical dimension less than 100 nm in length, so when nano-scale Ni(OH)2 is added into common micro-sized spherical Ni(OH)2, it can fully fill in the interspace of common micro-sized Ni(OH)2 particles, which facilitates enough contact, thus shortens the route of proton diffusion among common micro-sized Ni(OH)2 particles [3]. On the other hand, the proton diffusion coefficient of nano-sized Ni(OH)2 is larger than that of micro-sized Ni(OH)2 (see Table 2). Both aspects accelerate the utilization of active materials in nickel hydroxide electrode.
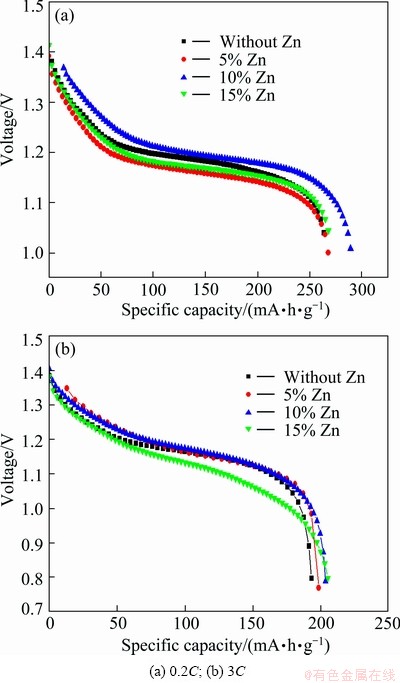
Fig. 4 Discharge curves of samples doped with different amount of Zn
3.5 Cyclic performance of samples
Figure 5 shows cyclic performance of nano-scale Ni(OH)2 doped with 10% zinc, in which cycles about 110 times are discharged at 0.2C. It can be seen from Fig. 5 that the rest capacity of test cell decreases gradually in the first 60 cycles, and then stabilizes at 85% of its initial capacity. The reduction of cyclic capacity at initial stage is related not only to common factors (e. g. the falling of active materials), but also to the agglomeration of nano-scale particles and Zn dissolving. Nano-scale particles are unstable and easy to agglomerate in charge/discharge processes, which would reduce the specific surface of nano-scale Ni(OH)2 and increase the polarization of reaction. Meanwhile, a small part of doped zinc may dissolve in electrolyte to make the capacity of test cell decrease.
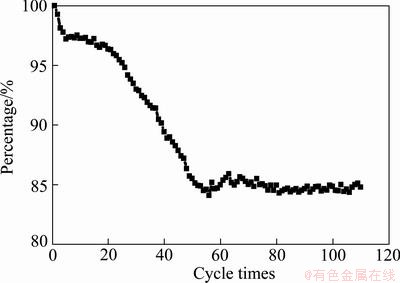
Fig. 5 Cycle performance of nano-scale Ni(OH)2 doped with 10% Zn discharged at 0.2C
4 Conclusions
1) Doping zinc can change the lattice structure of nano-scale Ni(OH)2.
2) Doping zinc can also change the peak potential and proton diffusion coefficient of nano-scale Ni(OH)2. Appropriate Zn doping amount can enhance the proton diffusion coefficient.
3) The discharge performance of Zn-doped nano- scale Ni(OH)2 is improved, especially the sample with 10% zinc, whose discharge specific capacity and discharge voltage are higher than those of other samples.
References
[1] HE X X, PU W H, CHENG H W, JIANG C Y, WANG C R. Granulation of nano-scale Ni(OH)2 cathode materials for high power Ni-MH batteries [J]. Energy Conversion and Management, 2006, 47: 1879-1883.
[2] HU W K, GAO X P. Evaluation of nano-crystal sized α-nickel hydroxide as an electrode material for alkaline rechargeable cells [J]. J Power Sources, 2006, 160: 704-710.
[3] GUAN X Y, DENG J C. Preparation and electrochemical performance of nano-scale nickel hydroxide with different shapes [J]. Materials Letters, 2007, 61: 621-625.
[4] ZHOU H B, ZHOU Z T. Preparation, structure and electrochemical performances of nanosized cathode active material Ni(OH)2 [J]. Solid State Ionics, 2005, 176: 1909-1914.
[5] WANG Hui-jing, LIU Chang-jiu, LIU Ai-fang, SONG Sha, YUAN Gui-fa, GU De-long. Preparation and electrochemical performance of doped Co amorphous Ni(OH)2 [J]. Journal of Guilin University of Technology, 2007, 27(2): 262-265. (in Chinese)
[6] SUN Hai-feng, JIANG Wen-quan, YU Li-min, FU Zhong-zhen, GUO Rong-gui, LI Tao, YANG Hui. Preparation and electrochemical properties of petal-shaped nickel hydroxide with cobalt doping [J]. Materials Review, 2011, 25(4): 49-52. (in Chinese)
[7] LIU Chang-jiu, WANG Hui-jing, SUN Dan, LU Li-jun. Synthesis and preparation of Cu-doped amorphous nano-Ni (OH)2 [J]. Acta Scientiarum Naturalium Universitatis Sunyatseni, 2007, 46(Appl): 283-284.
[8] YU Dan-mei, CHEN Chang-guo, ZHOU Shang-qi, YANG Yu-qiong. Modification study of nano-Ni(OH)2 doped copper [J]. Battery Bimonthly, 2005, 35(2), 102-104. (in Chinese)
[9] ZHOU Huan-bo, ZHOU Zhen-tao. Structure and electric properties of Al-doped nano-sized Ni(OH)2 [J]. Battery Bimonthly, 2005, 35(3): 181-183. (in Chinese)
[10] WU Mei-yin, WANG Jian-ming, ZHANG Jian-qing, CAO Chu-nan. Structure and electrochemical performance of Mn-substituted nickel hydroxide [J]. Acta Physico-Chimica Sinica, 2005, 21(5): 523-527.
[11] WANG Xin, FU Xian-zhu, LIANG Ying, LIAO Dai-wei. Structure and electrochemical performance of Mg-doped nickel hydroxide [J]. Chinese Journal of Power Source, 2007, 31(9): 732-735. (in Chinese)
[12] LIU Chang-jiu, SUN Dan, GU De-long, WU Hua-bin. Structure and electro-chemical synthesis of Y-doped amorphous nano-Ni(OH)2 [J]. Rare Metal Materials and Engineering, 2009, 38(9): 1556-1561. (in Chinese)
[13] REN Jun-xia, ZHOU Zhen, YAN Jie. Effects of yttrium-doping on high-temperature electrochemical performances of nickel hydroxide electrodes [J]. Acta Phys Chim Sin, 2007, 23(5): 738-742. (in Chinese)
[14] PROVAZI K, GIZ J, ANTONIA L H, DE T S. The effect of Cd, Co, and Zn as additives on nickel hydroxide opto-electro-chemical behavior [J]. J Power Sources, 2001, 10(2): 224-232.
[15] YU Dan-mei, ZHOU Shang-qi, CHEN Chang-guo, WEN Li, WANG Hua-qing. Effect of the amount of zinc-doped on the electrochemical performance nickel hydroxide [J]. Journal of Chongqing University, 2004, 3(1): 74-77.
[16] LI Shu-tang. X-ray diffractometry fundament of crystals [M]. Beijing: Metallurgic Industry Press, 1990: 169-175. (in Chinese)
[17] MACARTHUR D M. The hydrated nickel hydroxide electrode potential sweep experiments [J]. J Electrochem Soc, 1970, 117(4): 422-426.
锌掺杂纳米Ni(OH)2的制备及其电化学性能
赵 力,刘志豪,金 雷
哈尔滨工业大学 化工学院,哈尔滨 150001
摘 要:采用沉淀转化法制备出掺杂Zn的纳米Ni(OH)2。利用XRD和TEM对材料的结构和微观形貌进行分析,利用循环伏安技术和恒流充放电技术对材料的电化学性能进行研究。结果表明,随着Zn掺杂量的增加,纳米Ni(OH)2晶格参数发生相应的变化,同时,材料的团聚逐渐加重。在0.2C和3C放电倍率下,掺杂10% Zn的纳米Ni(OH)2放电比容量比未掺杂的样品分别提高8%和6%。经110次循环后,掺杂10%Zn的样品放电比容量仍可达到其初始比容量的85%。因此,掺杂适量的Zn可以提高纳米Ni(OH)2的放电性能。
关键词:纳米材料;氢氧化镍;锌掺杂;电化学性能
(Edited by Hua YANG)
Foundation item: Project (BK2008591) supported by the Natural Science Foundation of Jiangsu Province, China; Project (2009RFQXG065) supported by Harbin Special Foundation of Technological Innovation Talent, China
Corresponding author: Li ZHAO; Tel: +86-451-86413721; E-mail: dhx907@hit.edu.cn
DOI: 10.1016/S1003-6326(13)62563-7