
Microstructure and mechanical properties of AZ81 magnesium alloy with Y and Nd elements
XIE Jian-chang(谢建昌), LI Quan-an(李全安), WANG Xiao-qiang(王小强), LI Jian-hong(李建弘)
School of Materials Science and Engineering, Henan University of Science and Technology,
Luoyang 471003, China
Received 11 May 2007; accepted 20 September 2007
Abstract: The effects of rare earth(RE) elements Y and Nd (w(Y)/w(Nd)=3?2) with total content of 1%-4% on microstructures and elevated temperature mechanical properties of AZ81 magnesium alloy were investigated. The results show that, proper content of rare earth elements makes the microstructures of AZ81 magnesium alloy refine obviously and the quantity of β-Mg17Al12 phases reduce, and Al2Y and Al2Nd form. After solid solution treatment, with increasing content of rare earth elements, the tensile strength and elongation of the alloys (at room temperature, 150 ℃ and 250 ℃) increase first, then decrease. When the content of rare earth elements is up to 2%, the values of tensile strength at room temperature and 150 ℃ are up to their maxima simultaneously, 282 MPa and 212 MPa, respectively. Meanwhile, the values of elongation at room temperature and at elevated temperature are also up to their maxima, 13% and 15%, respectively.
Key words: AZ81 magnesium alloy; rare earth; yttrium; neodymium; microstructure; mechanical properties
1 Introduction
The magnesium alloy is the lightest constructional material, which has lower density, higher specific strength, higher specific stiffness, better cast characteristics, better electric conductivity, better thermal conductivity than steel and aluminum alloys. So it is widely used in automobile industry, electron industry and 3C product. However, its lower strength and creep strength at elevated temperature cause the poor heat resistance of magnesium alloy, which is the main factor of limiting its application at elevated temperature[1-3].
The properties of AZ81 alloy are very similar to AZ91D alloy[4-5]. Rare earth elements can effectively improve the heat resistance of magnesium alloy, because they can form the high-melting point compounds with other elements and refine the grain. The effect of RE elements Y and Nd on microstructures and mechanical properties of AZ81 alloy was studied in this work.
2 Experimental
AZ81(A) alloy, metallic aluminum (99.98%), metallic zinc (99.97%), Mg-Y master alloy and Mg-Nd master alloy were used as raw materials. The studied alloy was designed as Mg-8.0Al-0.75Zn-0.15Mn-xRE, where x is the total content of rare-earth elements, x=0, 1, 2, 3, 4 respectively. The mass ratio of Y to Nd is 3?2. The designed compositions of the alloys are listed in Table 1.
Table 1 Designed compositions of alloys (mass fraction, %)

All the raw materials should be dried at 200 ℃ before the melting started. The alloys were melted in a vacuum induction furnace by graphite (99.9%) crucible under protection of argon atmosphere. And the alloys were held for 15 min at 720 ℃, then were poured into a metallic mold (d 18 mm×200 mm) at 690-700 ℃. At last, the as-cast bars of alloys covered with MgO powders were heated for solid solution treatment at 420 ℃ for 10 h and aging treatment at 250 ℃ for 8 h.
The tensile tests were carried out at a strain rate of 1 mm/min in a SHIMADZU AG-I 250 kN precision universal material test machine at room temperature (written as 20 ℃), 150 ℃ and 250 ℃, respectively. Three bars were tested for each point. To the elevated temperature tensile tests, the alloys were held for 15 min at their corresponding temperatures. Microstructures, fracture surface morphologies and compositions of the alloys were analyzed by optical microscopy and scanning electron microscopy (JSM-5610LV) with energy dispersive spectroscopy. Phase analyses were performed with a X’pert X-ray diffractometer.
3 Results and analysis
3.1 Effect of RE elements Y and Nd on micro- structure of AZ81 magnesium alloy
Based on the phase diagrams of Mg-Al binary system and Mg-Al-Zn ternary system, the microstructures of as-cast alloys consist of α-Mg solid solution, network eutectic structure (α+β-Mg17Al12) and precipitated phase inside the grains[6-7].
The microstructures of the alloys after solid solution and aging treatment are shown in Fig.1. As can be seen in Fig.1(a), β-Mg17Al12 phases of AZ81 magnesium alloy precipitate again both inside grains and on grain boundaries after solid solution and aging treatment, which are scattered on the grain boundaries in coarse bulk and inconsecutive network, while inside the grains are in fine particle structure. From the phase diagram of Mg-Al binary system, it can be known that when AZ81 magnesium alloys are heated for solid solution treatment at 420 ℃, the supersaturated α-Mg solid solutions of the alloys first form, then the solid solutions directly form incoherent equilibrium phase with the course of aging treatment, while no other pre-precipitates and transition phases form[8-10]. The microstructures of the alloys with RE elements (Y+Nd) are shown in Figs.1(b)-(e). The size of bulk eutectic structure of the alloys becomes small and the quantity decreases gradually, and particulate phase increases inside the grains. RE elements and aluminum form a kind of high-melting point compound (Al-RE), which causes the reduction of the content of aluminum in solid solution, meanwhile, the electronegative differences between Y, Nd and Mg, Al decide that Y and Nd are more easier to form compound with Al than Mg, so compound (Al-Y, Al-Nd) is easier to form after solid solution treatment, while the quantities of β-Mg17Al12 phase reduce obviously in Figs.1(c) and (d). Especially when the content of RE elements is up to 4%, bulk β-Mg17Al12 phases along grain boundary almost disappear in Fig.1(e). When the content of RE elements is lower, the grains of AZ81 are refined and the microstructures are homogeneous in Fig.1(b). With increasing the content of RE elements, the quantity of precipitated phase also increases, its grain is coarsened and its distribution shows a tendency of non-equilibrious segregation in Figs.1(c) and (d).
Fig.1 Microstructures of AZ81+RE alloys after solid solution and aging treatment: (a) AZ81; (b) AZ81+1%RE; (c) AZ81+2%RE; (d) AZ81+ 3%RE; (e) AZ81+4%RE
XRD patterns shown in Fig.2 assume that when the content of RE elements is up to 1%, the microstructures of the alloys mainly consist of α-Mg base, β-Mg17Al12 and Al2Y phase inside the grains in Fig.2(a). When the content of RE elements is up to 4%, the microstructures of the alloys mainly consist of α-Mg base, secondary phase (Al2Y) in grain, while there is not β-Mg17Al12 phase inside the grains in Fig.2(b), which is consistent with Fig.1. SEM image and EDS result of the microstructures of the alloys after solid solution and aging treatment shown in Fig.3 indicate that when the content of RE elements is up to 1%, the particulate phases are much fine, which are distributed homogeneously with globular shape in the matrix. The compositions of the alloy are 23.17% Mg, 52.63% Al, 18.55% Y, 5.65% Nd (molar fraction) by EDS analysis, and the molar ratio of Al to RE is 2?1 when the α-Mg solid solution is excluded, so the particulate phases of the microstructure are judged as Al2Y and Al2 Nd, and Y, Nd contents are little in α-Mg as shown in Fig.3(a). When the content of RE elements is up to 4%, the quantity of particulate phase of microstructure increases, its grain becomes coarse and its distribution shows a tendency of non-equilibrious segregation. The compositions of the alloy are 9.36% Mg, 60.72% Al, 21.80% Y, 8.12% Nd (molar fraction) by EDS analysis, and the particulate phases of microstructure are also judged as Al2Y and Al2 Nd, but the content of Al2Y is much more than Al2 Nd in Fig.3(b). With increasing the content of RE elements, the quantity of bulk Al-RE phase is increased, the size is coarsened, and non-equilibrious segregation appears, which causes heterogeneousness of the microstructure and composition of alloys, induces stress concentration, and deteriorates the mechanical properties of the alloys. Meanwhile, this may also cause the increase of viscosity, the decline of fluidness and the increase of casting defects[11-14].
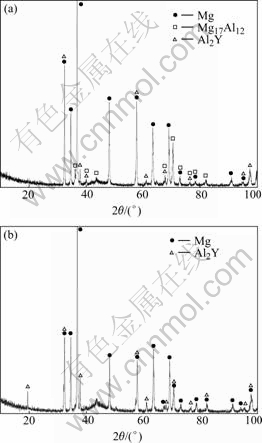
Fig.2 XRD patterns of AZ81+RE alloy: (a) AZ81+1%RE; (b) AZ81+4%RE
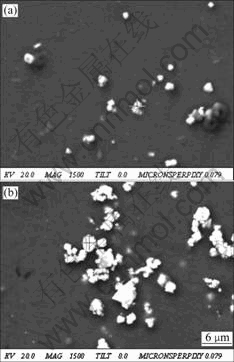
Fig.3 SEM images of AZ81+RE alloys after solid solution and aging treatment: (a) AZ81+1%RE; (b) AZ81+3%RE
LUO et al[15] studied the way of enhancing aging hardening treatment on the AZ91. The result assumed that the β-Mg17Al12 phase appeared in three different kinds of shapes, crystallography characteristics and interface structures in the microstructure of AZ91 alloys after aging treatment. The first kind of precipitated phase was coarse, and its quantity was more, which occupied 90% of the whole precipitated phases. However, it could not hinder dislocation motion because of its shape and crystallography characteristics, so it was bad for aging strengthening. The second and third kinds of precipitated phases were good for aging strengthening. They could hinder dislocation because of their shapes and crystallography characteristics, and the precipitated phases were vertical to or intersected with base plane. So one of available ways to enhance the effect of aging hardening of the alloy is to limit the first precipitated phases and increase the density of the second and third precipitated phases through alloying and heat treatment. The mechanical properties and compositions of AZ81 are very similar to AZ91D, and their strengthening mechanism should be the same.
3.2 Effect of RE elements Y and Nd on mechanical properties of AZ81 alloy
After solid solution and aging treatment, the tensile properties of the alloys are shown in Figs.4 and 5. As shown in Fig.4, with increasing the content of RE elements, the tensile strengths of the alloys at room temperature and 150 ℃ both first increase, then decrease. When the content of RE elements is 2%, the values of tensile strength at room temperature and 150 ℃ are up to their maxima simultaneously, 282 MPa and 212 MPa, respectively. Compared with AZ81 without RE elements, the strengths of the alloys are enhanced by 39% and 35%, respectively. When the content of RE elements is over 2%, the strengths of alloys drop sharply, which is consistent with the result that phase transformation appears. From above analysis, it can be known that proper content of RE elements (1.2%Y, 0.8%Nd) can enhance the strength of alloy at room temperature and at elevated temperatures availably. The strength of the alloys is first up then down with increasing the content of RE elements at 250 ℃, but the effect is less. Relationship between elongation and RE content in AZ81 alloys shown in Fig.5 assumes that the elongation of the alloys at room temperature and at elevated temperatures also increases first, then decreases with further increasing the content of RE elements. When the content of RE elements is 2% (1.2%Y, 0.8%Nd), the elongation at room temperature and 150 ℃ is up to the maxima simultaneously, 13% and 15%, respectively. When the content of RE elements is over 2%, the elongation of the alloys descends quickly. From above analysis, it can be known that the RE elements (1.2%Y, 0.8%Nd) can not only enhance the strength of the alloy at room temperature and at elevated temperatures, but also improve the plasticity.
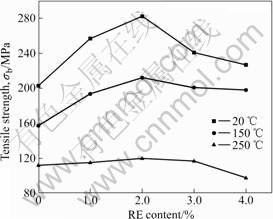
Fig.4 Relationship between σb and RE content in AZ81 alloys
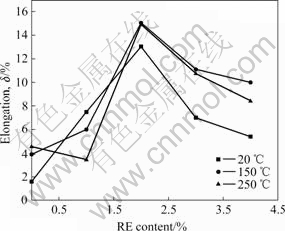
Fig.5 Relationship between δ and RE content in AZ81 alloys
For AZ81 alloys, β-Mg17Al12 phase is the main strengthening phase at room temperature in AZ81 alloys, but the thermal stability of β-Mg17Al12 phase whose melting point only is 437 ℃ is poor, which is easily intenerated and has poor strength at elevated temperatures, so it worsens the mechanical properties of AZ81 alloy at elevated temperatures. RE elements Y and Nd have biggish solid solubility in magnesium alloy. Not only the solution strengthening but also aging precipitated strengthening can be used, then the elevated properties of the alloys are enhanced. In this work, the main reasons of enhancing the elevated temperature properties of AZ81 alloy with Y, Nd are the function of solution strengthening, the dispersion hardening of (Al2Y, Al2Nd) and the reduction of β-Mg17Al12 phase because of the combination of Y, Nd and Al. The Al-RE phase of primary dispersion distribution has higher thermal stability than β-Mg17Al12 phase at grain boundaries in AZ81 alloy, which can pin the near grain motion at elevated temperatures and hinder effectively the motion of grain boundaries and dislocation. The effect of RE elements on heat resistant properties of the alloys also shows that RE elements make the permeability between phase boundary and grain boundary minimize, make the agglomeration of phase boundary slow, limit the movement of dislocation in whole course, make the defect of metal-oxide reduce in surface and change the crystalline lattice parameters[16], then they make the alloys have good anti-oxidation activity.
When the content of RE elements is over excessive, a lot of unsymmetrical distribution conglomerations (Al-RE) appear in the microstructure of the alloys, which makes the distribution of particle phase (Al-RE) non-uniform and the effect of dispersion strengthening weak, causes unsymmetry of composition and microstructure of the alloys, arouses stress concentration, and leads to the decline of the elevated temperature mechanical properties of the alloys.
3.3 Fracture behavior observation
SEM fractographs are shown in Fig.6. The photographs of AZ81 without RE elements shown in Fig.6(a) indicate brittle fracture, in which quasi-cleavage is the main characteristic, with obvious quasi-cleavage steps and tearing ridges. When the content of RE elements is 1%, fractures of the alloys show tearing ridges clearly, a few circular dimples appear locally, and the characteristics of quasi-cleavage and ductile fracture form as shown in Fig.6(b). When the content of RE elements is up to 2%, the photographs of the alloy are typical ductile fracture (Fig.6(c)) with a large number of dimples and tearing ridges of connected dimples, and obvious necking phenomenon in tensile test. The corresponding elongation is 13%. When the content of RE elements is 3%, the fracture of the alloys is brittle one, and corresponding elongation is low as shown in Fig.6(d). From the results of above analysis, the plasticity of the alloys can be enhanced obviously when the content of RE elements is 2% (1.2%Y, 0.8%Nd).
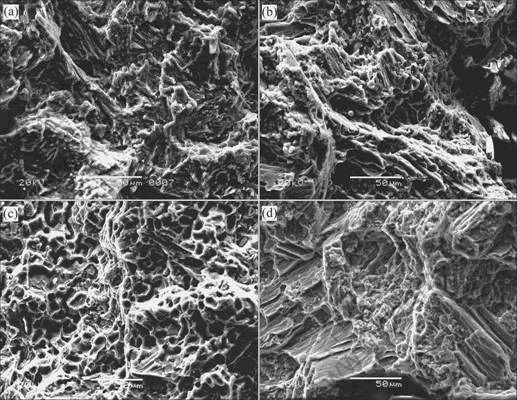
Fig.6 SEM fractographs for AZ81+RE alloys at room temperature: (a) AZ81; (b) AZ81+1%RE; (c) AZ81+2%RE; (d) AZ81+3%RE
4 Conclusions
1) With increasing the content of RE elements (Y+Nd) from 1% to 2%, the microstructure of AZ81 is obviously refined and the quantity of β-Mg17Al12 phase is reduced. Meanwhile, elevated temperature phases of Al2Y and Al2Nd and the solution strengthening of Y and Nd can improve the mechanical properties of the alloy at room temperature and elevated temperatures.
2) After solid solution and aging treatment, with increasing the content of RE elements, the tensile strength and elongation of the alloys are both (at room temperature, 150 ℃ and 250 ℃) increase first, then decrease basically.
3) When the content of RE elements is 2%, the values of tensile strength at room temperature and 150 ℃ are up to their maxima simultaneously, 282 MPa and 212 MPa, respectively. The elongation of AZ81 alloy can also be enhanced. When the content of RE elements is 2%(1.2%Y, 0.8%Nd), the values of elongation at room temperature and 150 ℃ are up to their maxima simultaneously, 13% and 15%, respectively.
References
[1] M?THIS K, GUBICZA J, NAM N H. Microstructure and mechanical behavior of AZ91 Mg alloy processed by equal channel angular pressing [J]. Journal of Alloys and Compounds, 2005, 394(1):194-199.
[2] WANG Xiao-feng, ZHAO Jiu-zhou. Hot rolling characteristics of spray-formed AZ91 magnesium alloy [J]. Trans Nonferrous Met Soc China, 2007, 17(2): 238-243.
[3] SHEPELEVA L, BAMBERGER M. Microstructure of high pressure die cast AZ91D modified with Ca and Ce [J]. Materials Science and Engineering, 2006, 425(1): 312-317.
[4] SHANTHI M, LIM C Y H, LU L. Effects of grain size on the wear of recycled AZ91 Mg [J]. Tribology International, 2007, 40(2):335-338.
[5] LETZIG G D, KAINER K U. Measurement of crack induced damping of cast magnesium alloy AZ91 [J]. Journal of Alloys and Compounds, 2004, 378(1): 220-225.
[6] RAVI KUMAR N V, BLANDIN J J, DESRAYAUD C. Grain refinement in AZ91 magnesium alloy during thermo-mechanical processing [J]. Materials Science and Engineering, 2003, 359(1): 150-157.
[7] ???EK L, GREGER M, PAWLICA L, DOBRZA?SKI L A. Study of selected properties of magnesium alloy AZ91 after heat treatment and forming [J]. Journal of Materials Processing Technology, 2004, 158(1): 466-471.
[8] RAVI KUMAR N V, BLANDIN J J. Effect of alloying elements on the ignition resistance of magnesium alloys [J]. Scripta Materialia, 2003, 49(3): 225-230.
[9] JIANG Ju-fu, LUO Shou-jing. Microstructure evolution of processed Mg-Al-Zn alloy by equal channel angular extrusion in semi-solid isothermal treatment [J]. Trans Nonferrous Met Soc China, 2006, 16(6): 1313-1319.
[10] MICHAEL M. Magnesium and magnesium alloys [M]. Ohio: Materials Park, 2000.
[11] DENG Gang, ZHANG Jing-ping. Market and application of die-casting magnesium alloys [J]. Special Casting & Nonferrous Alloys, 2006(1): 46-49. (in Chinese)
[12] LIU Hong-wei, LUO Cheng-ping, LIU Jiang-wen. Effects of yttrium and mischmetal on microstructure and mechanical properties of Mg-Al-Zn alloy [J]. Special Casting & Nonferrous Alloys, 2003(5): 14-17. (in Chinese)
[13] PEI Li-xia, ZHANG Jin-shan, GAO Yi-bin. The effect of La on microstructure and macro-hardness of AZ91 magnesium alloy [J]. Research Studies on Foundry Equipment, 2005(1): 20-22.
[14] ZHANG Shi-chang, WEI Bo-kang, CHEN Wei-chen. Effect of yttrium and mischmetal on fluidity and solidification structures of AZ81 magnesium alloy [J]. Foundry, 2004, 53(2): 118-121.
[15] LUO Cheng-ping, LIU Wen-jiang, XIAO Xiao-ling. Methods of improving aged strengthening of AZ91 magnesium alloy [C]// Proceedings of the New Progress on Material Science and Engineering ’2002. Beijing: 2002: 688-700.
[16] GUO Qiang, YAN Hong-ge. Fracture behaviors of AZ80 magnesium alloy during multiple forging processes [J]. Trans Nonferrous Met Soc China, 2006, 16(4): 892-896.
Corresponding author: LI Quan-an; Tel: +86-379-64234194; E-mail: heitu100@163.com
(Edited by YANG Bing)