J. Cent. South Univ. Technol. (2008) 15(s1): 126-129
DOI: 10.1007/s11771-008-330-2

Viscoelastic rheological property of different types of
polymer solutions for enhanced oil recovery
MENG Ling-wei(孟令伟)1, KANG Wan-li(康万利)1, ZHOU Yang(周 阳)1,
WANG Zhi-wei(王志伟)1, LIU Shu-ren(刘述忍)1, BAI Bao-jun(白宝君)2
(1.Enhanced Oil Recovery Research Center, China University of Petroleum, Qingdao 266555, China;
2. Missouri University of Science and Technology, Rolla, MO 65401, USA)
Abstract: The capability of hydrophobic association polymer (HAPAM) to displace oil is different from that of hydrolyzed polyacrylamide (HPAM) because they have different rheological properties. The viscoelasticity of five polymers was measured using Physica MCR301 rheometer and was compared. The five polymers include three HAPAMs with relative molecular mass of 1 248×104 (TypeⅠ), 750×104 (TypeⅡ), and 571×104 (Type Ⅲ) separately and two HPAMs with relative molecular mass of 1 200×104 and 3 800×104 respectively. The experiment results indicate that the viscoelasticity of HAPAM is better than that of HPAM. The storage modulus G′ and the loss modulus G″ for HAPAM solutions are also larger than those for HPAM. Comparing the rheological curves of different HAPAM types, it is found that the viscosity of typeⅡ and type Ⅲ is almost same at different shear rates while the viscosity of type I is the lower than that of Types Ⅱ and Ⅲ. The storage modulus G′ and the loss modulus G″ for three types of HAPAM were measured in low oscillation frequency range, and the results show that G′ is greater than G″ for all three different types of HAPAM, but their loss modulus is almost same, and the G′ is in the order of type Ⅱ>type Ⅲ>type I. In addition, the G′ and G″ increase with aging time for all three HAPAM solutions were stayed at different days. The viscoelasticity of type Ⅰ reaches the highest value when aging time is 9 d at 45 ℃, but it is 7 d for type Ⅱ and type Ⅲ. The different viscoelasticity properties can be attributed to self-organization supermolecule networks which is formed by hydrophobic association of HAPAM molecular and molecular chain entanglement.
Key words: hydrophobic association polymer; rheology; storage modulus; loss modulus; self-organization; aggregate
1 Introduction
At present, polymer flooding has been widely applied for enhanced oil recovery(EOR) and can efficiently produce more than 10% oil recovery over water flooding. The polymer viscosity is mainly used to control the mobility ratio between water and oil phases thus improve sweep efficiency. Recent research also indicates that the viscoelasticity of polymer also aids in sweeping residual oil in porous blind end and oil film in pore or pore throats with abrupt change of radius, and thus improves displacing efficiency[1]. Hydrolyzed polyacrylamide (HPAM) is the polymer that is widely used for EOR currently and the rheological viscoelasticity of HPAM have been widely studied[2-4]. But HPAM has the disadvantages of easy degradation by shear, sensitivity to salt, etc. A new type of polymer, called hydrophobic association polymer (HAPAM) has been developed to minimize the disadvantages. A hydrophobic association polymer is a water-soluble polymer, onto which short hydrophobic side-chains have been grafted[5]. SHASHKINA et al[6] studied the hydrophobic aggregation in aqueous solutions of hydrophobically modified polyacrylamide in the vicinity of critical association concentration(CAC), and found that the rheological properties of aqueous polymer solutions depended on the aggregate in which the formation of hydrophobic domains plays a role of physical cross-links between polymer chains. CAO et al[7] studied the dynamic viscoelasticity of HAPAM using rheometer and also the dynamic viscoelasticity in porous media. HAPAM not only overcomes some weakness of HPAM, but it also has lots of additional advantages, such as increased viscosity, interfacial activity, solubilization and emulsification capability. In this work the viscous and viscoelasticity properties of different types of polymer were compared and the viscoelasticity variation with different standby time was studied. The structural strength of supermolecule networks was studied by stress scan.
2 Experimental
2.1 Materials and Instruments
Materials: HPAM with relative molecular mass of
1 200×104 and 3 800×104; HAPAM I, Ⅱ and Ⅲ with the relative molecular mass of 1 248×104, 750×104 and 571×104 respectively; NaCl analytically pure, made by Rui Jinte Chemical Limited Corporation, Tianjin; Deionized water and simulated water with NaCl concentration of 6.8 g/L.
Instrument: Physica MCR301 rheometer made in Anton Paar Company, Austria.
2.2 Preparation of sample solutions
Simulated water was prepared by NaCl with a concentration of 6.8 g/L.
Aqueous HAPAM solutions were prepared with the simulated water. The solutions were stirred at room temperature for at least 3 h. The concentrations for different hydrophobic association polymer were 2. 5 g/L.
2.3 Rheological measurements
The rheological measurements were carried out using a Physica MCR301 rheometer with a cone-plate measuring system. The temperature was maintained at (45±0.1) ℃. For the shear-dependent behavior, the viscosity measurements were carried out at shear rates ranging from 0.1 to 4 000 s-1 by rotational test.
3 Results and discussion
3.1 Dynamic viscoelasticity of HPAM with different relative molecular mass
Figs.1 and 2 show the dynamic viscoelasticity of HPAM with relative molecular mass of 1 200×104 and 3 800×104, respectively. Fig.1 also shows the stress scan results under the concentrations of 1.0 g/L and 2.5 g/L. It is found that the storage modulus G′ and the lose modulus G″ increase with increasing HPAM concentration and the viscoelasticity becomes more
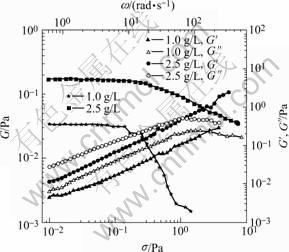
Fig.1 G′ and G″ vs frequency and G* vs stress (MW=1 200×104)
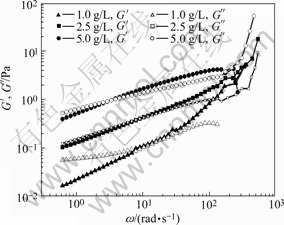
Fig.2 G′ and G″ vs frequency (MW=3 800×104)
obvious. There is a crossing point for G′ and G″ of each sample, where the frequency is called specified frequency(SF). In addition, SF moves to low frequency with increased HPAM concentration. The loss modulus G″ is larger than the storage modulus G′ when angular frequency is less than SF, under which the viscous component dominates the viscoelastic properties of HPAM solutions. However, when angular frequency is more than SF, the elasticity component becomes a dominating factor.
The value of G* in Fig.1 keeps constant with increasing stress (σ) until the critical stress value (σC) reaches, and then G* begins to decrease. The region that G* is independent of the applied stress amplitude is referred to linear viscoelastic region. When the linear viscoelastic region exists, it is implied that supermolecule networks of physical entanglement have formed in polymer solutions. Furthermore, the network structure strength depends on the linear stress region length[8]. Linear viscoelastic region with a concentration of 2.5 g/L is larger than that of 1.0 g/L.
3.2 Rheology and dynamic viscoelasticity of different HAPAM solutions
Fig.3 shows the rheological curves for 3 types of HAPAM. The results indicate that the viscosity plateau for II and Ⅲ are obvious, but type Ⅰ enters into the shear-thinning area as the shear rates increase very fast. The three samples all show obvious shear-thickening effect when the shear rate is more than 1 000 s-1. It is pointed out that the viscosity of type Ⅰ is the lowest, and the viscosity of Ⅱ and Ⅲ are almost same at same shear rates.
Fig.4 shows G′ and G′ of the 3 types of HAPAM and HPAM with a relative molecular mass of 3 800×104 at different angular frequency and stress. G* is almost the same for HAPAM in the linear viscoelastic region. However, the linear viscoelastic region length of HPAM
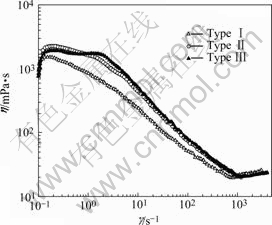
Fig.3 Rheological curves of HAPAM
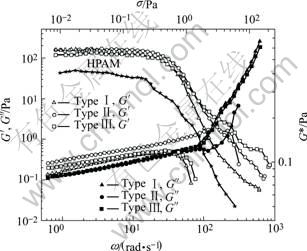
Fig.4 G′ and G″ vs frequency and G* vs stress
with 3 800×104 is smaller than that of HAPAM, which may be caused by the weak network structure strength of HPAM. The dynamic viscoelastic property in Fig.4 indicates G′ and G″ increase with angular frequency for the 3 systems. Just like HPAM, G′ and G″ also has a cross point (SF). But different from HPAM, G′ is larger than G″ when the angular frequency is less than SF, indicating that viscous component plays a role. G″ of the 3 types of HAPAM is almost same and the order of G′ is Ⅱ>Ⅲ>Ⅰ. As the angular frequency is greater than SF, the elasticity component plays a dominating role. When the angular frequency continuously increases, G′ will disappear. The supramolecular aggregates or molecular chain entanglement networks are destroyed due to the nonlinear viscoelasticity of polymer solutions at high angular frequency.
3.3 Dynamic viscoelasticity of 3 types of HAPAM with different days
G′ and G″ are drawn in different coordinate systems in order to show them clearly. Figs.5-7 present the dynamic viscoelasticity with different aging time, G″ is in a large coordinate system and G′ is in a small coordinate system. When the angular frequency is small, G′ and G″ for all of HAPAM solutions increase as aging time increases, and G′ is always greater than G″. The peak viscoelasticity with types Ⅰ is seen at the 9th day, and the peaks of the other two, types Ⅱand Ⅲ are seen at the 7th day. The rules are different at high angular frequency because of nonlinear viscoelasticity. According to Fig.4, G′ and G″ have a SF, so the SF changes as time goes on. It is known that G′ is equal to G″ at the crossing point, according to formula tan δ=G″/G′=1/(ωτ), the following equation could be obtained: ω=1/τ, where τ is characteristic relaxation time and the unit is s. τ is dependent on the molecular structure in polymer solution. Fig.8 shows the effect of aging time on characteristic relaxation time for 3 types of HAPAM. It is shown that τ of type Ⅰ decreases firstly, and then increases and stays steady from 9th day. However, τ of type Ⅱ increases until the 7th day, then keeps constant. The variation of type Ⅲ is similar to typeⅠ, but the max maximum value appears at the 7th
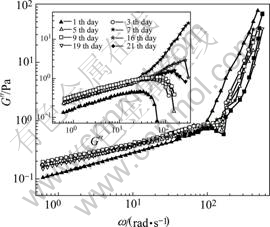
Fig.5 Dynamic viscoelasticity of typeⅠwith different placement days
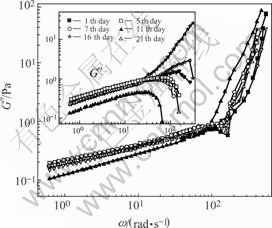
Fig.6 Dynamic viscoelasticity of type Ⅱ with different placement days
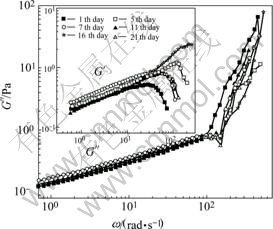
Fig.7 Dynamic viscoelasticity of type Ⅲ with different placement days
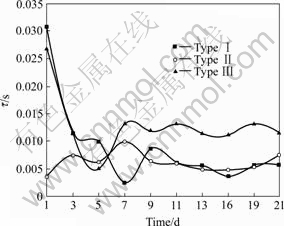
Fig.8 Characteristic relaxation time for 3 types of HAPAM
day. The changing rules of characteristic relaxation time correspond to the dynamic viscoelasticity. The difference of HAPAM viscoelasticity with the aging time results from HAPAM networks structure. HAPAM is water-soluble polymer with some hydrophilic macro chain. The hydrophobic group tends to form large supramolecular aggregates and form networks through self-association among intramolecular or intermolecular. Therefore, the viscous force increases since the intermolecular force increases. Meanwhile, the elasticity increases since supramolecular network strength increases. When HAPAM solutions are aged for different days, the association and dissociation of aggregates from molecular chain will occur because of the thermal motion or intermolecular force. Finally, association and dissociation reaches a new balance state.
4 Conclusions
1) HPAM solutions with relative molecular mass of 1 200×104 and 3 800×104 are viscous fluid when the angular frequency is smaller than SF, but they are elasticity fluid when the angular frequency is greater than SF. The larger linear viscoelastic region from stress scan is the result of stronger physical entanglement and supermolecule network formation.
2) Linear viscoelastic region and supermolecule network strength of HAPAM solutions are larger than those of HPAM solutions and thus have more viscoelasticity.
3) The dynamic viscoelasticity of HAPAM changes with aging time and the highest viscoelasticity of type Ⅰ is the 9th day, both of type Ⅱ and Ⅲ are the 7th day. The changing rules with characteristic relaxation time correspond to the dynamic viscoelasticity.
References
[1] ZHAO Feng, DU Yu-kou, LI Chang-xing, LI Chang-xing, TANG Ji-an, YANG Ping. Study on viscoelastic properties of partially hydrolyzed polyacrylamide solution [J]. Acta Phys-Chim Sin, 2004, 20(11): 1385-1388. (in Chinese)
[2] WANG De-min, JIANG You-lin, WANG Yan, GING Xiao-hong, WANG Gang. Rheology and special requirements on the flow system and equipment for large scale injection, production and gathering of viscous-elastic polymer fluids in chemical floods and methods to solve the problems that emerge[J]. SPE 77496, 2002: 1-8.
[3] XU Guan-li, SUN Gang, SHAO Zhen-bo, SHI Cheng-fang, JI Bing-yu. Study on aqueous solution viscosity and elasticity of blends of commercial partially hydrolyzed polyacrylamides with different relative molecular mass [J]. Oilfield Chemistry, 2006, 23(4): 341-344. (in Chinese)
[4] LI Dao-shan, KANG Wan-li, ZHU Hong-jun. Studies on viscoelasticity of aqueous hydrolyzed polyacrylamide solutions [J]. Oilfield Chemistry, 2003, 20(4): 347-349. (in Chinese)
[5] LAURENCE P, CAROLE K, NADEGE P, DOMINIQUE H. Synthesis of graft polyacrylamide with responsive self-assembling properties in aqueous media [J]. Polymer, 2007, 48: 7098-7112.
[6] SHASHKINA Y A, ZAROSLOV Y D, SIMIRNOV V A, PHILIPPOVA O E. Hydrophobic aggregation in aqueous solutions of hydrophobically modified polyacrylamide in the vicinity of overlap concentration [J]. Polymer, 2003, 44: 2289-2293.
[7] CAO Bao-ge, LUO Ping-ya, LI Hua-bin, ZHANG Yong. Viscoelasticity and rheological behaviors of hydrophobic association polymer solution [J]. Acta Petrolei Sinica, 2006, 27(1): 85-88. (in Chinese)
[8] XUE Xin-sheng, GUO Yong-jun, NIU Shuang-hui, GUO Guang-ji, ZHANG Xin-min. Research on viscoelasticity property of hydro water associative polyacrylamide [J]. Drilling Fluid & Completion Fluid, 2005, 22(3): 50-56. (in Chinese)
(Edited by YUAN Sai-qian)
Foundation item: Project(20873181) supported by the National Natural Science Foundation of China; Project(2007AA06Z214) supported by the High-tech Research and Development Program of China; Project(ts20070704) supported by Taishan Scholars Construction Engineering
Received date: 2008-06-25; Accepted date: 2008-08-05
Corresponding author: KANG Wang-li, Professor, Ph D, Tel: +86-13589332193; E-mail: Kangwanli@126.com