
Catalytic graphitization of PAN-based carbon fibers with electrodeposited Ni-Fe alloy
ZHOU Hai-hui1,2, PENG Qi-ling1, HUANG Zhen-hua1, YU Qiang1, CHEN Jin-hua1,2, KUANG Ya-fei1
1. College of Chemistry and Chemical Engineering, Hunan University, Changsha 410082, China;
2. State Key Laboratory of Chemo/Biosensing and Chemometrics, Hunan University, Changsha 410082, China
Received 8 April 2010; accepted 15 August 2010
Abstract: Ni-Fe alloy was electrodeposited on the surface of polyacrylonitrile (PAN)-based carbon fibers, and catalytic graphitization effect of the heat-treated carbon fibers was investigated by X-ray diffractometry and Raman spectra. It is found that Ni-Fe alloy exhibits significant catalytic effect on the graphitization of the carbon fibers at low temperatures. The degree of graphitization of the carbon fibers coated with Ni-Fe alloy (57.91% Fe, mass fraction) reaches 69.0% through heat treatment at 1 250 °C. However, the degree of graphitization of the carbon fibers without Ni-Fe alloy is only 30.1% after being heat-treated at 2 800 °C. The catalytic effect of Ni-Fe alloy on graphitization of carbon fibers is better than that of Ni or Fe at the same temperature, indicating that Ni and Fe elements have synergic catalytic function. Furthermore, Fe content in the Ni-Fe alloy also influences catalytic effect. The catalytic graphitization of Ni-Fe alloy follows the dissolution-precipitation mechanism.
Key words: Ni-Fe alloy; electrodeposition; degree of graphitization; carbon fibers
1 Introduction
Widespread attention has been paid to the graphitic materials, which have many unique properties, such as high electric conductivity, high thermal conductivity, high resistance to strong acids and bases. These unique properties result in many potential applications in the fields of filler for composite materials, electric materials, catalyst support[1-2], sensors[3-4], Li-ion battery[5], electrochemical capacitors[6], and so on. However, the properties of graphitic materials such as thermal and electric conductivities and tensile modulus can be affected by degree of graphitization. Therefore, the degree of graphitization is an important parameter for the use of carbon materials.
Adding certain inorganic or organic additives could accelerate the graphitization of carbon[7-8], with lower temperature, which is called catalytic graphitization[9]. In previous works, transition metals have been used as catalyst precursors for graphitization. To the best of our knowledge, Fe and Ni have been reported to have catalytic property to convert carbon materials into graphitic materials at low temperatures [10]. WEISWEILER et al[11] studied the graphitization of monolithic glasslike carbon with molten metals as catalysts, and they concluded that elements such as Co, Fe, Pt, Mo and Cr were highly effective in catalyzing the graphitization. Lately, MALDONADO-HODAR et al[12] used transition metals to produce graphite and supported that Cr and Fe seemed to be the best catalysts for graphitization of carbon aerogels. Ni has also been known to be one of the most effective catalysts for the graphitization of amorphous carbon[8, 13]. TZENG et al[7, 14] investigated the catalytic graphitization of electroless Ni-P-coated polyacrylonitrile (PAN)-based carbon fibers. And they proposed the possible mechanism of catalytic graphitization that nickel rather than phosphorous in the Ni-P coating should be responsible for the catalytic graphitization[14].
Up to date, we have not seen any report about catalytic graphitization of carbon materials adopting Ni-Fe alloy as catalyst. To obtain carbon fibers with a high degree of graphitization, the present study is focused on catalytic graphitization of PAN-based carbon fibers with electrodeposited Ni-Fe alloy.
2 Experimental
The PAN-based carbon fibers T-700 were obtained from Japan Toray corporation and ultrasonically washed in an alkaline solution for 50 min. The electrolyte was prepared using AR grade reagents (purchased from Xi Long chemical factory in Shantou, China) and double-distilled water. The bath composition and parameters used for electrodepositing Ni-Fe alloy are listed in Table 1. The ratio of Ni to Fe in Ni-Fe alloy was controlled by changing the concentrations of nickelous sulfate and ferrous sulfate. Energy dispersive spectrum (EDS) was performed to detect the compositions of the Ni-Fe alloy coatings and the results are listed in Table 2. The samples without catalyst were denoted as HTT-(X) (HTT=heat treatment temperature, X=temperature values), and the samples with catalyst were abbreviated as HTT-(W)-(X) (X=temperature values, W=w(Fe)). Under argon atmosphere, the samples were kept in electric resistance furnace at targeted temperatures for 120 min.
Table 1 Bath composition and parameters used for electrodepositing Ni-Fe alloy
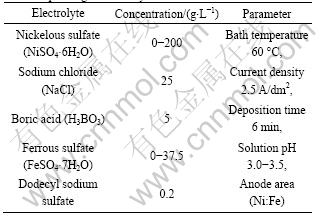
Table 2 Quantitative chemical compositions of different Ni-Fe alloys

X-ray diffraction (XRD) data were collected on a Rigaku Dmax-2400X instrument using Cu Kα radiation (λ=0.154 06 nm) by scanning in the 2θ range of 23°-29°. The morphologies of the samples were characterized by JSM-6700F scanning electron microscope (SEM). Raman spectra of heat-treated carbon fibers were recorded using LABRAM-010 Raman microscope. Gaussian fitting procedure was applied to high-frequency (G-band) and low-frequency (D-band) peaks of each spectrum.
3 Results and discussion
3.1 Effect of heat treatment temperature
X-ray diffraction peak profile analysis is a widely used method for the determination of d002, crystallite size (Lc) and degree of graphitization of carbon materials[15-16]. Fig.1 shows the XRD patterns of carbon fibers without alloy coating at different heat treatment temperatures (HTTs). It can be observed from Fig.1 that the peak becomes sharp with the increase of HTT in addition to the shift of the (002) peak to the right. Moreover, when the carbon fibers were heat-treated at 2 800 °C, (002) peaks could only be seen as a very broad hump, which indicates the non-graphitization characteristic. Fig.2 shows the XRD patterns of carbon fibers with Ni-Fe alloy coating (mass fraction of Fe is 57.91 %) at different HTTs. It is noted that the (002) peak shifts to right and becomes sharp with the increase of HTT. The (002) peak changes from an asymmetric one at 1 250 °C to a symmetric one at above 1 300 °C. Table 3 summarizes the values of d002, Lc and degree of graphitization of the PAN-based carbon fibers with and
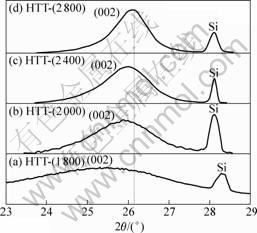
Fig.1 XRD patterns of PAN-based carbon fibers without catalyst at different HTTs: (a) 1 800 °C; (b) 2 000 °C; (c) 2 400 °C; (d) 2 800 °C
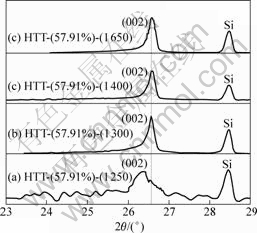
Fig.2 XRD patterns of PAN-based carbon fibers with catalyst at different HTTs: (a) 1 250 °C; (b) 1 300 °C; (c) 1 400 °C; (d) 1 650 °C
Table 3 Interlayer spacing (d002), crystallite size (Lc) and degree of graphitization (G) of PAN-based carbon fibers after being heat-treated at different HTTs
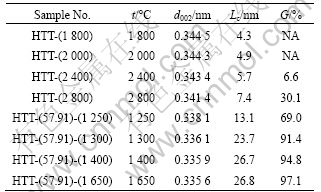
without catalyst at different HTTs. From Table 3, it can be found that the d002 value of carbon fibers without alloy coating decreases from 0.344 5 nm to 0.341 4 nm, and Lc increases from 4.3 nm to 7.4 nm with increasing HTT from 1 800 °C to 2 800 °C , demonstrating the growth of graphite crystals. When the HTT was increased to 2 800 °C, the degree of graphitization is only 30.1%, indicating that graphitization process is very slow. However, for the carbon fibers with alloy coating, with increasing HTT from 1 250 °C to 1 650 °C, d002 value decreases from 0.338 1 nm to 0.335 6 nm, which is very close to 0.335 4 nm for natural graphite. Lc increases from 13.1 nm to 26.8 nm, and the degree of graphitization increases from 69.0% to 97.1%. Thus, it is obvious that Ni-Fe alloy has a significant catalytic graphitization effect at low temperature.
Raman spectroscopy is commonly used to analyze the microscopic bonding structure of carbon-related materials, which is useful for characterizing various carbon materials[17-20]. Fig.3 shows the Raman spectra for the carbon fibers without alloy coating (Fig.3(a)) and with alloy coating (Fig.3(b)) in the region from 1 100 to 1 880 cm-1. As shown in Fig.3(a) and Fig.3(b), D-band gradually becomes weak with the increase of HTT. Compared with D-band, G-band distinctly becomes strong, demonstrating that graphite component increases and disordered carbon decreases. The ratio of D-band area to G-band area (AD/AG) is a function of HTT. The smaller the value of AD/AG, the higher the degree of graphitization. The decrease of AD/AG with increasing HTT indicates the growth of graphitic domains, i.e. the increase of graphitization. Fig.4 presents the relationship between AD/AG and HTT for carbon fibers with and without catalyst. The value of AD/AG for the carbon fibers without catalyst decreases from 1.42 to 0.68 when HTT increases from 1 800 °C to 2 800 °C. However, the value of AD/AG for the carbon fibers with catalyst (mass fraction of Fe is 57.91%) decreases from 2.30 to 0.05 when HTT increases from 1 250 °C to 1 650 °C. Consequently, higher HTT can accelerate graphitization process.
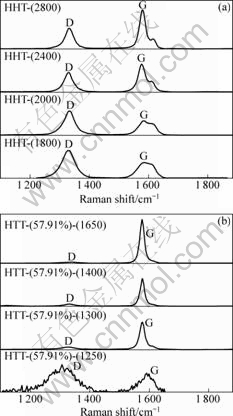
Fig.3 Raman spectra of carbon fibers treated at different HTTs: (a) Samples without catalyst; (b) Samples with catalyst containing 57.91% Fe
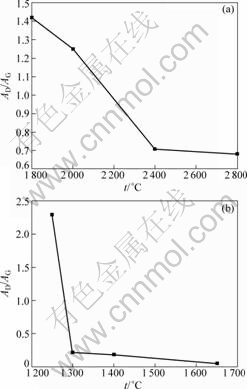
Fig.4 Effect of heat treatment temperature on AD/AG for carbon fibers without catalyst (a) and carbon fibers with catalyst (b)
3.2 Effect of Fe content in Ni-Fe alloy coating
XRD patterns of the carbon fibers with catalyst containing different Fe contents treated at 1 300 °C are shown in Fig.5. From Fig.5, it can be observed that the (002) peak first becomes sharp and gets blunt afterwards with the increase of Fe content. Furthermore, it should be mentioned that the (002) peak first shifts to the right and then to the left. Table 4 presents the values of the d002 , Lc and degree of graphitization of the carbon fibers coated with different Fe contents catalysts after being heat-treated at 1 300 °C. As the Fe content in Ni-Fe alloy was increased to 57.91%, a decrease of d002 from 0.339 2 nm to 0.336 1 nm was obtained. Moreover, an obvious increase of Lc from 7.0 nm to 23.7 nm was found. When the Fe content in Ni-Fe alloy was increased from 57.91% to 100%, d002 increased from 0.336 1 nm to 0.336 6 nm. However, a decrease of Lc value from 23.7 nm to 17.8 nm was observed. From Table 4 and Fig.5, it can be observed that the Ni-Fe alloy has better catalytic effect on graphitization than Ni or Fe alone, and the ratio of Fe to Ni has a significant influence on catalytic effect. The alloy containing 57.91% Fe has the best catalytic effect on graphitization, and the degree of graphitization reaches 91.4%. Consequently, Ni-Fe alloy exhibits a significant synergic catalytic effect for the graphitization of PAN-based carbon fibers. The Raman spectra of the carbon fibers with catalyst containing different Fe contents treated at 1 300 °C are illustrated in Fig.6. It can be observed that D-band gradually becomes weak at first and then becomes strong with the increase of Fe content in Ni-Fe alloy. However, the change tendency of G-band is adverse. Fig.7 depicts the values of AD/AG for the carbon fibers with catalyst containing different Fe contents treated at 1 300 °C. Apparently, the degree of graphitization initially increases with the increase of Fe content in Ni-Fe alloy and decreases afterwards. The results of Raman measurements are consistent with the results obtained from XRD data.
Table 4 Interlayer spacing (d002), crystallite size (Lc) and degree of graphitization (G) of carbon fibers coated with different Fe contents catalysts after being heat-treated at 1 300 °C
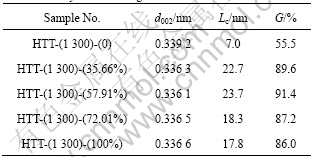
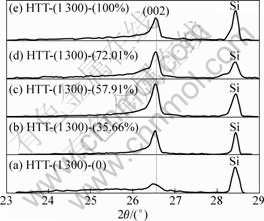
Fig.5 XRD patterns of carbon fibers with catalyst containing different Fe contents: (a) 0; (b) 35.66%; (c) 57.91%; (d) 72.01%; (e) 100%
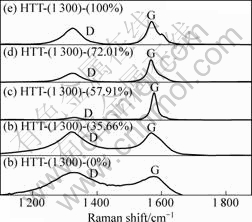
Fig.6 Raman spectra of carbon fibers with catalyst containing different Fe contents: (a) 0; (b) 35.66%; (c) 57.91%; (d) 72.01%; (e) 100%
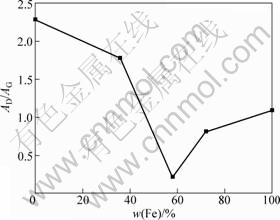
Fig.7 Values of AD/AG for carbon fibers with catalyst containing different Fe contents at 1 300 °C
3.3 Catalytic mechanism for graphitization
At present, catalytic mechanism for graphitization is not well defined. According to Acheson’s work, the formation of graphite involved a step in which carbon first combined with catalyst and then dissociated[21]. FITZER and KEGEL[22] firstly proposed the dissolution-precipitation mechanism. KONNO and SINCLAIR[23] adopted cobalt as catalyst for graphitization, and proposed the dissolution-precipitation mechanism. In accordance with the dissolution- precipitation mechanism, some scholars conjectured the formation and decomposition of unstable loosely bonded carbide [10]:
C(disordered)+Me→MeC→Me+C(graphite)
In the present study, Ni-Fe alloy was adopted as catalyst for graphitization of carbon fibers. When Ni-Fe alloy reached the melting point, the molten alloy dissolved carbon. Then, carbon precipitated as graphite, and the molten alloy was forced out of graphitic crystals. Fig.8 shows the SEM images of the carbon fibers with catalyst after being heat-treated at definite HTTs and the original carbon fibers. The results were similar to the phenomena in TZENG’s experiment[7]. From Fig.8, it can be observed that the morphology of the carbon fibers coated with catalyst changes evidently after heat treatment. The carbon fibers’ sectional and external parts change from flat to coarse after catalytic graphitization. From Fig.8(b), it can be observed that the outer parts of the carbon fibers are rougher than the center. Moreover, there existed micropores on the carbon fibers, as shown in Fig.8(d), which is different from Fig.8(c). Apparently, Fig.8 indicates that the process of catalytic graphitization of the carbon fibers proceeded from the outer surface to the center, which is consistent with the dissolution- precipitation mechanism. However, according to CACCIAMANI’s phase diagram[24], the melting point of Ni-Fe alloy depends on the ratio of Ni to Fe. When Ni and Fe formed Ni-Fe alloy, the melting point is lower than that of Ni or Fe alone. Moreover, when Fe content is 57.91% (mass fraction), the melting point of the Ni-Fe alloy is the lowest among the Ni-Fe alloys with Fe content from 35.66% to 72.01% (mass fraction). The molten metals with low melting point easily dissolve carbon, and gradually convert the PAN-based carbon fibers to finer graphite mass. In addition, the conversion of sp3 carbon to sp2 carbon would occur, which can lead to sp2 carbon clustering and eventual graphitization.
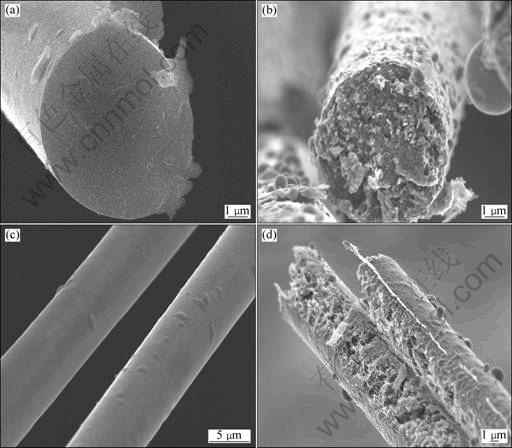
Fig.8 SEM images of carbon fibers: (a) Sectional configuration of original carbon fiber; (b) Sectional configuration of carbon fibers after graphitization; (c) External configuration of original carbon fibers; (d) External configuration of carbon fibers after graphitization
4 Conclusions
1) Ni-Fe alloy has a significant catalytic effect on the graphitization of PAN-based carbon fibers. The degree of graphitization of PAN-based carbon fibers coated with Ni-Fe alloy (57.91% Fe) reaches 69.0% after being heat-treated at 1 250 °C, which is much higher than that of the original carbon fibers (30.1%) after being heat-treated at 2 800 °C.
2) Ni-Fe alloy has better catalytic effect on graphitization than Ni or Fe alone at the same temperature, indicating that Ni and Fe elements have synergic catalytic function.
3) The Fe content in Ni-Fe alloy has a significant influence on catalytic effect and has an optimum value of 57.91%.
4) The catalytic graphitization of Ni-Fe alloy follows the dissolution-precipitation mechanism.
References
[1] JAYASRI D, NARAYANAN S S. Electrocatalytic oxidation and amperometric determination of BHA at graphite wax composite electrode with silver hexacyanoferrate as electrocatalyst [J]. Sensors and Actuators B, 2006, 119(1): 135-142.
[2] JEONG S H, LIM D C, BOO J H, LEE S B, HWANG H N, HWANG C C, KIM Y D. Interaction of silver with oxygen on sputtered pyrolytic graphite [J]. Applied Catalysis A, 2007, 320: 152-158.
[3] SANTINI A O, OLIVERIA J E, PEZZA H R, PEZZA L. A new potentiometric ibuprofenate ion sensor immobilized in a graphite matrix for determination of ibuprofen in tablets [J]. Microchemical Journal, 2006, 84(1): 44-49.
[4] RODRIGUEZ N P, COFRE R, ZAGAL J H, BEDIOUI F. Electrocatalytic activity of cobalt phthalocyanine CoPc adsorbed on a graphite electrode for the oxidation of reduced l-glutathione (GSH) and the reduction of its disulfide (GSSG) at physiological pH [J]. Bioelectrochemistry, 2007, 70(1): 147-154.
[5] ROCKA N L, KUMTA P N. Synthesis and characterization of electrochemically active graphite-silicon-tin composite anodes for Li-ion applications [J]. Journal of Power Sources, 2007, 164(2): 829-838.
[6] WANG H Y, YOSHIO M. Graphite, a suitable positive electrode material for high-energy electrochemical capacitors [J]. Electrochemistry Communications, 2006, 8(9): 1481-1486.
[7] TZENG S S. Catalytic graphitization of electroless Ni-P coated PAN-based carbon fibers [J]. Carbon, 2006, 44(10): 1986-1993.
[8] MARSH H, WARBURTON A P. Catalysis of graphitization [J]. Journal of Applied Chemistry of the USSR, 1970, 20(5): 133-142.
[9] ZHOU H H, YU Q, PENG Q L, WANG H, CHEN J H, KUANG Y F. Catalytic graphitization of carbon fibers with electrodeposited Ni-B alloy coating [J]. Materials Chemistry and Physics, 2008, 110(2-3): 434-439.
[10] RESHETENKO T V, AVDEEVA L B, ISMAGILOV Z R, PUSHKAREV V V, CHEREPANOVA S V, CHUVILIN A L, LIKHOLOBOV V A. Catalytic filamentous carbon: Structural and textural properties [J]. Carbon, 2003, 41(8): 1605-1615.
[11] WEISWEILER W, SUBRAMANIAN N, TERWIESCH B. Catalytic influence of metal melts on the graphitization of monolithic glasslike carbon [J]. Carbon, 1971, 9(6): 755-761.
[12] MALDONADO-HODAR F J, CASTILLA C M, RIVERA- UTRILLA J, HANZAWA Y, YAMADA Y. Catalytic graphitization of carbon aerogels by transition metals [J]. Langmuir, 2000, 16(9): 4367-4373.
[13] OYA A, OTANI S. Catalytic graphitization of carbons by various metals [J]. Carbon, 1979, 17(2): 131-137.
[14] TZENG S S, LIN Y H. The role of electroless Ni-P coating in the catalytic graphitization of PAN-based carbon fibers [J]. Carbon, 2008, 46(3): 555-558.
[15] SEVILLA M, FUERTES B A. Catalytic graphitization of templated mesoporous carbons [J]. Carbon, 2006, 44(3): 468-474.
[16] NODA T, KATO H. Heat treatment of carbon under high pressure [J]. Carbon, 1965, 3(3): 289-290.
[17] RUSOP M, SOGA T, JIMBO T, UMENO M, SHARON M. Semiconducting amorphous camphoric carbon nitride thin films [J]. Surface Review and Letters, 2005, 12(4): 587-595.
[18] BALDAN M R, ALMEIDA E C, AZEVEDO A F, GONCALVESB E S, REZENDE M C, FERREIRAA N G. Raman validity for crystallite size La determination on reticulated vitreous carbon with different graphitization index [J]. Applied Surface Science, 2007, 254(2): 600-603.
[19] GONG Q J, LI H J, WANG X, Fu Q G, WANG Z W, LI K Z. In situ catalytic growth of carbon nanotubes on the surface of carbon cloth [J]. Composites Science and Technology, 2007, 67(14): 2986-2989.
[20] WANG Y, ALSMEYER D C, MCCREERY R L. Raman spectroscopy of carbon materials: structural basis of observed spectra [J]. Chem Mater, 1990, 2(5): 557-563.
[21] BARANIECKL C, PINCHBECK P H, PICKERING F B. Some aspects of graphitization induced by iron and ferro-silicon additions [J]. Carbon, 1969, 7(2): 213-218.
[22] FITZER E, KEGEL B. Reactions of carbon saturated vanadium carbide with disordered carbon (effects on catalytic graphitization) [J]. Carbon, 1968, 6(4): 433-436.
[23] KONNO T J, SINCLAIR R. Crystallization of amorphous carbon in carbon-cobalt layered thin films [J]. Acta Metallurgica et Materialia, 1995, 43(2): 471-484.
[24] CACCIAMANI G, KEYZER J D, FERRO R, KLOTZ U E, LACAZE J, WOLLANTS P. Critical evaluation of the Fe-Ni Fe-Ti and Fe-Ni-Ti alloy systems [J]. Intermetallics, 2006, 14(10-11): 1312-1325.
聚丙烯腈基炭纤维表面电沉积Ni-Fe合金的催化石墨化性能
周海晖1,2, 彭奇龄1,黄振华1,余 强1,陈金华1,2,旷亚非1
1. 湖南大学 化学化工学院,长沙 410082;
2. 湖南大学 化学/生物传感与计量学国家重点实验室, 长沙 410082
摘 要:在聚丙烯氰基炭纤维上电沉积Ni-Fe合金催化剂,并采用X-射线衍射和拉曼光谱方法考察其对热处理炭纤维石墨化的催化性能。结果表明:Ni-Fe合金在低温条件下对炭纤维石墨化有着优异的催化性能。附有Ni-Fe合金(铁的质量分数为57.91%)的聚丙烯腈基炭纤维经过1 250 ℃热处理后其石墨化度为69.0%,而空白样炭纤维经过2 800 ℃热处理后其石墨化度仅为30.1%。在相同温度下,Ni-Fe合金对碳纤维石墨化的催化性能优于纯铁和纯镍的,这表明Ni和Fe元素之间存在协同催化作用。同时,研究表明Ni-Fe合金对石墨化的催化遵循溶解再析出机理。
关键词:镍-铁合金;电沉积;石墨化度;碳纤维
(Edited by YANG Hua)
Foundation item: Project (2006CB600903) supported by the National Basic Research Program of China; Project (2010GK3208) supported by Science and Technology Program of Hunan Province, China
Corresponding author: KUANG Ya-fei; Tel: +86-731-88821874; Fax: +86-731-88713642; E-mail: yafeik@163.com
DOI: 10.1016/S1003-6326(11)60754-1