Removal of cadmium and zinc ions from aqueous solution by living Aspergillus niger
LIU Yun-guo(刘云国)1, FAN Ting(樊 霆)1, ZENG Guang-ming(曾光明)1,
LI Xin(李 欣)1, TONG Qing(童 庆)1, 2, YE Fei(叶 菲)1, ZHOU Ming(周 鸣)1,
XU Wei-hua(徐卫华)1, HUANG Yu-e(黄玉娥)1
1. Department of Environmental Science and Engineering, Hunan University, Changsha 410082, China;
2. School of Resources and Environmental Engineering, Wuhan University of Technology,
Wuhan 430070, China
Received 25 October 2005; accepted 6 March 2006
Abstract: The potential of living Aspergillus niger to remove cadmium and zinc from aqueous solution was investigated. Effects of pH, initial concentration, contact time, temperature and agitation rate on the biosorption of Cd(Ⅱ) and Zn(Ⅱ) ions were studied. The optimum adsorption pH value for Cd(Ⅱ) and Zn(Ⅱ) were 4.0 and 6.0. The best temperature and agitation rate were in the range of 25-30 ℃ and 120 r/min for all metal ions. Under the optimal conditions, the maximum uptake capacities of Cd(Ⅱ) and Zn(Ⅱ) ions are 15.50 mg/g and 23.70 mg/g at initial concentrations of 75 mg/L and 150 mg/L, respectively. Biosorption equilibrium is established within 24 h for cadmium and zinc ions. The adsorption data provide an excellent fit to Langmuir isotherm model. The results of the kinetic studies show that the rate of adsorption follows the pseudo-second order kinetics.
Key words: Aspergillus niger; biosorption; cadmium; zinc; biosorption isotherm; kinetic model
1 Introduction
Heavy metals like copper, mercury, chromium, cadmium, lead, nickel and zinc cause serious threat to environment, animals and human for their extreme toxicity[1]. Many industries including metal plating, mining, battery, pigment, dyestuff and chemical industries release heavy metal like cadmium, zinc in waste streams[2]. Heavy metal ions are commonly removed by chemical precipitation, ion exchange and chemical oxidation-reduction[3], but these methods are relatively expensive and ineffective especially when the concentration of heavy metal is low. Biological treatment, based on living or non-living microorganisms or plants, offers the advantages, such as low operating cost and high efficiency[4].
Metal accumulative bioprocesses generally are divided into two broad categories: biosorption (passive process) and bioaccumulation (active process)[5]. It should be pointed out that the term ‘biosorption’ has been used to describe the passive non-metabolic mediated process of metal ion binding by living or inactivated biomass[6]. Using living cultures in bioremediation could avoid the productive process of powdering and drying[7], but the application of living cells to remove metal has some limitations, such as sensitivity of pH value, high metal concentration and external metabolic energy[8]. However, such problems can be overcome via strain selection and exploitation of organic wastes as carbon substrates.
Many microbial species such as algae, bacteria and fungi are known to have high metal adsorbing capacities [9,10]. Among the main strains, fungi biomass offers the advantage of having a high percentage of cell wall material that shows excellent metal–binding properties. Aspergillus niger(A.niger) was used for the production of citric acid and enzymes, and also for removing heavy metal[11]. However, the study of removing heavy metal by living A. niger is limited. In this study, active A. niger was used as sorbent for removal of heavy metal.
The purpose of the present study is to investigate the ability of living A. niger in Cd(Ⅱ) and Zn(Ⅱ) removal. The effects of the pH, initial metal concentration, temperature, contact time and agitation rate on biosorption of Cd(Ⅱ) and Zn(Ⅱ) using active A. niger biomass were described. In addition to this, the biosorption equilibrium isotherm and kinetic model were also studied. 2 Material and methods 2.1 Microorganism
Aspergillus niger (AF 91004) used in this study was obtained from China Center for Type Culture Collection (CCTCC) and maintained on potato-dextrose agar (PDA) slants and stored at 4 ℃. Subculture was made every 3 months.
The fungus was cultivated on a rotary shaker (120 r/min) at 26 ℃ for 72 h in 250 mL conical flask containing 100 mL of growth medium. The growth medium consisted of (g/L): dextrose, 20; peptone, 10; NaCl, 0.2; CaCl2, 0.1; KCl, 0.1; K2HPO4, 0.5; NaHCO3, 0.05; MgSO4, 0.25; Fe(SO4)2·7H2O, 0.005. After this period, the harvested biomass was filtered and washed three times with sterile physiological saline.
2.2 Metal solutions
Stock solution of 1 000 mg/L of cadmium and zinc solutions were prepared using nitrate salts of analytical grade. The working metal solutions were prepared in physiological saline (0.9%). Before mixing the microorganisms, the pH value of each test metal solution was adjusted to desirable value with 1 mol/L NaOH and 1 mol/L HCl.
2.3 Biosorption experiments
The biosorption experiments were carried out using the batch equilibration at different pH values, initial metal concentration, contact time, temperature and agitation rate. The test solutions of Cd(Ⅱ) and Zn(Ⅱ) ions were agitated on a shaker for 72 h which was enough for adsorption equilibrium. Unless otherwise conditions stated, the initial metal concentration, temperature and agitation rate were 50 mg/L, 26 ℃ and 120 r/min, respectively. In kinetic studies, samples were taken out at different intervals. Equal wet biomass was added to each flask, and its corresponding dry wet (0.2 g) of the biomass was determined at each batch of experiment by drying it at 105 ℃ for 2 h. All experiments were carried out in 250 mL conical flask with 100 mL metal solution on a rotary shaker. Before analysis the samples were centrifuged at 4 000 r/min for 10 min, then the supernatant was analyzed for the remaining metal ion concentrations by using an atomic adsorption spectrophotometer (PE AA700). All the experiments were conducted in twice and average values were used in the analysis.
The amount of heavy metal adsorbed by the biomass was calculated using the following equation:
(1)
where Q is the amount of metal adsorbed by biomass, mg/g; ρ0 is the initial concentration of metal ion, mg/L; ρe is the final concentration of metal ion, mg/L; m is the mass of the biomass in the adsorption medium, g; V is the initial volume of the adsorption medium, L[12,13].
3 Results and discussion 3.1 Effect of pH on metal removal
It is well known that metal ion adsorption on both non-specific and specific sorbents is pH dependent[14]. The solution pH affects the chemistry of the metals, the activity of functional groups (carboxylate, phosphate and amino groups) on the cell wall as well as the competition of metallic ions for the binding site[15]. The effects of the initial pH on biosorption of Cd(Ⅱ) and Zn(Ⅱ) ions are evaluated in the pH range of 2.5-6.0 and 1.5-7.0, respectively(Fig.1). It can be seen that metal uptake increases with increasing pH in the range of pH 3.0-4.0 for Cd(Ⅱ) and pH 3.0–6.0 for Zn(Ⅱ), and maximum Cd(Ⅱ) and Zn(Ⅱ) uptake capacities are 15.1 mg/g and 18.25 mg/g at pH 4.0 and 6.0, respectively. At low pH (<2.0) the biosorption capacity for all metal ions is very low, because large hydrogen ions compete with metal ions at sorption sites. As the pH (from 3.0 to 6.0) increases, more negatively charged cell surface becomes available thus facilitating greater metal uptake [12]. However, metal precipitates at high pH values (>7.0) inhibit the contact of metal with the most fungal biomass[16].
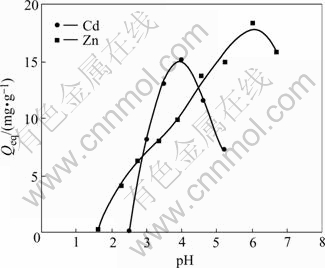
Fig.1 Effect of pH on biosorption of Cd(Ⅱ) and Zn(Ⅱ) ions (ρ0=50 mg/L, θ= 26 ℃, t=72 h, r=120 r/min)
3.2 Effect of initial metal ion concentration
Fig.2 shows Cd(Ⅱ) and Zn(Ⅱ) uptake capacities of A. niger as a function of the initial concentration of metal ions (in the range of 25-200 mg/L) in the medium. The adsorbed amount of Cd(Ⅱ) and Zn(Ⅱ) ions increases with the increasing initial metal ion concentration. This uptrend may be due to an increase in electrostatic interactions, involving sites of progressively lower affinity for metal ions[15]. From the figure, no more metal uptake by cells is observed over 75 mg/L initial Cd(Ⅱ) concentrations and 150 mg/L initial Zn(Ⅱ) concentrations, receptively. The maximum Cd(Ⅱ) and Zn(Ⅱ) uptake capacity are determined as 17.35 mg/g and 24.60 mg/g, respectively.
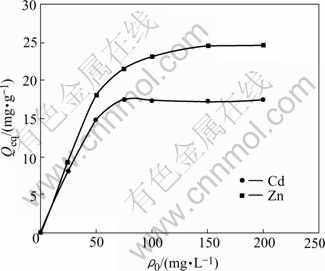
Fig.2 Effect of initial metal ion concentration on biosorption of Cd(Ⅱ) and Zn(Ⅱ) ions (pH=4 for Cd (Ⅱ); pH=6 for Zn (Ⅱ), θ=26 ℃, t=72 h, r=120 r/min)
When compared with the adsorptive capacity values that have been reported, the maximum Cd(Ⅱ) and Zn(Ⅱ) sorption with A. niger could be promising. For example, HOLAN and VOLESKRY[17] obtained 19 mg/g Cd(Ⅱ), and Zn(Ⅱ) uptake capacities were 21.6 mg/g by Myxococcus xanthus and 13.1mg/g by Saccharomyces cerevisiae[18]. The maximum Cd(Ⅱ) and Zn(Ⅱ) uptake capacities by Mocus rouxii were 8.46 mg/g and 7.75 mg/g, respectively[19].
3.3 Effect of contact time
The contact time is of great importance in adsorption for the assessment of the suitability of these fungal preparations to serve as biosorbents in a continuous flow system. Fig.3 shows the biosorption time curves of Cd(Ⅱ) and Zn(Ⅱ) ions on live biomass at the initial concentration of 50 mg/L. It can be seen that biosorption consists of two phases: a primary rapid phase that accounts for the major part in the total metal biosorption, and a second slow phase that contributes to a relatively small part. Most of the metal ions are adsorbed from aqueous solution within the first 6 h and there is no more metal ions uptake after 24 h. This trend suggests that the uptake may be due to the interaction with functional groups located on the surface of the cells at the first rapid stage, and intercellular accumulation in the second phase.
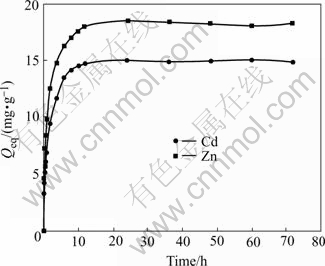
Fig.3 Effect of contact time on biosorption of Cd(Ⅱ) and Zn(Ⅱ) ions (pH=4 for Cd(Ⅱ); pH=6 for Zn(Ⅱ), ρ0=50 mg/L, θ=26 ℃, r=120 r/min)
Data on the contact time of heavy metal ions by various biosorbents have shown a wide range of adsorption time. The biosorption equilibrium time of Pb(Ⅱ) on the dead A. niger was 5 h[10]. YAN[19] observed that Cd(Ⅱ) and Zn(Ⅱ) biosorption on pretreated Mucor rouxii biomass reached equilibrium in 6 h and 7 h at initial pH 6.0, respectively. The Cd(Ⅱ) and Zn(Ⅱ) biosorption rate of immobilized Funalia trogii reached saturation within 1 h[20]. However, the biosorption of Cr(Ⅵ) adsorbed on dead bacterium Baccllus thuringiensis was very fast and reached equilibrium within 15 min[21]. There are several para- meters that determine the biosorption rate such as the stirring rate of the aqueous phase, structural properties both of the support and the biosorbent, amount of sorbent, properties of the ion under study, initial concentration of ionic species and, of course, existence of other metal ions, which may compete with the ionic species of interest for the active biosorption sites. Therefore, it is very difficult to compare the biosorption times reported.
3.4 Effect of temperature
Fig.4 indicates that the change of temperature influences the biosorption rates of Cd(Ⅱ) and Zn(Ⅱ) ions by A. niger. Maximum biosorption of Cd(Ⅱ) and Zn(Ⅱ) is obtained at different temperatures in the range of 25-30 ℃. The increase of metal uptake at higher temperature may be due to either higher affinity of sites for metal or more binding sites on the relevant cell mass. At higher temperature the energy of the system facilitates Cd(Ⅱ) and Zn(Ⅱ) ions attaching on the surface and also when the temperature is very high, there is a decrease of metal sorption due to distortion of some sites of the cell surface available for metal biosorption [22].
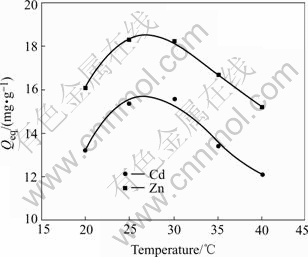
Fig.4 Effect of temperature on biosorption of Cd(Ⅱ) and Zn(Ⅱ) ions (pH=4 for Cd(Ⅱ); pH=6 for Zn(Ⅱ), ρ0=50 mg/L, t=72 h, r=120 r/min)
3.5 Effect of agitation rate
The effect of shaker rotation speed (0-200 r/min) on biosorption by A. niger was studied. Optimal value of adsorption capacity is obtained at the stirring speed of 120 r/min as shown in Fig.5. This indicates that the shaking rate assures all the cell wall binding sites are available for cadmium and zinc uptake. That is to say, a moderate speed gives the best homogeneity for the mixture suspension. At high stirring speed, vortex phenomena occurs and the suspension is no longer homogenous which makes the adsorption of Cd(Ⅱ) and Zn(Ⅱ) difficult.
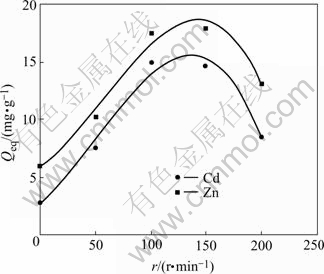
Fig.5 Effect of agitation rate on biosorption of Cd(Ⅱ) and Zn(Ⅱ) ions (pH=4 for Cd(Ⅱ); pH=6 for Zn(Ⅱ), ρ0=50 mg/L, θ=26 ℃, t=72 h)
3.6 Equilibrium study
There are many theoretical models to describe the adsorption process of heavy metal ions by active and inactive microorganisms. Among these models, the Langmuir and Freundlich equilibrium models have been applied in this study. The Langmuir isotherms equation is valid for monolayer sorption onto surface containing finite number of identical sorption sites which is described by the following equation:
(2)
where Qeq (mg/g) and ρeq (mg/L) are the amount of adsorption and the residual concentration in solution at equilibrium, respectively; Qmax (mg/g) and b are Langmuir constants denoting maximum adsorption capacity and the affinity of the binding sites, respectively, which can be determined by the linearised Langmuir isotherm as below:
(3)
The Freundlich equation is purely empirical based on sorption on a heterogeneous surface, which is commonly presented as
(4)
where KF and n are the Freundlich constants related to adsorption capacity and the adsorption intensity, respectively.
The Langmuir adsorption isotherms of Cd(Ⅱ) and Zn(Ⅱ) ions obtained at 26 ℃ are shown in Fig.6. The adsorption constants and correlation coefficients of Langmuir and Freundlich isotherms are given in Table 1.
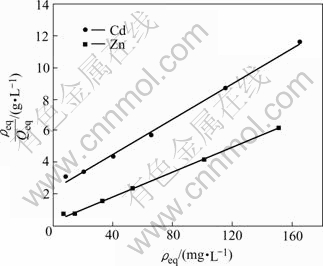
Fig.6 Langmuir adsorption isotherm of Cd(Ⅱ) and Zn(Ⅱ) on A.niger (pH=4 for Cd (Ⅱ); pH=6 for Zn (Ⅱ), θ=26 ℃, t=24 h, r=120 r/min)
In view of the correlation regression coefficients, the adsorption process can be well described by Langmuir model. The Langmuir constant (Qmax) values are close to the experimental values, and this can be evidence that the surface of the sorbent is homogenous and sorption stops at one monolayer. On the other hand, the magnitudes of KF and n (Freundlich constants) show easy separation of metal ions from aqueous medium and indicate favorable adsorption. As seen from Table 1, n values are high enough for separation.
3.7 Kinetic modeling
With respect to the kinetic modeling of Cd(Ⅱ) and Zn(Ⅱ) biosorption, the pseudo-first order and pseudo- second order rate equation were used. The first order rate equation of Lagergren based on solid capacity is expressed as
(5)
where Qt and Qeq is sorption capacity at time t and at equilibrium, respectively; and k1, ad is paeudo-first order rate constant. After integration and applying boundary conditions, t=0 to t=t and Qt=0 to Qt=Qeq; the integrated form of Eqn.(5) becomes as
(6)
where value of k1, ad can be determined from the slope of the plot of the lg(Qeq-Qt) vs t. In most cases the first-order equation of Lagergren does not fit well during the entire adsorption period and is generally applicable in the initial 20-30 min of the sorption process[23].
Contrary to the first order model, the pseudo-second order model predicts the behavior over the whole adsorption time and is in agreement with adsorption mechanism being the rate-controlling step. The pseudo-second order rate equation is expressed as
(7)
where k2, ad is pseudo-second order rate constant. After integration and applying boundary conditions, t=0 to t=t and Qt=0 to Qt=Qeq; the integrated form of Eqn.(7) becomes
(8)
where k2, ad can be determined from the intercept of linearized plot of 1/Qt vs 1/t(Fig.7).
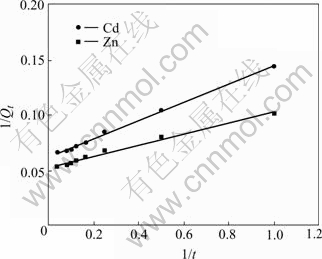
Fig.7 Linearized pseudo-second order kinetic plots of Cd (Ⅱ) and Zn (Ⅱ) ions (pH=4 for Cd (Ⅱ); pH=6 for Zn (Ⅱ), ρ0=50 mg/L, θ=26 ℃, r=120 r/min)
The parameters of pseudo-first and pseudo-second order rate kinetics are presented in Table 2. The theoretical Qeq values estimated from the first-order kinetic give significantly different values compared with the experimental values, and the correlation coefficients are also found to be slightly lower. The correlation coefficients for the second-order kinetic model and the theoretical values of Qeq also agree well with the experimental ones. Both facts suggest that the sorption of cadmium and zinc ions system is not a first-order reaction and the second-order kinetic, which relies on the assumption that biosorption may be the rate-limiting step.
Table 1 Adsorption isotherm parameters for Cd(Ⅱ) and Zn(Ⅱ) on A.niger

Table 2 First-order and second-order kinetics constants for biosorption of Cd2+ and Zn2+

4 Conclusions
A. niger is well suited for removing Cd(II) and Zn(II) from aqueous solution, due to its high capacity of biosorption. The initial pH significantly influences Cd(II) and Zn(II) uptake and maximum adsorption are observed at pH 4.0 and 6.0. The biosorption medium temperature and the agitation rate can affect the sorption capacity. The biosorption equilibriums reaches in 24 h. The removal capacities of Cd(II) and Zn(II) ions increase with increase of initial metal ions concentration. The maximum uptake capacities are 15.50 mg/g and 23.70 mg/g at initial metal ion concentrations of 75 mg/L for Cd(II) and 150 mg/L for Zn(II) ions. The biosorption process of both metal ions can be described by Langmuir isotherm. For all heavy metal ion systems studied, the rate of adsorption is found to follow the pseudo-second order kinetics.
The present study indicates that A. niger can be used as efficient living biosorbent for removal of Cd(II) and Zn(II) ions from wastewater. The biosorption system by living A. niger applied in the reality exiting treatment technologies should be more studied.
References
[1] KAEWSARN P, YU Q. Cadmium (II) removal from aqueous solutions by pre-treated biomass of marine alga Padina sp [J]. Environmental Pollution, 2001, 112: 209-213.
[2] ZHANG M K, KE Z X. Copper and zinc enrichment in different size fractions of organic matter from polluted soils [J]. Pedosphere, 2004, 14(1): 27-36.
[3] D?NMEZ G C, AKSU Z, ?ZTURK A, KUTSAL T. A comparative study on heavy metal biosorption characteristics of some algae [J]. Process Biochemistry, 1999, 34: 885-892.
[4] VOLESKY B. Detoxification of metal-bearing effluents: biosorption for the next century [J]. Hydrometallurgy, 2001, 59: 203-216.
[5] VEGLIO F, BEOLCINI F. Removal of metals by biosorption: a review [J]. Hydrometallurgy, 1997, 74(44): 301-316.
[6] PAGNANELLI F, ESPOSITO A, TORO L, VEGLIO F. Metal speciation and pH effect on Pb, Cu, Zn and Cd biosorption onto Sphaerotilus natans: Langmuir-type empirical model [J]. Water Research, 2003, 37(3): 627-633.
[7] MALIK A. Metal bioremediation through growing cells [J]. Environmental International, 2004, 30: 261-278.
[8] D?NMEZ G, AKSU Z. Bioaccumulation of copper (II) and nickel (II) by the non-adapted and adapted growing Candida spp [J]. Water Research, 2001, 35(6): 1425- 1434.
[9] SELATINA A, BAKHTI M Z, MADANI A, KERTOUS L, MANSOURI Y. Biosorption of Cd2+ from aqueous solution by a NaOH-treated bacterial dead Streptomyces rimosus biomass [J]. Hydrometallurgy, 2004, 75: 11-24.
[10] CHEN Y, LIAN B. Ability of Bacillus mucilaginosus GY03 strain to adsorb chromium ions [J]. Pedosphere, 2005, 15(2): 225-231.
[11] DURSUN A Y, USLU G, CUCI Y, AKSU Z. Bioaccumulation of cooper (Ⅱ), lead (Ⅱ) and Chromium (Ⅱ) by growing Aspergillus niger [J]. Process Biochemistry, 2003, 38: 1647-1651.
[12] AHUJA P, GUPTA R, SAXENA R K. Zn2+ biosorption by Oscillatoria anguistissima [J]. Process Biochemistry, 1999, 34: 77-85.
[13] GULNAZ O, SAYGIDEGER S, KUSVURAN E. Study of Cu(Ⅱ) biosorption by dried activated sludge: effect of physico-chemical environment and kinetics study [J]. Journal of Hazardous Materials, 2005, B120: 193-200.
[14] SHI J G, YUAN X Z , ZHANG S F, DAI F. Removal of cadmium and lead from sediment by rhamnolipid [J]. Trans Nonferrous Met Soc China, 2004, 14(1): 66-70.
[15] BALDRIAN P. Interactions of heavy metals with white-rot fungi [J]. Enzyme and Microbial Technology, 2003, 32 (1): 78-91.
[16] AL-ASHEH S, DUVNJAK Z. Adsorption of copper and chromium by Aspergillus carbonarius [J]. Biotechnology Progress, 1995, 11: 638-642.
[17] HOLAN Z R, VOLESKY B. Accumulation of Cd (Ⅱ), Pb (Ⅱ) and Ni (Ⅱ) by fungal and wood biosorbent [J]. Applied biochemistry and biotechnology, 1995, 53(2): 133-146.
[18] OMAR N B, MERROUN M L, PENALVER J M A, MUNOZ M T G. Comparative heavy metal biosorption study of brewery yeast and Myxococcus xanthus biomass [J]. Chemosphere, 1997, 35(10): 2277-2283.
[19] YAN G Y, VIRARAGHAVAN, THIRUVENKATACHARI P P. Heavy-metal removal from aqueous solution by fungus Mucor rouxii [J]. Water Research, 2003, 37: 4486-4496.
[20] ARICA M Y, BAYRAM?GLU G, YILMAZ M, BEKTAS S, GENC O. Biosorption of Hg2+, Cd2+, and Zn2+ by Ca-alginate and immobilized wood-rotting fungus Funalia trogii [J]. Journal of Hazardous Materials, 2004, B109: 191-199.
[21] SAHIN Y, OZTURK A. Biosorption of the chromium(Ⅵ) ions from aqueous solution by the bacterium Bacillus thuringiensis [J]. Process Biochemistry, 2005(40): 1895-1901.
[22] PURANIK P R, PAKNIKAR K M. Biosorption of lead, cadmium and zinc by Citrobacter strain MCM B-181: characterization studies [J]. Biotechnology Progress, 1999, 15: 228-237.
[23] ASKU Z, TEZER S. Equilibrium and kinetic modelling of biosorption of Remazol Black B by Rhizopus arrhizus in a batch system: effect of temperature [J]. Process Biochemical, 2000, 34: 431-439.
Foundation item: Project(04JJ3013) supported by the National Natural Science Foundation of Hunan, China; Project(2001AA644020) supported by the National High-Tech Research Development Program of China
Corresponding author: LIU Yun-guo; Tel: +86-731-8649208; E-mail: Liuyunguo@hun.cn
(Edited by LI Xiang-qun)