
Synthesis of TiB2 nanocrystalline powder by mechanical alloying
TANG Wen-ming(汤文明), ZHENG Zhi-xiang(郑治祥), WU Yu-cheng(吴玉程), WANG Jian-min(王建民), L? Jun(吕 珺), LIU Jun-wu(刘君武) School of Materials Science and Engineering, Hefei University of Technology, Hefei 230009, China
Received 19 January 2005; accepted 29 July 2005
Abstract: TiB2 nanocrystalline powder was synthesized by mechanical alloying of Ti-67B elemental powder. X-ray diffraction(XRD) and transmission electron microscopy(TEM) were used to study the structural evolution of the powder during ball milling. The effects of heat treatment on the structural evolution and thermal stability of the mechanically alloyed(MAed) Ti-67B powder were also discussed. During ball milling the Ti-67B powder, a solid solution of B in Ti, Ti(B) is firstly formed. When the powder is milled for 10 h, the amorphous transition of Ti(B) from the crystalline to the amorphous phase occurs. When the powder is milled for 20 h, nanocrystalline TiB2 is formed from the amorphous Ti(B). When the powder is milled for 60 h, only TiB2 is detected with grain size of 10 nm. The formation of TiB2 nanocrystalline is controlled by the gradual diffusion reaction mechanism. During heat-treatment of the MAed Ti-67B powder, the structural changes of TiB2, including grain growth and lattice ordering degree increasing may occur.
Key words: TiB2; mechanical alloying; structural evolution; nanocrystalline
1 Introduction
TiB2 has been widely used in some industrial fields owing to its high melting temperature, hardness, elastic modulus, electro-conductibility and thermal diffusivity, and excellent refractory properties and chemical inertness. Usually, TiB2 has been produced by the reduction of TiO2 and B2O3 with carbon and active metal, and the self-propagating high-temperature synthesis[1-4]. Because the reaction temperature is very high in these processes, the grain growth rate of TiB2 is rapid. So, it is very difficult to obtain pure and ultrafine-grain TiB2 powder by these processes. This has an inevitable effect on the properties of TiB2.
In 1970s, a high-energy ball milling process, named mechanical alloying(MA) was established to prepare oxide dispersion strengthened alloys, non-equilibrium alloys, intermetallics, and so on[5]. This technique has many advantages, especially, it is a simple method to synthesize the nanocrystalline materials at room temperature. Recently, MA has often been used to synthesize the metal carbides, borides and silicides, which provides a novel route to prepare fine-grained ceramic powders[6-9]. Although several studies on the synthesis of TiB2 have been reported, but the formation mechanism of TiB2 is not clear till now, mainly because of the insufficient analysis methods. And the effect of heat treatment on the structure of the mechanically alloyed TiB2 powder has not been handled. In the present study, MA technique is employed to synthesize TiB2 nanocrystalline by ball milling the Ti-67B elemental powder. The structural evolution of the element powder during ball milling is studied in details by several analysis methods, especially selected area electronic diffraction analysis. The effects of heat treatment on the structure and the thermal stability of the mechanically alloyed powder are studied, and the reaction mechanism of the formation of TiB2 via MA technique is discussed.
2 Experimental
Elemental Ti (<45 μm) and B(<45 μm) powders were firstly blended in the composition of Ti-67B (mole fraction, %). Mechanical alloying of the Ti-67B powder was carried out using a GN-2 type ball mill operated at 700 r/min to form the products TiB2. The hardened steel balls of 12 mm diameter were employed as ball milling medium. The mass ratio of ball to powder was maintained at 10∶1.
To minimize oxidation and nitriding of the blended powder, the steel vial was first evacuated with a vacuum pump, and then filled with argon with a purity of 99.99% (volume fraction). The heat treatment process was carried out by annealing the MAed Ti-67B powders at temperatures from 900 ℃ to 1 100 ℃ for 1 h. Similarly, in order to prevent oxidation and nitriding of the MAed powder in the process, the MAed powder was sealed into a silica tube. The tube was first evacuated, and then filled with the pure argon before heating.
A D/max-γB type X-ray diffractometer was used to characterize the structural evolution of the Ti-67B powder during mechanical alloying and heat treatment. According to the XRD results, the average grain sizes of the phases existed in the Ti-67B powder can be calculated[10]. A H800 type transmission electron microscope equipped with electronic diffractometer (TEM +SAED) was used to analyze the morphology and structure of the MAed powder. Before TEM and SAED analyses, the powder was firstly ground, ultrasonically dispersed in alcohol, and then glued on a copper net.
3 Results and discussion
3.1 Structural evolution during MA
The XRD patterns for the Ti-67B powder milled from 0 (as-mixed powder) to 60 h are illustrated in Fig.1. The crystallographic data, including the Bragg angle (2θ) and the main crystal face spacing of the phases (D) of the powder are listed in Table 1. After milling for 1 h, the diffraction peaks of Ti shift to lower angles, and D value of Ti(010) increases due to the dissolution of B atoms in the hcp Ti lattice. Under the equilibrium condition at room temperature, the solid solubility of B in Ti is nearly zero[11], but during MA the large volume fraction of grain boundaries present in the nanocrystalline state are expected to enhance the solid solubility[12]. Because the radius of B atom (0.098 nm) is more less than that of Ti atom (0.145 nm), the B atoms may be located into the interstices of the Ti lattice to form a solid solution of B in Ti, named Ti(B). With prolonging milling time, the continuous shift of the Ti(B) diffraction peaks is detected, suggesting the increasing of the content of B in Ti(B). Meanwhile, the initial sharp diffraction peaks of Ti are considerably broadened as a result of the refinement of the Ti(B) grains and the introduction of internal strain. In Fig.2, the grain size of Ti(B) during ball milling is described as a function of milling time. In the initial stage (<2 h), the grain size of Ti(B) decreases rapidly. In the next stage (>2 h), the grain size decreases very slowly, which varies in the range from 20 nm to 15 nm. It is suggested that the welding and fracturing frequencies are balanced gradually after milling the Ti-67B powder for 2 h and above.
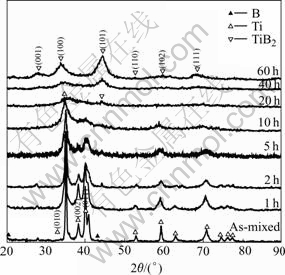
Fig.1 XRD patterns showing structural evolution of Ti-67B elemental powder milled for different times
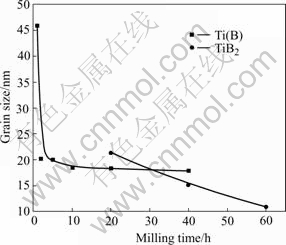
Fig.2 Grain sizes of phases of milled powder vs milling time
Table 1 XRD data of Ti-67B elemental powder
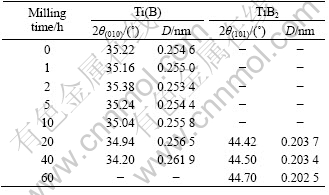
In the XRD pattern of Ti-67B powder milled for 10 h, the peaks of Ti(B) are very broad with very low intensity, suggesting that Ti(B) is mainly in amorphous state. Figs.3(a) and (b) show the morphologies and the SAED patterns of the Ti-67B powder MAed for 10 h, respectively. The average particle size of the MAed powder is about 0.1 μm. The diffraction pattern of the MAed powder is a halo, indicating an amorphous phase, which is consistent with the XRD result mentioned above. When the powder is milled for 20 h, a new phase corresponding to TiB2 appears, suggesting the weak diffraction peak of hexagonal TiB2 (101). And the TEM image of the MAed powder (Fig.3(d)) shows that the particle is spherical and its size is about 50 nm. In the SAED pattern, the diffraction speckles and the diffraction halo are both detected. Compared with the above XRD result, the diffraction speckles may indicate the hexagonal TiB2. So, when the Ti-67B powder is milled for 20 h, the TiB2 crystalline forms in the amorphous Ti(B) matrix. With prolonging the milling time from 20 h to 60 h, the intensities of the TiB2 diffraction peaks increase, and the amount of TiB2 increases. After ball milling for 60 h, no Ti(B), but TiB2 is detected. The electronic diffraction pattern of TiB2 polycrystalline also shows that the MAed powder is mainly composed of crystalline TiB2 (Fig.3(f)). The powder particles are fog-like (Fig.3(e)). During MA of the Ti-67B powder from 20 h to 60 h, the grain size of TiB2 drops roughly at a constant rate (Fig.2). When the powder is milled for 60 h, the grain size of TiB2 is about 10 nm. In summary, the structural evolution of the Ti-67B powder during ball milling can be described as: Ti+B→Ti(B)nanocrystalline→Ti(B)amorphous→TiB2 nanocrystalline.
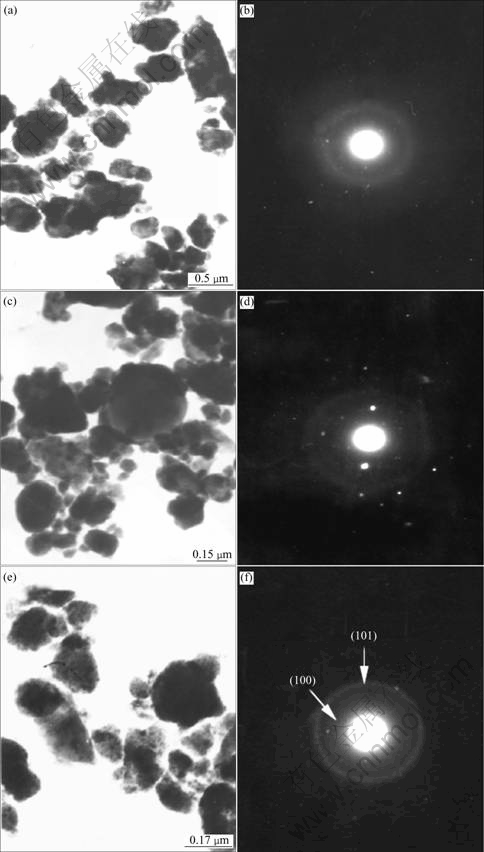
Fig.3 TEM images of Ti-67B elemental powder milled for different times: (a) 10 h; (b) SAED pattern for (a); (c) 20 h; (d) SAED pattern for (c); (e) 60 h; (f) SAED pattern for (e)
3.2 Synthesizing mechanism of TiB2
Up to date, two kinds of reaction mechanisms have been accepted in MA[13,14]: 1) The colliding balls undergo severe plastic deformation, causing the flattened particles contact with clean surface, and alloys form through gradual diffusion of thin layers, which is called the gradual diffusion reaction (GDR); 2) The alloys form through a reaction taking place within a short period with the liberation of large heat after certain milling time, which is called self-propagating high-temperature synthesis or self-sustained reaction during mechanical alloying, and it is suggested that mechanical impact plays an important role in igniting the reaction. So, the reaction mechanism is usually named mechanically induced self-propagating reaction(MSR)[13]. The MSR was often observed in highly exothermic system, such as Ni-Al, Mo-Si, Nb-C. After the MSR, the XRD diffraction peaks of the reaction products are always sharper and higher. It is due to that the reaction products are completely formed within a short time after ball milling for certain duration. And, the grain sizes of the products increase rapidly, which is attributed to the high temperature resulted from the large formation heat release of the reaction products. The MSR was also widely reported in the studies on synthesizing TiB2 by MA the Ti-67B elemental powder, which is attributed to the large formation heat release (
)=-324 kJ/mol)[1, 13-16].
In the present study, the peaks of TiB2 are broader and lower during milling the Ti-67B powder as shown in Fig.1. Only a small amount of TiB2 has been formed after milling the Ti-67B powder for 20 h. The amount of TiB2 increases gradually with milling time after milling the powder from 20 h to 60 h. After milled for 60 h, the Ti-67B powder is fully composed of TiB2. Apparently, under the employed condition, no MSR but the GDR controls the formation of TiB2. According to the GDR mechanism, a thin TiB2 layer may be firstly formed by the reaction between Ti and B during ball milling the Ti-67B powder. The diffusion of the Ti and B atoms through the TiB2 layer is necessary to sustain the reaction, till that Ti(B) in the powder is completely conversed to TiB2.
3.3 Heat treatment of MAed Ti-67B powder
After ball milled for 60 h, the Ti-67B powder was subsequently heat-treated at temperatures ranged from 900 ℃ to 1 100 ℃ for 1 h. The XRD patterns of the heat-treated powders are shown in Fig.4. More diffraction peaks of hexagonal TiB2 are detected, which are sharper and higher compared with those of TiB2 of the MAed powder. It is well known that the grain growth, the lattice deformation reduction, the internal strain elimination and the lattice ordering degree increase can all result in the shape change of the XRD patterns of the phases of the MAed powder[5]. In Table 1, the D value of TiB2 (101) is 0.202 5 nm after the Ti-67B powder is milled for 60 h. When the powder is then heat treated at 1 000 ℃ for 1 h, it is equal to 0.203 3 nm(Table 2), which approaches to that of the ideal TiB2 (101) (0.203 7 nm). The lattice ordering degree increases remarkably. In the MAed powder, the lattice deformation is high, and the lattice ordering degree is low, indicating that the powder is in non-equilibrium state. During heat treatment of the MAed powder, the lattice rearrangement of TiB2 results in the lattice deformation decreasing, and the lattice ordering degree increasing. The structure of TiB2 in the MAed powder gradually turns to be the ideal state. Simultaneously, the high lattice deformation energy acts as the driving force for the grain growth of TiB2 in the MAed powder. It promotes the grain growth of TiB2 during heat treatment. When the MAed powder is heat treated at temperature below 1 000 ℃, the grain growth rate of TiB2 is lower (Fig.5). After heat treated at 1 000 ℃ for 1 h, the grain size of TiB2 is 20 nm, which is only one times bigger than that of TiB2 of the MAed powder. When the MAed powder is heat treated at temperature above 1 000 ℃, the grain growth rate of TiB2 is higher. After heat-treated at 1 100 ℃ for 1 h, the grain size of TiB2 is 41.2 nm, which is four times bigger than that of TiB2 of the MAed powder, and two times bigger than that of TiB2 heat treated at 1 000 ℃ for 1 h.
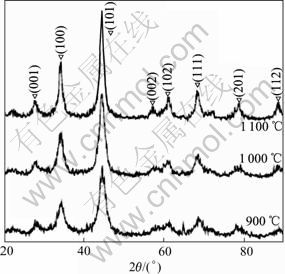
Fig.4 XRD patterns of MAed TiB2 powder after heat-treatment at various temperatures
Table 2 XRD data of Ti-67B powder after heat treatment
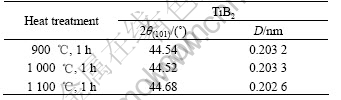
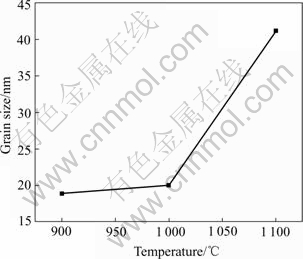
Fig.5 Grain size of TiB2 vs heat-treating temperature after Ti-67B powder milled for 60 h
4 Conclusions
1) TiB2 nanocrystalline powder can be synthesized by ball milling the Ti-67B elemental powder. The structural evolution of the Ti-67B powder during ball milling is: Ti+B→Ti(B)nanocrystalline→Ti(B)amorphous→ TiB2 nanocrystalline. The formation of TiB2 is controlled by the GDR mechanism.
2) During ball milling the Ti-67B powder, Ti(B) is firstly formed. After ball milling only for 2 h, the grain size of Ti(B) drops steeply to 20.2 nm. With prolonging milling time, it decreases slowly, which varies between 15 nm to 20 nm. After the powder milled for 10 h, an amorphous Ti(B) phase is formed. After the powder milled for 20 h, a new phase corresponding to hexagonal TiB2 appears. The amount of TiB2 in the MAed powder increases with milling time. After the powder milled for 60 h, the amorphous Ti(B) has entirely been transferred into TiB2. The grain size of TiB2 decreases with the increasing of the milling time, which is about 10 nm after milling for 60 h.
3) During ball milling the Ti-67B powder, the particle shape turns to be spherical; the particle size decreases with increasing the milling time. When the Ti-67B powder is milled for 60 h, the powder particles are fog-like investigated by TEM.
4) During heat-treating the Ti-67B powder milled for 60 h, the structural change of TiB2, including grain growth and lattice ordering degree increase may occur. When the MAed powder is heat-treated at temperature below 1 000 ℃, the grain size of TiB2 increases slowly, the MAed powder is thermally stable. When the MAed powder is heat treated at temperatures above 1 000 ℃, the TiB2 grain grows fast. The thermal stability of the MAed powder decreases.
References
[1] Huang Y, Lee J K. Preparation of TiB2 powders by mechanical alloying [J]. Mater Letts, 2002, 54(1): 1-7.
[2] Lü L, Lai m o, Su y, TEO H L, FENG J L. In situ TiB2 reinforced Al alloy composites [J]. Scripta Mater, 2001, 45(9): 1017-1023.
[3] Radev D D, Marinov M. Properties of titanium and zirconium diborides obtained by self-propagated high-temperature synthesis [J]. J Alloys Compd, 1996, 244(1-2): 48-51.
[4] Xiang X, Qing Y. Progress in TiB2 and its composites [J]. J Ceram, 1999, 20(2): 112-117.(in Chinese)
[5] Murty B S, Ranganathan S. Novel materials synthesis by mechanical alloying/milling [J]. Inter Mater Rev, 1998, 43(1): 101-141.
[6] Eskandarany M S, Konno T J, Sumiyama K, SUZUKI K. Formation of TiC and SiC refractory compounds by mechanical alloying[J]. J Japan Soc Powder Powder Metall, 1996, 43(2): 579-583.
[7] Gupta R, Gupta A. Mechanical alloying of Fe-B powders [J]. Mater Sci Eng A, 2001, 304/306(1): 442-445.
[8] LIU L, CUI K. Mechanical alloying of refractory metal-silicon systems [J]. J Mater Proc Technol, 2003, 138(3): 394-398.
[9] YEN B K, Aizawa T, Kihara J. Synthesis and formation mechanisms of molybdenum silicides by mechanical alloying [J]. Mater Sci Eng A, 1997, 239/240(1): 515-521.
[10] Tang W M, Zheng Z X, Wu Y C, TANG H J, DONG J L. Structural evolution of the Fe75Al25 binary system during mechanical alloying and subsequent heat treatment [J]. Trans Mater Heat Treat, 2004, 25(3): 18-21.(in Chinese)
[11] Lu L, Lai M O, Wang H Y. Synthesis of titanium diboride TiB2 and Ti-Al-B metal matrix composites [J]. J Mater Sci, 2000, 35(1): 241-248.
[12] WANG Er-de, Liu J L, Liu Z Y. The research progression on the kinetics of extension solid solubility induced by mechanical alloying [J]. Powder Metallurgy Technology, 2002, 20(2): 109-112.(in Chinese)
[13] Ye L L, Liu Z G, Quan M X, GUO J T. Different reaction mechanisms during mechanical alloying Ti50C50 and Ti33B67 [J]. J Appl Phys, 1996, 80(3): 1910-1912.
[14] Takacs L. Self-sustaining reaction induced by ball milling [J]. Prog Mater Sci, 2002, 47(4): 355-414.
[15] Calos N J, Forrester J S, Schafer G B. The mechanisms of combustion and continuous reactions during mechanical alloying [J]. J Solid State Chem, 2001, 158(2): 268-278.
[16] Surganarayana C. Mechanical alloying and milling [J]. Prog Mater Sci, 2001, 46(1-2): 1-184.
Corresponding author: TANG Wen-ming; Tel: +86-551-2901372; Fax: +86-551-2901362; E-mail: wmtang69@126.com
(Edited by YUAN Sai-qian)