
Effect of graphite content on wear property of graphite/Al2O3/Mg-9Al-1Zn-0.8Ce composites
ZHANG Mei-juan(张美娟)1, YANG Xiao-hong(杨晓红)1, LIU Yong-bing(刘勇兵)1,
CAO Zhan-yi(曹占义)1, CHENG Li-ren(程丽任)1, PEI Ya-li(裴亚利)2
1. Key Laboratory of Automobile Material of Ministry of Education, Jilin University, Changchun 130025, China;
2. Beijing Institute of Aerospace Testing Technology, Beijing 100074, China
Received 26 November 2008; accepted 1 July 2009
Abstract: Using squeeze-infiltration technique, Mg-9Al-1Zn-0.8Ce composite reinforced by graphite particles and Al2O3 short fibers was fabricated. The reinforcing phases combined closely with the matrix and no agglomeration was observed. The microstructure, hardness and wear property of the composites with the graphite content of 5%, 10%, 15% and 20% were investigated, respectively. The results reveal that Ce tends to enrich around the boundaries of graphite particles and Al2O3 short fibers, and forms Al3Ce phase. When the graphite content increases to 20%, the grain size becomes small. Moreover, with increasing the graphite content, the microhardness of the composites decreases but the wear resistance increases. The graphite which works as lubricant during dry sliding process decreases the wear loss. At low load, the wear mechanism of the composite is mainly abrasive wear and oxidation wear; at high load, except that the composite with 20% graphite is still with abrasive wear and oxidation wear, the wear mechanism of other composites changes to delamination wear.
Key words: magnesium matrix composite; Ce; graphite; wear resistance
1 Introduction
Among the light metals, Mg and its alloys have high potential in automotive and aerospace industries due to their low densities and high specific strength[1]. However, its poor resistance to wear and corrosion is a serious impediment preventing them from being used as widely as Al alloys[2-4]. Recently, some researches have been done on magnesium matrix composites containing particles, fibers or rare earth elements, which exhibit higher properties than single alloy[5-7]. KUMAR et al[8] investigated the Mg-SiC composites and discussed the formation of MgO during the early stage of abrasive wear. CONTRERAS et al[9] investigated the Al-Mg/TiC composites and found the aluminum carbide forming at the interface. Most researches use hard particles like SiC or TiC particles as reinforcing phase; however, investigation on self-lubricating magnesium matrix composites is less. Especially, using graphite as reinforcing phase which works as lubricant during sliding is absent[10-13]. In the present work, to make use of the combination of lubricating graphite and hard Al2O3 short fiber, a set of graphite and Al2O3 reinforced Mg-9Al-1Zn-0.8Ce magnesium matrix composites were fabricated. The effects of graphite content on the microstructure, hardness and wear property were investigated along with the wear mechanism during the dry sliding process.
2 Experimental
Mg-9Al-1Zn-0.8Ce alloy was chosen as matrix alloy. The short fibers with a diameter of 8-12 μm and a length of 300-700 μm contained 98.9% Al2O3. The size of graphite particles was 100-150 μm. The content of Al2O3 short fibers kept constant at 8% (volume fraction) and volume fraction of the graphite were 5%, 10%, 15% and 20%, respectively.
The process of fabricating the composites was composed of two steps. First, graphite and Al2O3 short fibers were used to prepare the preform. Second, the molten Mg-9Al-1Zn-0.8Ce alloy was added into the preform by squeeze-infiltration technique. The pouring temperature was 680 ℃, the applied pressure was 55 MPa and was maintained for 60 s. The worn surface was analyzed by JSM-6700F scanning electron microscope (SEM) with an energy-dispersive X-ray spectrometer (EDS). The pin-on-disc type apparatus MM2000 was used to test dry sliding wear behavior. In this system, the test specimen was d6 mm×12 mm in dimension and the disc (d70 mm) was made of GCr15 steel with the hardness of HRC55. The disc was kept rotating at a constant speed of 0.785 m/s, and slid a distance of 376.8 m. The density of sample was measured using the standard Archimedes method with distillated water. Mass changes before and after wear tests were used to calculate the volume wear loss.
3 Results and discussion
3.1 Influence of graphite and Al2O3 additions on microstructure of composites
The optical microstructures of the composites with different graphite (Gr) contents are shown in Fig.1. It is revealed that the graphite particles and Al2O3 short fibers combine closely with the matrix, and no agglomeration and casting defect are observed evidently. The graphite takes the form of flake and Al2O3 short fibers appear in round and needle shape. Some rod-like phases are found around the boundaries of graphite and Al2O3 short fibers, as shown in Fig.1(e). It is Al3Ce phase confirmed by XRD analysis due to the electronegativity for the reason that Ce is in preference to form Al3Ce phase with Al. As the graphite content increases from 5% to 15%, the grain size changes little; but when the graphite content increases to 20%, the grains become uniform and the particle size becomes small. This indicates that in solidification process more graphite particles which divide the liquid can provide more nucleate regions and finally lead to smaller grain size.
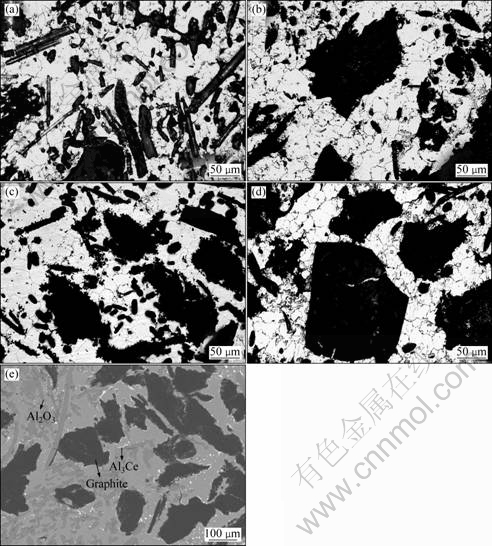
Fig.1 Optical microstructures of composites: (a) w(Gr)=5%; (b) w(Gr)=10%; (c) w(Gr)=15%; (d) w(Gr)=20%; (e) Backscattering image of composite with 20% graphite
3.2 Influence of graphite content on wear resistance of composites
The microhardness of the composite matrix away from the graphite and Al2O3 short fibers was measured.
The microhardnesses of the composites containing 5%, 10%, 15% and 20% graphite are HV110.12, HV106.68, HV90.03 and HV86.17, respectively. The microhardness decreases with increasing the graphite content. Although the presence of Al3Ce phase and the effect of fine grain strengthening can enhance the strength of the matrix, the graphite particle is still a soft phase which decreases the strength of the matrix and affects the microhardness.
The variation of wear loss with load of the composites of different graphite contents is shown in Fig.2. It is revealed that the wear loss goes up with increasing load for all composites. At 20 N and 60 N, the composites with 5%, 10% and 15% graphite have similar wear losses. At high load their wear losses show different phenomena and the wear loss of the composite with 5% graphite increases sharply. The composite with 20% graphite keeps the best wear resistance. Compared with the composite with 5% graphite, the wear loss of the composite with 20% graphite descends by 35% and 40% at 20 N and 180 N, respectively. This implies that besides the presence of Al2O3 short fibers can increase the strength of the matrix and bear load, graphite added delays the changes from mild wear to severe wear and works effectively at high load[14].
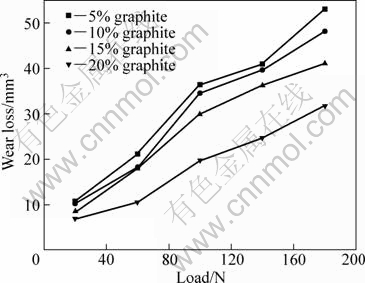
Fig.2 Variations of wear loss with load of composites
3.3 Wear mechanism of composites
Fig.3 displays the SEM morphologies of the worn surface and EDS analysis results of the composites with 5% graphite at 20 N and 60 N. At 20 N the worn surface which has no evident grooves is made up of graphite and oxide film. It is proved by EDS analysis that the peak value of oxygen element is high. It is known that at low load the wear mechanism of Mg alloy is mainly abrasive wear and oxidation wear, so the worn surface presents grooves and powder debris[15]. But, there are no clear grooves on the worn surface of the composites at 20 N. It is only covered with oxide film. This is because that at the beginning of the dry sliding process, for the reason of abrasive wear, the worn surface has slight grooves and debris. While sliding the graphite smears on the worn surface, and its lubricating effect decreases the influence of abrasive wear, so the grooves become less. At the same time, more friction heat concentrates on the worn surface, so the worn surface is easier to be oxidized. But, the test load is not high enough to peel off the oxidative alloys as they form, so the oxidative alloys form continuous oxide area and finally the oxidative alloys mixed with graphite form the oxide film. Other composites also show the similar phenomenon at 20 N, and they display similar wear loss at 20 N, as shown in Fig.2.
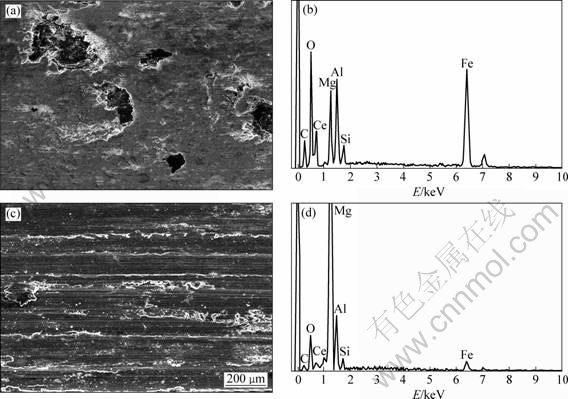
Fig.3 SEM morphologies of worn surface and EDS analysis results of composites with 5% graphite: (a), (b) At 20 N; (c), (d) At 60 N
After increasing the test load to 60 N, the worn surface of the composite with 5% graphite shows many parallel grooves and debris, which indicates the wear mechanism is abrasive wear and oxidation wear, as shown in Fig.3(c). At 60 N the test load is high enough to peel off the oxidative alloys, so it is hard to form large continuous area of oxide film and the oxidative alloys take the form of powder debris or extrudes on the edge of grooves. The EDS analysis result also confirms that the content of oxygen element on the worn surface is low.
The SEM morphology of the worn surface of the composite with 5% graphite at 180 N is shown in Fig.4(a). With increasing the load the worn surface deteriorates. The grooves become discontinuous; and some metal chips break off and form stripping hole. But, the worn surface of the composite with 20% graphite (Fig.4(b)) is nearly intact. The graphite particles which still act as lubricant can be also seen on the worn surface. During the dry sliding process they are extruded from the worn surface and smear on the surface, then form graphite film, which improves the wear resistance of the composite at high load[16-17].
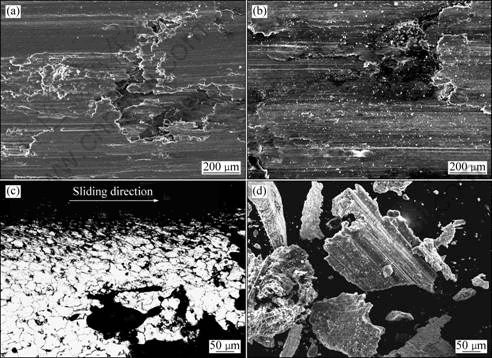
Fig.4 SEM morphologies of worn surface of composites at 180 N: (a) w(Gr)=5%; (b) w(Gr)=20%; (c) Optical photograph of cross-sectional profile; (d) SEM morphology of debris
The optical photograph of cross-sectional profile shows (Fig.4(c)) that the plastic deformation occurs on the surface layer. The grains next to the surface are elongated along the contrary side of the sliding direction, which results in the cracks initiating in sub-surface. At the same time, the plastic deformation makes some Al2O3 short fibers break up which can no longer bear load and decrease the strength of the composites. So, the worn surface is easier to be peeled off and gives rise to wear debris in flakes. The composite with 5% graphite has larger strip debris compared with the composite with 20% graphite, as shown in Fig.4(d). Because small debris influences wear process less, large debris deteriorates the contact surface. So, large debris speeds up the changes from mild wear to severe wear.
Although Al3Ce phase which has better thermal stability than β-Mg17Al12 can prevent the slide of grain boundaries, the plastic deformation inevitably takes place at high load. Adding more graphite can provide better lubricating effect on the worn surface and decrease the influence of plastic deformation in the sub-surface. So, the composite with 20% graphite can still keep a low wear loss at 180 N. At high load, except that the composite with 20% graphite is still with abrasive wear and oxidation wear, the wear mechanism of others changes to delamination wear.
4 Conclusions
1) Graphite particles and Al2O3 short fibers reinforced Mg-9Al-1Zn-0.8Ce composites can be fabricated by squeeze-infiltration technique. When the graphite content increases to 20%, the grain size becomes small.
2) The microhardness increases with the decrease of graphite content. The wear resistance of the composites increases with increasing the graphite content.
3) At low load, the wear mechanism of the composites is mainly abrasive wear and oxidation wear; at high load, except that the wear mechanism of the composite with 20% graphite is still abrasive wear and oxidation wear, the wear mechanism of others changes to delamination wear.
Acknowledgments
The authors thank D. T. CAO and D. T. AN for discussion and beneficial suggestion.
References
[1] MORDIKE B L, EBERT T. Magnesium: Properties- applications-potential [J]. Materials Science and Engineering A, 2001, 302(1): 37-45.
[2] WEI Xiao-wei, HUANG Qing-min. Elevated temperature properties of Mg-12Li-Al-MgO composites [J]. Transactions of Nonferrous Metals Society of China,2006, 16(2): 432-437.
[3] RODRIGUEZ J, MARTIN A, LLORCA J. Modeling the effect of temperature on the wear resistance of metals reinforced with ceramic particles [J]. Acta Materialia, 2000, 48(4): 993-1003.
[4] RAJAN T P D, PRABHU K N , PILLAI R M, PAI B C. Solidification and casting/mould interfacial heat transfer characteristics of aluminum matrix composites [J]. Composites Science and Technology, 2007, 67(1): 70-78.
[5] FU Gao-feng, ZHANG Jing-xin, LIU Ji, WANG Zhao-fei. Wear behavior of Al-Si alloy matrix composites reinforced by γ-Al2O3 decomposed from AACH [J]. Transactions of Nonferrous Metals Society of China,2006, 16(5): 1159-1162.
[6] HU Qiao-dan, LUO Peng, YAN You-wei. Microstructures, densification and mechanical properties of TiC-Al2O3-Al composite by field-activated combustion synthesis [J]. Materials Science and Engineering A, 2008, 486(1/2): 215-221.
[7] ZHANG Guo-ying, ZHANG Hui, GAO Ming, WEI Dan. Mechanism of effects of rare earths on microstructure and properties at elevated temperature of AZ91 magnesium alloy [J]. Journal of Rare Earths, 2007, 25(3): 348-351.
[8] KUMAR B V M, BASU B, MURTHY V S R, GUPTA M. The role of tribochemistry on fretting wear of Mg-SiC particulate composites [J]. Composites Part A: Applied Science and Manufacturing, 2005, 36(1): 13-23.
[9] CONTRERAS A, CHAVEZ C A, FLORES O, PEREZ R. Structural, morphological and interfacial characterization of Al-Mg/TiC composites [J]. Materials Characterization, 2007, 58(8/9): 685-693.
[10] LIM C Y H, LIM S C, GUPTA M. Wear behaviour of SiCp-reinforced magnesium matrix composites [J]. Wear, 2003, 255(1/6): 629-637.
[11] GU X Y, SUN D Q, LIU L. Transient liquid phase bonding of TiC reinforced magnesium metal matrix composites (TiCp/AZ91D) using aluminum interlayer [J]. Materials Science and Engineering A, 2008, 487(1/2): 86-92.
[12] WANG Ji-jie, GUO Jin-hua, CHEN Li-qing. TiC/AZ91D composites fabricated by in situ reactive infiltration process and its tensile deformation [J]. Transactions of Nonferrous Metals Society of China, 2006, 16(4): 892-896.
[13] LIN C B, CHANG R J, WENG W P. A study on process and tribological behavior of Al alloy/Gr.(p) composite [J]. Wear, 1998, 217(2): 167-174.
[14] CHEN H, ALPAS A T. Sliding wear map for the magnesium alloy Mg-9Al-0.9Zn (AZ91) [J]. Wear, 2000, 246(1/2): 106-116.
[15] AN J, LI R G, LU Y, CHEN C M, XU Y, CHEN X, WANG L M. Dry sliding wear behavior of magnesium alloys [J]. Wear, 2008, 265(1/2): 97-104.
[16] LIU Yong-bing, ROHATGI P K, RAY S. Tribological characteristics of aluminum-50% graphite composite [J]. Metallurgical Transactions A, 1993, 24(1): 151-159.
[17] WALKER J C, RAINFROTH W M, JONES H. Lubricated sliding wear behaviour of aluminium alloy composites [J]. Wear, 2005, 259(1/6): 577-589.
Foundation item: Project(2006BAE04B04-1) supported by the Special Task Document of National Science and Technology Program of China; Project(20060308) supported by Science and Technology Development Program of Jilin Province, China; Project supported by “985 Project” of Jilin University, China
Corresponding author: LIU Yong-bing; Tel: +86-431-85095874; E-mail: ybingliu@jlu.edu.cn
DOI: 10.1016/S1003-6326(09)60122-9
(Edited by YANG Bing)