J. Cent. South Univ. Technol. (2007)04-0520-04
DOI: 10.1007/s11771-007-0101-5
Preparation and enantiosorption of L-aspartic acid pillared hydrotalcites
PENG Xia-hui(彭霞辉)1,2, HUANG Ke-long(黄可龙)2
(1. Department of Chemistry and Environment Science, Changsha University, Changsha 410003, China;
2. School of Chemistry and Chemical Engineering, Central South University, Changsha 410083, China)
______________________________________________________________________
Abstract:L-aspartic acid (Asp) pillared hydrotalcites were prepared by direct reaction of the L-Asp anion with layered double hydroxides (LDHs). The obtained samples were characterized by X-ray diffractometry (XRD), Fourier transform infrared (FTIR), and thermogravimetric and differential thermal analysis (TG/DTA). The results show that the initial interlayer carbonate ions can be completely replaced by the L-Asp anion under the controlled conditions. The pillared hydrotalcites have a crystallized supramolecular structure and thermal stability. The L-Asp pillared LDHs were used in the enantiosorption of enantiopure phenylalanine (Phe), the results suggest that L-Asp pillared LDHs exhibit an excellent enantiosorption capability for D-Phe, and the adsorption isotherm fits Freundlich equation.
Key words: L-aspartic acid; hydrotalcites; preparation; enantiosorption; phenylalanine
______________________________________________________________________
1 Introduction
Among layered compounds, layered double hydroxides (LDHs) and organo-LDHs represent a kind of the most technologically promising materials because of their easy preparation and broad uses as adsorbents[1]. The LDHs can be described as layered compounds of brucite-like structure (Mg(OH)2), with positively charged layers due to a partial substitution of divalent M2+ metal ions by trivalent M3+ metal ions. Between the layers there are counter anions to balance the electrical charge and coordinating water molecules. LDHs are commonly represented by the general formula: [M2+1-xM3+x (OH)2]x+(An-x/n)?mH2O, where M2+ and M3+ can be any divalent and trivalent metal ions with ionic radius similar to Mg2+, x is the molar ratio of M3+ to (M2++M3+) and An- is the interlamellar charge-compensating anion. Certain bulky organic or anions may be used as pillaring agents via anion exchange. LDHs are used for preparing pillared layer materials, most frequently organo-LDHs[2]. It is especially so, if the pillars are chiral, these quasi two-dimensional channel systems are not only well defined structures with huge pore openings, but the optically active pillars create a chiral environment. Materials like these may easily find use in chiral separation just as in enantioselective adsorption[3]. In view of fundamental research it is an added bonus if the same compound can be used for cationic as well as anionic intercalation. L-aspartic acid(Asp) can be the compound of choice, which is a key amino acid to form protein[4-5].
The preparation and characterization of L-Asp pillared clay materials were reported in this work. The host substance was Mg/Al hydrotalcite for anionic pillaring, the guest molecule was L-Asp. The enantiosorption of enantiopure phenylalanine(Phe) with L-Asp pillared materials and LDHs was investigated using batch methods, D-Phe and its L-isomer were served as a model in this study.
2 Experimental
2.1 Preparation of L-Asp pillared LDHs
A Mg/Al-LDH (n(Mg)/n(Al)=2.11:1) using carbonate as the interlayer anion was synthesized by a modified coprecipitation method as described in Ref.[6].
L-Asp pillared LDH was prepared by adding L-Asp (0.066 mol) to a suspension of the above LDH (0.008 mol) in 100 mL distilled water. The suspension slowly dissolved with effervescence and a clear solution was obtained. This solution was added dropwise to an alkaline solution (0.05 mol NaOH) dissolved in 100 mL decarbonated water), maintaining pH value above 9, followed by refluxing for 6 h. The resulting solid was recovered by filtration, washed, and dried at 75 ℃ for 24 h, giving the L-Asp pillared LDH[7].
2.2 Characterization
X-ray diffraction (XRD) measurement was performed on a Rigaku Rint 6000 powder X-ray diffract- ometer, using Cu Kα radiation at 30 mA, 40 kV. Fourier transform infrared (FTIR) spectra were obtained using a NICOLET AVATAR 360 FTIR spectrophotometer by the standard KBr disk method. Thermogravimetric analysis and differential thermal analysis (TG/DTA) were carried out on PCT-1A thermal analysis system produced locally in flowing air at a heating rate of 10 ℃/min. The samples were dried at 100 ℃ for 24 h prior to analysis.
2.3 Enantiosorption experiments
Enantiopure Phe solutions were prepared by dissolving enantiopure Phe in deionized water. The initial pH value of enantiopure Phe solutions was about 5.2. A series of enantiopure Phe solutions with desired pH values were obtained by adjusting with dilute HCl or NaOH solutions. Phe enantiosorption experiments were carried out using a batch method. The effects of contact time, kind of sorbents and solution pH on Phe enantisorption were investigated, respectively. 100 mg of L-Asp pillared LDH or carbonate-LDH was weighed into 50 mL corex glass tube with plug, which was then filled with a 50 mL enantiopure Phe solution (concentration ranging from 5 to 180 mg/L). The tubes were placed in a water bath at 25 ℃ and gently shaken for specific time or until sorption equilibrium. The initial (ρ0, mg/L) and the equilibrium (ρe, mg/L) concentrations were analyzed by UV-2101 spectroscope (SHIMADAZU, Japan), the absorbance was measured at 250 nm. The amount of adsorbed Phe (qe) was determined from the difference between ρ0 and ρe:
qe=(ρ0-ρe)×V/W (1)
where V and W are the volume (L) of enantiopure Phe solution and the sorbent quality (g) in adsorptive system, respectively. The sorption isotherms were obtained by plotting the amount of D (L)-Phe adsorbed qe vs ρe in the equilibrium solution[8].
3 Results and discussion
3.1 Characterization of materials
The XRD patterns(Fig.1) for the Mg/Al carbonate-LDH precursor show the characteristic reflections corresponding to a well-crystallized layered phase with an interlayer spacing of 0.76 nm (Table 1). The powder XRD patterns of the material are of typical layered material, showing the basal peak and higher order reflections at low angle. Intercalation of the L-Asp anion can be clearly seen in each case by virtue of the increase in basal spacing compared with that in the carbonate precursor. The thickness of the brucite-like layer of LDH is 0.48 nm, the gallery height (0.79 nm) for the L-Asp pillared material (Table 1). These data suggest a monolayer arrangement for the intercalated L-Asp oriented perpendicular to the LDH layers[9-11].
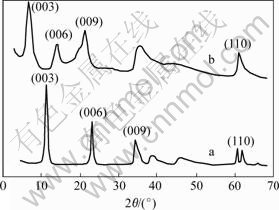
Fig.1 XRD patterns for LDH (a) and L-Asp-LDH (b)
Table 1 XRD data of diffraction peaks of LDH and L-Asp-LDH
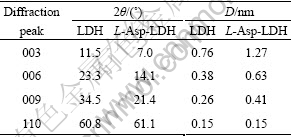
The LDH and intercalated L-Asp materials were further confirmed by examination of the FTIR spectra shown in Fig.2. The spectrum of the LDH-carbonate precursor has an intense absorption at 1 361 cm-1. The weak absorption peaks of the C—H stretch are observed in region from 2 950 to 3 200 cm-1, and the strong absorption peak of COO- antisymmetric stretch at 1 400 cm-1 is observed. The peaks at 1 596 and 1 640 cm-1 correspond to the bending vibration of N—H group and O—H group, respectively. In the low- frequency region, the absorption peaks of the spectra that correspond to the lattice vibration modes are attributed to M—O (840 and 650 cm-1) and O—M—O (430 cm-1) vibrations[12-13]. These peaks demonstrated that L-Asp was intercalated into the LDH. Thermogravimetric analysis results for the LDH show an initial distinct reduction in mass at 70-240 ℃ (about 14%, mass fraction) arising from loss of surface adsorbed and interlayer water. A major mass loss is observed starting at 260 ℃ and continuing up to 550 ℃, resulting from simultaneous dehydroxylation of the inorganic layers and decomposition of intercalated carbonate anions (about 25%, mass fraction) (Fig.3). The corresponding DTA trace for the LDH shows two endotherms centered at 230 ℃ and 410 ℃ associated with these processes.
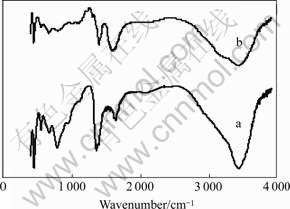
Fig.2 FTIR spectra for LDH (a) and L-Asp-LDH(b)
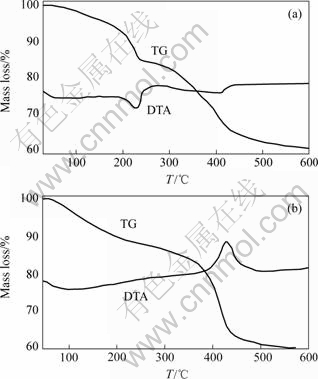
Fig.3 TG/DTA curves for LFHs(a) and L-Asp-LDHs(b)
In contrast, the intercalated LDHs underwent a mass loss between ambient temperature and 250 ℃ (about 18%, mass fraction), resulting from loss of surface adsorbed and interlayer water. The onset of a rapid and major mass loss (about 30%, mass fraction) at 320-500 ℃ reveal that dehydroxylation and decomposition of the organics occur in an exothermic process. The corresponding DTA trace shows exotherm at about 450 ℃ associated with combustion of the organic component.
3.2 Effect of time on adsorption
The effect of adsorption time on D-Phe by pillared LDH is shown in Fig.4, the amount of Phe adsorbed increases rapidly within initial 6 h, followed by a slow increase in the following 21 h. In order to ensure a complete sorption equilibrium, the adsorption time is chosen to be 27 h.
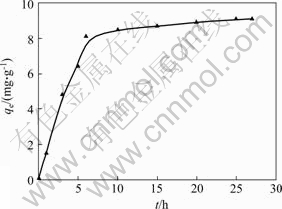
Fig.4 Effect of time on adsorption at D-Phe concentration of 100 mg/mL
3.3 Adsorption isotherms and enantiosorption mechanisms
The effect of different adsorbents on the adsorption of D(L)-Phe is shown in Fig.5. It is evident that adsorption capacities of LDHs are larger than those of pillared LDHs(PLDHs). These results suggest that LDHs may only be used as adsorbent to Phe without any selectivity. The main mechanism for Phe adsorption by LDHs is exchanged between Phe anions and previously adsorbed interlayer CO32- ions, though surface adsorption is presumed to be also involved. Large affinities of LDHs for CO32- prevent the exchange to a great extent, resulting in quantitative adsorption of Phe. The smaller adsorption capacity of pillared LDHs can be attributed to the peculiar property of structural construction, L-Asp as chiral ligand maybe forms binary complex with Mg2+ of pillared LDHs, which plays an important role in enantiosorption. Chiral recognition occurs by D-Phe forming ternary complexes with the binary complex. This suggests that pillared LDHs are very efficient enantiosorbents for D-Phe and particularly suitable to separate racemic Phe. Consequently, the use of L-Asp pillared LDHs as enantiosorbent was demonstrated to be an effective chiral separation method[14-15]. Isotherms for D-Phe adsorption by L-Asp-LDHs was modeled by two commonly used isotherm equations, Freundlich (Eqn.2) and Langmuir (Eqn.3).
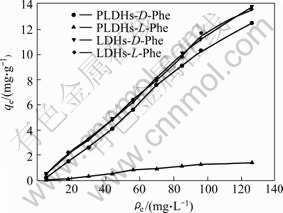
Fig.5 Effect of kind of adsorbent on adsorption of D(L)-Phe
(2)
(3)
where qm (mg/g) is the theoretical maximum monolayer sorption capacity, and KF, 1/n and KL are empirical constants[1].
Data for D-Phe adsorption by pillared LDHs were fitted better by the Freundlich equation than by the Langmuir equation based on the correction coefficient R, which is above 0.99 for Freundlich equation, and the corresponding fitting equation is:
(4)
The values of KF and 1/n are 0.030 3 and 1.285 7, respectively. From Fig.5, it is found that pillared LDHs exhibit a weak adsorption action on L-Phe. Data for L-Phe adsorption by pillared LDHs are not fitted better by both the Freundlich equation and the Langmuir equation.
3.4 Effect of pH value of solution
The effect of pH value on D-Phe adsorption is shown in Fig.6, indicating that qe increases with the rise of pH value of solution, arrives the maximum at the pH value of 5.5, and then decreases. It may be concluded that the effect of initial pH over a pH range (3.0–6.0) on qe is minimal probably due to pK=5.27 of Phe, Phe can only keep free molecular state at pH value of 5.5, which maybe favors the chiral recognition.
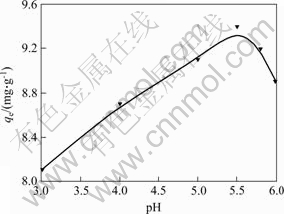
Fig.6 Effect of pH on sorption Conditions: the concentration of D-Phe in 100 mg/mL
4 Conclusions
1) L-Asp pillared hydrotalcites are assembled and intercalated by direct reaction of the L-Asp with Mg/Al-LDHs precursor. The samples obtained are characterized by XRD, FTIR, and TG/DTA. The results show that the initial interlayer CO32- can be completely replaced by the L-Asp anion under the controlled conditions. The pillared-hydrotalcites are of crystallized supramolecular structure and thermal stability.
2) The L-Asp pillared LDHs are used in the process of enantiosorption of enantiopure Phe. The results show that L-Asp–LDHs exhibit excellent enantiosorption capability for D-Phe, and adsorption isotherm fits Freundlich equation.
References
[1] YOU You-wen, ZHAO Hong-ting, VANVE G F. Adsorption of dicamba (3, 6-dichloro-2-methoxy benzoic acid) in aqueous solution by calcined-layered double hydroxide[J]. Applied Clay Science, 2002, 21: 217-226.
[2] GARDNER E A, YUN S K, KWON T, et al. Layered double hydroxides pillared by macropolyoxometalates[J]. Applied Clay Science, 1998, 13: 479-494.
[3] AISAWA S, TAKAHASHI S, OGASAWARA W. Direct intercalation of amino acids into layered double hydroxides by coprecipitation[J]. Journal of Solid State Chemistry, 2001, 162: 52-62.
[4] ZHU Zhi-liang, GAO Jun-ying, LI Feng-ting, et al. The study of adsorption of L-aspartic acid on silver sol by surface-enhanced Raman scattering[J]. Spectroscopy and Spectral Analysis, 2004, 24(1): 68-70. (in Chinese)
[5] LIU Yi, QIAO Shao-song, LI Jia-you. Microwave assisted rapid racemization of L-Phenylalanine[J]. Chemical Industry and Engineering Progress, 2005, 24 (8): 893-900. (in Chinese)
[6] ZHAO Yun, JIAO Qing-ze, EVANS D G, et al. Control of particle size of layered double hydroxides using non-equilibrium aging conditions[J]. Chinese Journal of Inorganic Chemistry, 2001, 11(6): 830-834. (in Chinese)
[7] CAIT E, PHEE M, WOOLFSON D N. Engineered and designed peptide-based fibrous biomaterials[J]. Current Opinion in Solid State and Materials Science, 2004 (8): 141-149.
[8] MAO Xu-zhua, YANG ping, XIE Li-mei, et al. Sorption of an anionic dye by uncalcined and calcined layered double hydroxides: A case study[J]. Journal of Hazardous Materials B, 2005, 120: 163-171.
[9] INACIO J, TAVIOT-GUE?HO C, FORANO C. Adsorption of MCPA pesticide by MgAl-layered double hydroxides[J]. Applied Clay Science, 2001, 18: 255-264.
[10] REN Ling-ling, HE Jing, EVANS D G, et al. Intercalation and assembly of supramolecular materials of glutamic acid pillared layered double hydroxides[J]. Chemical Journal of Chinese Universities, 2003, 24(1): 169-173. (in Chinese)
[11] REN Ling-ling, HE Jing, DUAN Xue. The intercalation and assembly of anion pillared materials[J]. Chemistry, 2001, 11: 686-691.
[12] FUNDABLE A′, PA′LINKO′ I, KIRICSIA I. Amino acids, precursors for cationic and anionic intercalation synthesis and characterization of amino acid pillared materials[J]. Journal of Molecular Structure, 1999, 482/483: 33-37.
[13] LI Dian-qing,FENG Tao,EVANS D G,et al. Assembly and structural characteristics of organic anion-pillared hydrotalcites[J]. The Chinese Journal of Process Engineering, 2002, 2(4): 355-360.
[14] YOU Qi-dong, LIN Guo-qiang. Chiral Drugs-Research and Application[M]. Beijing: Chemical Industry Press, 2004: 25-175.
[15] JIAO Fei-peng, HUANG Ke-long, PENG Xia-hui. Preparation and application of optical pure tartaric acid pillared Mg-Al layered double hydroxides[J]. The Chinese Journal of Nonferrous Metals, 2005, 16(6): 975-979.
______________________
Foundation item: Project(20376085) supported by the National Natural Science Foundation of China; Project(05C053) supported by Education Department of Hunan Province, China
Received date: 2006-10-28; Accepted date: 2007-01-07
Corresponding author: PENG Xia-hui, Professor, Doctorial candidate; Tel: +86-731-4261329; E-mail: pxiahui@yahoo.com.cn
(Edited by CHEN Wei-ping)