J. Cent. South Univ. Technol. (2009) 16: 0599-0607
DOI: 10.1007/s11771-009-0100-9

Impact of human motion on TVOCs inhalation dose under side re-circulated ventilation
ZHANG Quan(张 泉)1, ZENG Li-ping(曾丽萍)1, XIE Geng-xin(谢更新)2,
ZHANG Guo-qiang(张国强)1, NIU Jian-lei(牛建磊)3
(1. College of Civil Engineering, Hunan University, Changsha 410082, China;
2. College of Environmental Science and Engineering, Hunan University, Changsha 410082, China;
3. Department of Building Services Engineering, Hong Kong Polytechnic University, Hong Kong, China)
Abstract: The main objectives were to (1) calculate the total volatile organic compounds (TVOCs) inhalation dose, (2) analyze the proportions of human’s inhaled contaminant dose from different sources, and (3) present a newly defined ratio of relative inhalation dose level (RIDL) to assess indoor air quality (IAQ). A user defined function based on CFD (computational fluid dynamics) was developed, which integrated human motion model with TVOCs emission model in a high sidewall air supply ventilation mode. Based on simulation results of 10 cases, it is shown that the spatial concentration distribution of TVOCs is affected by human motion. TVOCs diffusion characteristic of building material is the most effective way to impact the TVOCs inhalation dose. From the RIDL index, case A-2 has the most serious IAQ problem, while case D-1 is of the best IAQ.
Key words: indoor air quality (IAQ); human motion; computational fluid dynamics (CFD) simulation; volatile organic compounds (VOCs); contaminant; relative inhalation dose level (RIDL) index
1 Introduction
Due to the higher emission rates of VOCs (volatile organic compounds) in the initial period, decorative workers are always exposed in seriously polluted working environment [1-2]. The worker’s installation process can be divided into motionless and motion stages. The short and periodical motions may result in variations of environmental parameters [3-5], and different ventilation systems also have different impact on IAQ (indoor air quality) [6-7]. However, the interactive effects of characteristics of building material, human motion and ventilation, specially the human motion on IAQ analysis, have been included rarely in any previous studies. In general, indoor contaminant concentration and inhalation dose are two widely used factors to assess IAQ. In fact, they are not enough to describe IAQ without consideration of inhalation concentration variation, the corresponding exposure risk and its exposure period. Thus, a newly defined factor, relative inhalation dose level (RIDL) index, was provided to analyze and assess IAQ.
2 Modeling methods and IAQ assessment index
2.1 Relative inhalation dose level (RIDL) index
In order to assess IAQ accurately, a new RIDL
index was presented with the consideration of the synthetical impact of concentration level, inhalation dose and the corresponding time interval and exposure weighted ratios. RIDL index could be calculated according to following steps.
2.1.1 Inhalation dose
Human’s TVOCs inhalation dose can be calculated by Eqn.(1) during indoor decorative process. Transient respiration has some differences due to different physical conditions and activity levels [4, 8-11].
(1)
where Qj, total is the total TVOCs inhalation dose for case j (μg); Qi, j is the inhalation dose at the ith time interval for case j; Cnose is concentration at nose (μg/m3); Vb(t) is inhaled air volume (L/min); and m is the number of time interval.
2.1.2 Normalized inhalation dose
Normalized inhalation dose
is obtained by Eqn.(2) to describe the relative inhalation dose level between Qmax and Qmin. It belongs to the range [0, 1].
(2)
where
is the normalized inhalation dose at the ith time interval for case j; Qmax and Qmin are the maximum and minimum inhalation doses in all of the time intervals,
respectively.
2.1.3 Frequency of relative inhalation dose
The normalized interval [0, 1] is divided equally into a certain number of subintervals k, and the frequency feature of relative inhalation dose in each subinterval is calculated by
×100% (3)
where Pi is the frequency in the ith subinterval; k is the number of subinterval in the range of [0, 1], which is divided into 10 equal intervals in this work; and Ni is the number of the normalized inhalation dose that belongs to the ith subinterval.
2.1.4 RIDL index
RIDL index is defined by Eqn.(4). For healthy IAQ design, the case of the lowest RIDL index should be chosen.
(4)
where ηRIDL is the RIDL index;
is the assumed time of case j;
is the maximal exposure period among all the cases; Wi is the exposure weighted ratio, which is defined as
(5)
where Cnose(max, i) is the concentration at the nose of the maximal normalized TVOCs dose in the ith subinterval (μg/m3); and Cthreshold is the proposed indoor threshold of TVOCs concentration in China (μg/m3), which is defined as 500 μg/m3 [12].
2.2 Contribution ratio of pollution source (CRP) index
To evaluate the relationship between the inhalation dose and the contaminant source, the contribution ratio of a pollution source (CRP) index is used [13-14]. CRP index denotes the ratio of inhaled dose Qi emitted from the ith source to the total inhaled dose emitted by all the pollution sources. If the CRP is greater, the associated contaminant source will lead to more problems.
(6)
where ηCRP, i is the CRP index; n is the total number of the contaminant source; and QTVOCs,i is the TVOCs inhalation dose from the ith contaminant source.
2.3 Governing equation of TVOCs
To build emission model of TVOCs, several assumptions are made as follows.
(1) VOCs are emitted from the dried floor material and have no impact on airflow.
(2) Sorption effect on the interior building surfaces is not considered.
(3) There is no chemical reaction inside the material to generate or consume the VOCs.
2.3.1 Diffusion of TVOCs inside building material
The internal diffusion process of TVOCs inside the dried building material is governed by
(7)
where Cm is TVOCs concentration inside the building material (μg/m3); Dm is the diffusion coefficient of TVOCs in building material (m2/s); and xj is the coordinate in building material (m).
The dependence of Dm on temperature is assumed to follow Arrhenius law that is expressed by
(8)
where D0 is the initial diffusion coefficient at a referenced temperature (m2/s); Ed is the activation energy of TVOCs in building material (30.5 kJ/mol); R is universal gas constant (8.314 5 J/(mol?K)); and T is the temperature (K).
2.3.2 Human motion model
The human motion is simulated by using the dynamic mesh technique whose integral form of the conservation equation for a general scalar
is shown as follows [15].
○
Γ
(9)
where V is the arbitrary control volume for the moving boundary; ρ is the air density (kg/m3); u is air velocity (m/s); ug is the velocity of the moving grid (m/s); Γ is the effective diffusion coefficient for
and
is the source term for 
2.3.3 Boundary conditions
(1) Material layer
The emission flux of TVOCs at the material interface is equal to that at the air interface, as shown in Eqn.(10).
(10a)
Furthermore, it is assumed that
Cm, interface=kmaCa, interface (10b)
where Da is the diffusion coefficient of TVOCs in air (m2/s), Ca is TVOCs concentration in air (μg/m3), and kma is the partition coefficient of the material.
The TVOCs flux transfer through the bottom interface of the material is assumed as 0 by
(11)
(2) Inlet and outlet
For a re-circulated ventilation mode, the boundary conditions of inlet and outlet are described as [13, 16].
(12)
(13)
where uinlet and uoutlet are the inlet and outlet air velocities that are perpendicular to the corresponding cells (m/s); Cinlet and Coutlet are the inflow and outflow concentrations at corresponding cells (μg/m3); f is the fresh air ratio; and Ainlet and Aoutlet are inlet and outlet cell areas (m2).
(3) Nose and other object
The boundary condition at the nostril opening is defined for inhalation process, and concentration of an exhalation process is assumed as follows:
(14)
Cnose=0 (15)
2.4 Case description
A room with high sidewall air supply and return air distribution is defined and shown in Fig.1.
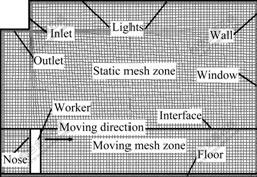
Fig.1 Diagram of side ventilation system model
The 2D configuration of the ventilation room is set as 4.5 m×3.0 m, and the window is set in the right-side wall. The temperatures of outside window and wall are assumed consistently as outdoor temperatures that are measured in a summer of Changsha, China [17]. The distance between the inlet and ceiling, and the distance between return grille and wall are both 0.15 m. The supply air temperature is set at 290 K. The indoor heat sources, mesh numbers and physical sizes are listed in Table 1. Ten cases are simulated under different conditions, which are listed in Table 2.
Table 1 Heat sources, mesh number and physical sizes
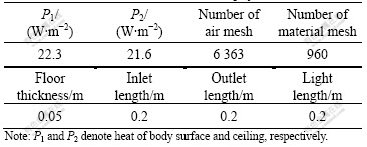
Table 2 Parameters of analyzed cases
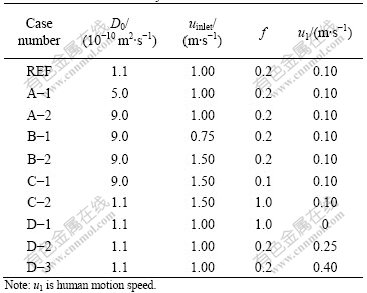
2.5 Numerical method
Personal exposure analysis and evaluation on IAQ are described by Eqns.(1)-(15). Firstly, having obtained the temperature distribution for indoor space and indoor floor at one time step, the diffusion coefficient at every cell node inside the floor is calculated using Eqn.(8). Secondly, the finite volume approach is used to discretize Eqn.(9), and the tri-diagonal matrix algorithm (TDMA) method [18] is used to solve the discrete equation sets with boundary conditions (Eqns.(10) and (11)). Thirdly, the indoor concentration distribution is gained by coupling equation sets Eqn.(7), Eqns.(12)-(15) and the concentration distribution at the interface of the building material. At last, the former results can be integrated with Eqns.(1)-(6) to calculate the CRP index and RIDL index with the consideration of human motion.
3 Validation of model
In order to verify the aforementioned model, the emission rate of TVOCs for case REF is compared with that of the numerical model put forward by HUANG and HAGHIGHAT [19]. In model of HUANG and HAGHIGHAT, kma and hm are set at 9 240 and 4.24×10-4, respectively according to Refs.[20-21]. Fig.2 shows emission rate of the two models. There is a good agreement for the emission rate of these two models. The correlation coefficient between the average emission rate of these two models is 99.5%.
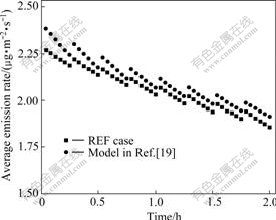
Fig.2 Comparison of average emission rate between case REF and model by HUANG and HAGHIGHAT [19]
4 Simulation results and discussion
4.1 Indoor air parameters based on moving scenario
We simulate a worker who installs eight wood boards in 2 h, whose length is 0.5 m and the initial concentration of the board is 5.28×107 ?g/m3. Installation time of one board is assumed as 15 min, and the human moves rightward and motion time interval is 5 s. During human’s motion, the acceleration and average velocity
are set at 0.4 m/s2 and 0.1 m/s, respectively. In this section, a moving scenario is assumed to gain insight into the impact of human motion on indoor variables, whose boundary conditions are defined as case REF listed in Table 2. The indoor temperature, concentration and streamline distributions fluctuate more obviously from 1.750 to 1.764 h, as shown in Fig.3. The low temperature region is expanded due to the human’s shift to right side for case REF moving scenario. A higher temperature region close to human body is observed, and it disappears along with time, as shown in Fig.3(a). Human’s breath zone gets purified, and the higher concentration region (C>500 μg/m3) moves to the left corner, as shown in Fig.3(b). Fig.3(c) shows that the clockwise airflow ‘A’ is expanded and counter-clockwise flow ‘B’ is shrunken simultaneously, then airflow ‘B’ disappears and clockwise airflow ‘A’ dominates the room. When the human moves, a counter-clockwise airflow near the person is observed and enhanced gradually.
4.2 Effect of human motion speed
The effect of human motion speed (u1) on TVOCs emission rate, inhalation concentration, TVOCs dose and CRP index is shown in Fig.4. Fig.4(a) shows that average emission rate falls down with the decrease of human motion speed u1. After every periodical human motion, the average emission rate increases and then decreases along with the increase of time. Fig.4(b) describes inhalation concentration variation with human motion
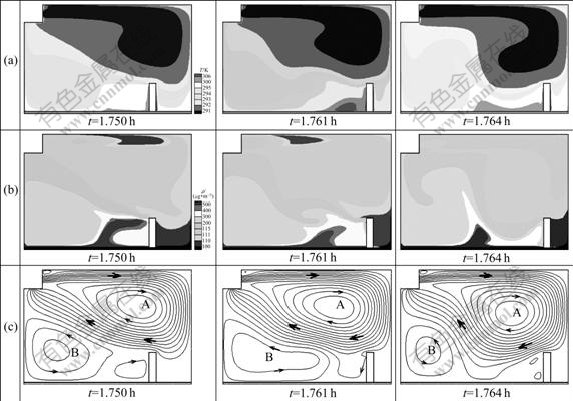
Fig.3 Temperature distribution (a), concentration distribution (b) and streamline distribution (c) under moving scenario
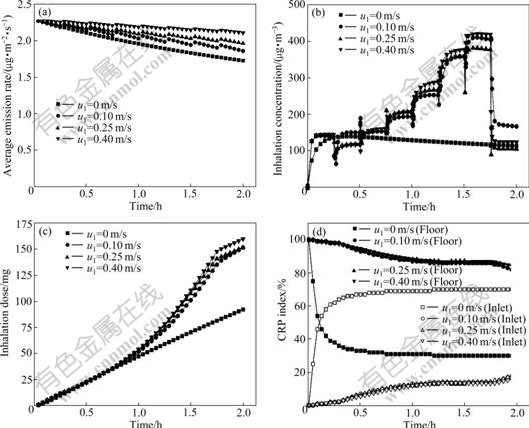
Fig.4 Influence of human motion speed u1 on different parameters: (a) Average emission rate; (b) Inhalation concentration;
(c) TVOCs inhalation dose; (d) CRP index
speed along with time. Inhalation concentration increases with increasing motion speed and fluctuates instantaneously after human motion. After human moves through main flow domain, inhalation concentration falls down quickly. Before 0.750 h, the inhalation concentration of motion cases is close to that of motionless case. After 0.750 h, the inhalation concentration of motion cases is greater than that of motionless case, which may be caused by the contaminant emitted from floor transported to inhalation area by human motion. Fig.4(c) shows that inhalation dose also enhances with increasing human motion speed. Inhalation dose is nearly the same at the initial stage (t<0.750 h). After that stage, differences between human motion and motionless cases are obviously observed. From Fig.4(d), because of the short motion period, we can conclude that CRP indices of floor and inlet are nearly kept at the same value except that for motionless case. Nearly 85% inhaled contaminant dose comes from floor and the rest comes from inlet for all human motion cases; while for motionless case, nearly 70% inhaled contaminant dose comes from floor and 30% inhaled contaminant comes from inlet. Among the inhalation doses, the majority dose comes from contaminant source and the fraction dose is from ventilation system for four different human motion speeds.
4.3 Effect of diffusion coefficient
Fig.5 illustrates the effect of coefficient D0 on average emission rate, inhalation concentration, inhalation dose and CRP index. From Fig.5(a), average emission rate fluctuates after human motion and its varying ranges of emission rate increase with increasing D0 level. When D0=1.1×10-10 m2/s, the emission rate is nearly kept at a constant value, which indicates that the internal diffusion dominates the TVOCs emission process. From Fig.5(b), the greater diffusion coefficient D0 results in greater inhalation concentration. When the human moves, a transient concentration fluctuation is observed in a short period of time. From Fig.5(c), higher D0 causes the higher inhalation dose. After 1.750 h, the decreased inhalation concentration may lead to the decrease of the slope of TVOCs inhalation dose curve. From Fig.5(d), although CRP index varies along with time, CRP indices with different D0 values are nearly equal to each other.
4.4 Effect of inlet airflow velocity
The inlet airflow velocity uinlet has small influence on average emission rate, which can be seen in Fig.6(a). The average emission rate also increases after human motion process. Inhalation concentration varies with time
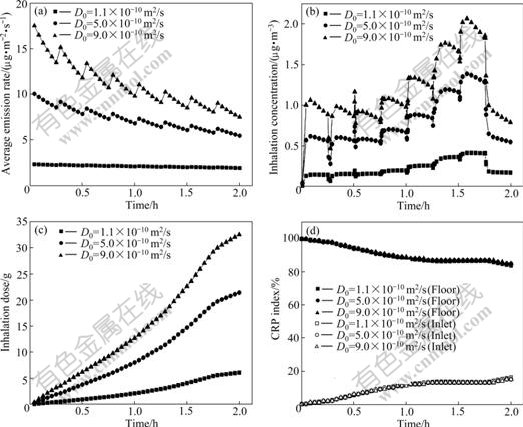
Fig.5 Influence of D0 on different parameters: (a) Average emission rate; (b) Inhalation concentration; (c) TVOCs inhalation dose; (d) CRP index
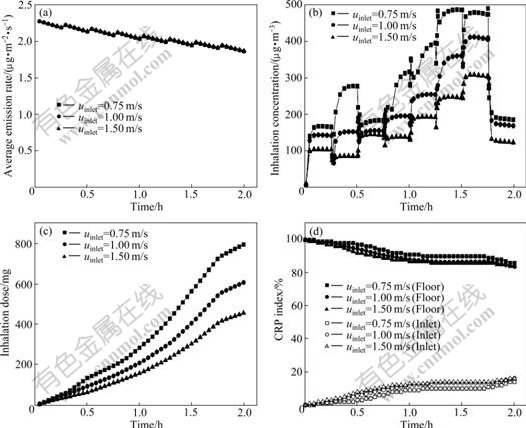
Fig.6 Influence of uinlet on different parameters: (a) Average emission rate; (b) Inhalation concentration; (c) TVOCs inhalation dose; (d) CRP index
periodically and the lower uinlet results in higher inhalation concentration, as shown in Fig.6(b). Fig.6(c) shows that inhalation dose increases with lower inlet velocity, which can be explained by higher inhalation concentration in lower velocity shown in Fig.6(b). From Fig.6(d), CRP index of the inlet increases little with the increase of uinlet, and variation tendency of CRP index at different inlet velocities is similar to each other.
4.5 Effect of fresh air ratio
The influence of fresh air ratio f on average emission rate, inhalation concentration, TVOCs inhalation dose and CRP index is illustrated in Fig.7. From Fig.7(a), the nearly same curves of emission rate with different f values show that f has little impact on the emission process. From Figs.7(b) and (c), inhalation concentration and TVOCs inhalation dose increase with the increase of f. From Fig.7(d), CRP index of inlet increases with the decrease of f. When f is set at 0.2, the CRP index of inlet is about 15%. When f equals 0.1, about 20% inhalation contaminant dose comes from inlet. When f=1.0, the CRP index of floor reaches 100%. The CRP index of floor falls down along with the increase of time, which leads to increasing CRP index of inlet.
4.6 Relative inhalation dose level index
From Figs.8(a1), (b1), (c1) and (d1), the normalized doses are described in a similar pattern under different boundary conditions, except the motionless case in Fig.8(a1). According to Figs.8(a2), (b2), (c2) and (d2), the variation trends of frequency of inhalation dose Pi with normalized dose have some similarities. The frequency in the subinterval [0.1, 0.3] is the largest and frequency in subinterval [0.6, 0.7] is the lowest. From Figs.8(a3), (b3), (c3) and (d3), the exposure weighted factors increase with the growth of normalized dose. The higher D0, lower uinlet and f lead to greater exposure weighted factor due to the higher concentration at the nose. For different human motion speeds, the exposure weighted factors are nearly the same, except the motionless case.
The values of relative inhalation does level (RIDL) index of different simulation cases are shown in Fig.9. Case A-2 with the largest D0 value has the highest RIDL index, which means that it will cause the most serious influence on occupant health, and case D-1 causes the least RIDL index. The impact of D0 deserves paying more attention than other boundary conditions, which suggests that control of diffusion coefficient D0 is the most effective way to improve the indoor air quality.
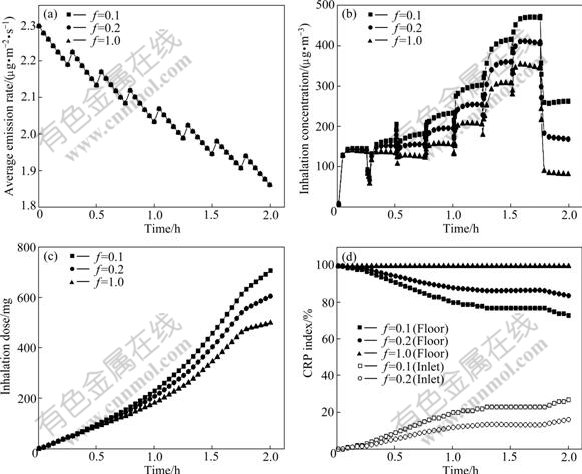
Fig.7 Influence of f on different parameters: (a) Average emission rate; (b) Inhalation concentration; (c) TVOCs inhalation dose; (d) CRP index
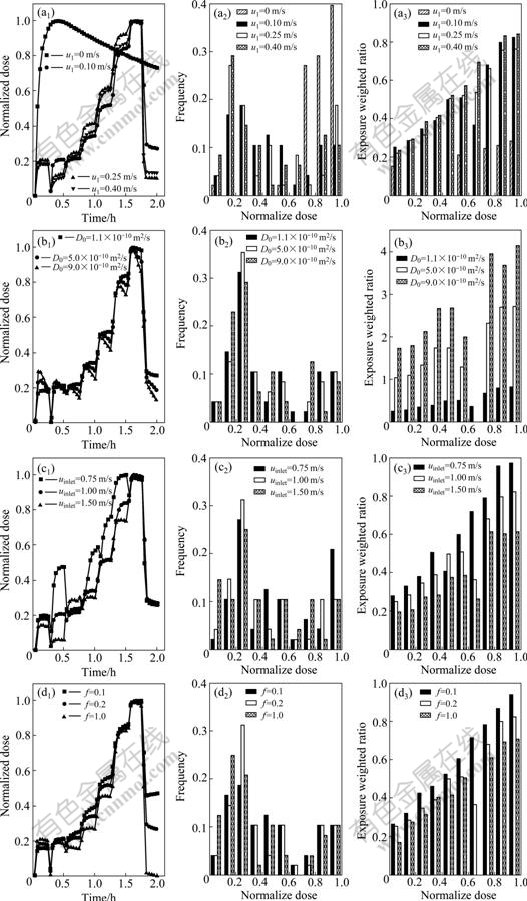
Fig.8 Normalized dose, frequency and exposure weighted ratio with human motion speed u1 (a), diffusion coefficient D0 (b), inlet airflow velocity uinlet (c) and fresh air ratio f (d)
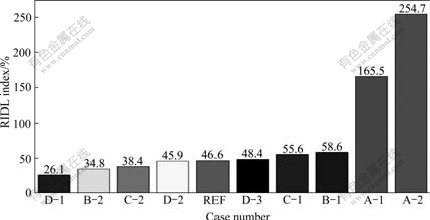
Fig.9 Human relative inhalation dose level (RIDL index) for 10 different cases
5 Conclusions
(1) Indoor temperature, concentration and velocity distribution are disturbed by human motion. For the motion case, nearly 85% inhaled contaminant dose comes from floor, while for motionless case, nearly 70% inhaled contaminant dose comes from floor.
(2) The higher diffusion coefficient D0 and human motion speed u1, lower inlet airflow velocity uinlet and fresh air ratio f result in higher concentration at the nose and greater TVOCs inhalation dose. Among the four influence factors, the control of D0 is the most effective way to reduce RIDL index. Meanwhile, the human motion will lead to more inhalation dose.
References
[1] LEE S C, GUO H, LI W M, CHAN L Y. Inter-comparison of air pollutant concentrations in different indoor environments in Hong Kong [J]. Atmospheric Environment, 2002, 36(12): 1929-1940.
[2] WOLKOFF P, NIELSEN G D. Organic compounds in indoor air—Their relevance for perceived indoor air quality [J]. Atmospheric Environment, 2001, 35(26): 4407-4417.
[3] BJORN E, NIELSEN P V. Dispersal of exhaled air and personal exposure in displacement ventilated rooms [J]. Indoor Air, 2002, 12(3): 147-164.
[4] MATTSSON M. On the efficiency of displacement ventilation, with particular reference to the influence of human physical activity [D]. Stockholm: Royal Institute of Technology, 1999.
[5] XIE Dong, WANG Han-qing, DENG Qi-hong, XIONG Jun. Investigation on different ventilation patterns in an office room [J]. J Cent South Univ Technol, 2007, 14(S3): 66-70.
[6] YANG X D, SREBRIC J, LI X T, HE G Q. Performance of three air distribution systems in VOC removal from area source [J]. Building and Environment, 2004, 39(11): 1289-1299.
[7] WANG Z, BAI Z, YU H, ZHANG J, ZHU T. Regulatory standards related to building energy conservation and indoor-air-quality during rapid urbanization in China [J]. Energy and Buildings, 2004, 39(12): 1299-1308.
[8] GAO Nai-ping, NIU Jian-lei. CFD study on micro-environment around human body and personalized ventilation [J]. Building and Environment, 2004, 39(7): 795-805.
[9] REN Z, STEWART J. Prediction of personal exposure to sources in industrial buildings using a sub-zonal model [J]. Environmental Modelling and Software, 2005, 20(5): 623-638.
[10] ZHU S W, KATO S, MURAKAMI S, HAYASHI T. Study on inhalation region by means of CFD analysis and experiment [J]. Building and Environment, 2005, 40(10): 1329-1336.
[11] HAYASHI T, ISHIZU Y, KATO S, MURAKAMI S. CFD analysis on characteristics of contaminated indoor air ventilation and its application in the evaluation of the effects of contaminant inhalation by a human occupant [J]. Building and Environment, 2002, 37(3): 219-230.
[12] WANG Xi-yuan, LIU Hong-kui, PAN Hong. Standard for indoor environmental pollution control of residential building in China [M]. Beijing: The Construction Department of China, 2001. (in Chinese)
[13] ZHANG Quan, ZHANG Guo-qiang. Study on TVOCs concentration distribution and evaluation of inhaled air quality under a re-circulated ventilation system [J]. Building and Environment, 2007, 42(3): 1110-1118.
[14] MURAKAMI S. Analysis and design of micro-climate around the human body with respiration by CFD [J]. Indoor Air, 2004, 14(7): 144-156.
[15] WANG Rui-jin, ZHANG Kai, WANG Gang. Fluent technical foundation and application example [M]. Beijing: Tsinghua University Press, 2007. (in Chinese)
[16] ZHANG Q, KATO S, TSAY Y S. Prediction of indoor TVOCs concentration distribution with a fluctuant temperature of the outwall under a mixing ventilation system [C]// Proceedings of Air Conditioning. Nagoya, 2005: 845-848.
[17] ZHANG Quan, YUAN Hao, ZHANG Guo-qiang, DENG Tian-fu. Study of the impact of human motion on indoor thermal comfort [J]. Journal of Hunan University: Natural Sciences, 2007, 34(8): 20-25. (in Chinese)
[18] ARAKAWA C. Computational fluid dynamics for engineering [M]. Tokyo: Tokyo University Press, 1994.
[19] HUANG H Y, HAGHIGHAT F. Modelling of volatile organic compounds emission from dry building materials [J]. Building and Environment, 2002, 37(12): 1349-1360.
[20] BODALAL A, ZHANG J S, PLETT E G. A method for measuring internal diffusion and equilibrium partition coefficients of volatile organic compounds for building materials [J]. Building and Environment, 2000, 35(2): 101-110.
[21] ZHANG Guo-qiang, YU Yue-bin, HAN Jie. Research on the influencing factors of VOCs emission from building materials [J]. Hunan University: Natural Science, 2004, 31(6): 60-64. (in Chinese)
(Edited by CHEN Wei-ping)
Foundation item: Projects(2006BAJ02A08, 2006BAJ02A05) supported by the National Science and Technology Pillar Program Project during the 11th Five-Year Plan Period; Project(2007-209) supported by the Excellent Youth Teacher of Ministry of Education of China
Received date: 2008-11-26; Accepted date: 2009-03-20
Corresponding author: ZHANG Quan, Professor, PhD; Tel: +86-731-88821254; E-mail: zhangquan@hnu.cn