
Influence of processing parameters on coating surface roughness of aluminum alloy
PAN Ming-qiang(潘明强), CHI Guan-xin(迟关心), WEI Dong-bo(韦东波), DI Shi-chun(狄士春)
School of Mechatronics Engineering, Harbin Institute of Technology, Harbin 150001, China
Received 10 June 2009; accepted 15 August 2009
Abstract: The influence of processing parameters on the coating surface roughness of LD10 aluminum alloy treated by the power source with positive and negative pulse in the alkaline electrolyte was discussed. Lots of experiments were done with different processing parameters including the positive final voltage, the negative final voltage, the frequency, the duty ratio, the processing time and electrolyte temperature. And the surface roughness tester TR200 was used to measure the coating surface roughness. The influence of the processing time on the surface roughness is the smallest, less than 0.2 μm. The surface roughness decreases gradually with the duty ratio decreasing or the frequency increasing; it decreases gradually with each of the other parameters increasing, and then increases gradually after achieving the minimum value. Appropriately by selecting processing parameters, the coating with Ra 0.6 can be obtained.
Key words: micro-arc oxidation; processing parameter; surface roughness; aluminum alloy
1 Introduction
Micro-arc oxidation with the remarkable advantages of surface modification, environmentally friendly technology, simple process and easy operation etc, obtains the rapid developing in recent years. Lots of researches focus on the process exploration, the coating properties and the development of power supply. Though few researches were about the surface roughness, some descriptions were mentioned in some papers. For example, YEROKHIN et al[1] used the optical microscope to observe the cross section of micro-arc oxidation coating, and discovered the coating with a dense inner layer transforming to a porous outer layer, with an associated surface roughness (Ra=3-6 μm). And by treating the 2024 aluminum with a modified plasma electrolytic oxidation, the surface roughness of the PBC-PEO coatings was much low (Ra=0.75-1.5 μm) due to the thinner porous layer[2]. LUKIYANCHUK et al[3] discovered that addition of boric acid into tungstate electrolyte promotes formation of coatings with rougher surfaces[3]. In fact, the influence of the coating thickness on the surface roughness was often mentioned. Many scholars have discovered that the surface roughness increases linearly with the coating thickness[4-6]. Though the coating surface roughness is mentioned in above references, the influence of the various factors on the surface roughness was not roundly analyzed. For the purpose to obtain the coating with good surface property, investigating the influencing factors of the coating surface roughness and controlling the surface roughness are very significant. This study aims to investigate the influence of the processing parameters on the surface roughness of the micro-arc oxidation coating on LD10 aluminum alloy.
2 Experimental
The LD10 aluminum alloy was used as the substrate material in the present study. The chemical compositions of alloy in mass fraction (%) are: Cu 3.9-4.8, Mg 0.4-0.8, Mn 0.4-1.0, Si 0.6-1.2, Fe 0.7, Zn 0.30, Ni 0.1, Ti 0.15, Al balance. Samples, in the plate shape with thickness of 5 mm and diameter of 30 mm, were treated according to the parameters presented in Table 1 by the power source with positive and negative pulse in the alkaline electrolyte composed of ADS10* (the chemical invented to treat Al by Harbin DS Numerical Control Equipment Co., Ltd.). The stainless steel tank of 50 L capacity was the cathode and the sample was the anode, and the negative duty ratio always equaled the positive duty ratio. The processing parameters were set directly, and the temperature precision was ±3 ℃. The voltage waveforms were controlled by the same program in all experiments.
Table 1 Experimental parameters
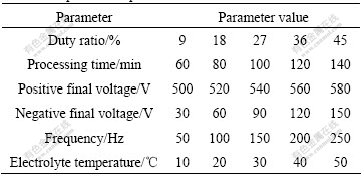
The substrates were ground and polished to Ra 0.1 μm, and then washed with tap water and dried in air. After the treatment finishing, the coating surface roughness was measured by the surface roughness tester TR200 with a precision of 0.01 μm, a range of 0.025-12.5 μm, and the sampling length of 0.8 mm. Each sample was measured continuously 5 times, 3 times free to measure and 2 times in the diameter survey in the mutually perpendicular direction. And then the average of 5 times was the value of the coating surface roughness. To reduce the measuring error, all samples were measured together.
3 Results and discussion
3.1 Influence of positive final voltage on surface roughness
The surface roughness of the micro-arc oxidation coating is strongly dependent on the substrate surface roughness and the coating surface microstructure and microshape, which formed by the overflow of the fusing material during processing. When the substrate surface roughness is invariable, the coating surface roughness is decided by the surface microstructure and microshape.
The reciprocal process, namely, the coating growth or the coating dissolution, always accompanies micro-arc oxidation process. In the growth process the particles and crater, as shown in Fig.1, are generated during the micro-arc discharging, and the new coating surface microstructure and micro shape form. And in the dissolution process, the amorphous phase part is dissolved and the micropore increases. Under the influence of the processing parameters and the electrolyte, the two processes always exist at the same time and affect each other. With changing parameters, the particles crater, and the micropore in diameter vary, and the microshape of the coating surface, as shown in Fig.2, will to change correspondingly.
In the micro-arc oxidation processing, the positive final voltage right affects the processing energy of a single pulse, which influences the particles and crater generated during micro-arc discharging in diameter, and the number and volume of the discharging spark. And
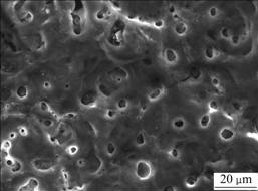
Fig.1 Coating surface microstructure
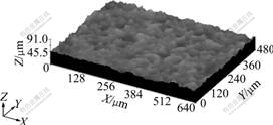
Fig.2 3-D microshape of coating surface
decreasing the particles and crater, and/or enhancing the spark density, the coating surface roughness will decrease.
Fig.3 represents the costing surface roughness vs the positive final voltage when other parameters are presented in the third row of Table 1. With increasing voltage, at first the surface roughness continues to decrease, then begins to increase quickly after reaching the minimum value Ra 0.6 μm when the voltage is 540 V.
When the positive final voltage is less than 540 V, the spark density is the key to influence the coating
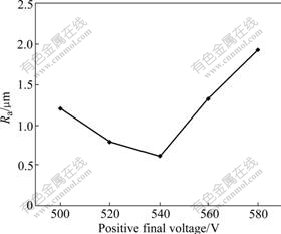
Fig.3 Influence of positive final voltage on coating surface roughness
surface roughness. With increasing voltage, the spark volume has little change, but the density quickly increases. The smaller the spark volume is, the less the product is generated during the micro-arc discharging, and the denser the spark is, the better the product overlaps and melts each other. The good overlapping and melting counteracts the increment of the surface roughness caused by the particles and crater. Thus, the changing tendency is reduced.
When the positive final voltage is more than 540 V, the spark volume is the key to influence the coating surface roughness. When the voltage increases, the spark volume increases fast, and the spark density starts to reduce because of the central discharging. The violent reaction causes the discharging channel to heat up, more fusing material outward, and passageway expanded. The big particles and crater are generated after the product is frozen. These cause the coating surface to be rougher finally.
3.2 Influence of negative final voltage on surface roughness
During processing, the electric field produced by the negative voltage is helpful to distributing the cation and anion evenly in the electrolyte. On one hand, the cation enters the electric discharge channel after once discharging under the action of the electric field, and joins in the next discharging process. On the other hand, the electric field makes the anion far away from the coating surface. These make the cation and anion even distribute near the workpiece surface, eliminate the polarized action in the oxidation process, and keep the superficial appearance consistent. At the same time, the negative electric field can dissolve the coating, and influence the discharging sparks. The influence of the negative voltage on the surface roughness is dependent on its function.
Fig.4 shows the relationship between the coating surface roughness and the negative final voltage. This curve is similar to that of the positive final voltage.
It is discovered in the experiment that the negative voltage can promote the electrolyte to dissolve the coating, and influence the quantity and volume of the discharging spark. When the negative final voltage is less than 90 V, it mainly makes the spark quantity increase. Therefore, the surface roughness change is small, and keeps the reducing tendency. When the negative final voltage is more than 90 V, it makes the spark volume increase. With the processing carrying on, the large red spark volume gradually increases, and the quantity gradually decreases. The higher the voltage is, the more quickly the spark quantity and volume change; and the surface becomes rougher and rougher.
3.3 Influence of frequency on surface roughness
As shown in Fig.5, with the frequency rising, the surface roughness reduces, and after the frequency achieves 150 Hz, it has little influence on the surface roughness.
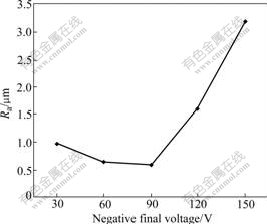
Fig.4 Influence of negative final voltage on coating surface roughness
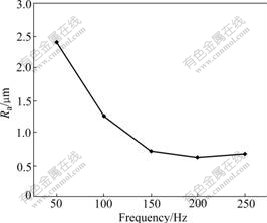
Fig.5 Influence of frequency on coating surface roughness
When the voltage and the duty ratio are fixed, the frequency is the only method to change the single pulse energy. Although there are many sparks to produce and vanish in one pulse period, the maintenance time of the single spark is different for different frequencies. According to the pictures taken by the high-speed camera, it is found that the maintenance time processed by the low frequency is longer than the time processed by the high frequency. Under the same processing voltage condition, the shorter the time which the spark continues is, the less the product is generated during discharging. And the little production will form the small particles and crater. Therefore, the coating surface roughness is low. Because of these reasons, the surface roughness presents the change rule as shown in Fig.5.
The frequency influences not only the coating surface roughness, but also the hardness, thickness and other properties. Because the product decreases with the frequency increasing, the incompleteness or very little of the fusing material can overlap each other. And the thickness decreases with the frequency increasing. When the frequency is more than 200 Hz, the thickness will reduce quickly, and the coating is incomplete. So, the best value of the frequency is 150 Hz.
3.4 Influence of duty ratio on surface roughness
Fig.6 shows the change rule of the coating surface caused by the duty ratio. From the change tendency of the curve, the coating surface roughness will increase with the duty ratio after the duty ratio is more than 27%.
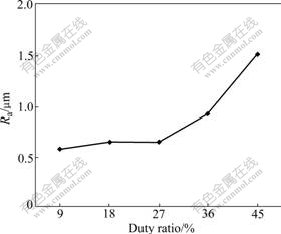
Fig.6 Influence of duty ratio on coating surface roughness
In the experiment, the time that the large red spark appears gradually shortens with the duty factor elevation. By comparison with processing with 27% duty ratio, the time of the large red spark appearing by 45% is ahead of 24 min. Because the energy of the large red spark is larger than that of small spark, the discharging product also increases, and the volume of the particles and crater is larger in the corresponding process, therefore, the surface roughness rises.
The larger the duty ratio is, the shorter the downtime between the two pluses is. Because the fusing material is frozen and is transformed into the crystal structure in the downtime, more material cannot transform when the downtime is short. And the amorphous phase is dissolved in the negative pulse part, which increases the holes and crater in the coating, and further increases the surface roughness. When the duty ratio is less than 27%, its influence is similar to the frequency influence. Though the influence of the coating surface roughness is very small, the coating is thin too. Therefore, the best value is 27%. Based on the above reason, the coating surface roughness processed by the different duty ratios presents the change rule, as shown in Fig.6.
3.5 Influence of processing time on surface roughness
As Fig.7 shows, the change rule of the coating surface roughness, caused by the processing time, presents similar to the frequency. When the time is 100 min, the minimum value Ra of 0.6 μm is obtained. From the curve, the influence of the processing time on the surface roughness is small on the whole experiment, and the difference is not more than 0.2 μm, and far less than that caused by other processing parameters.
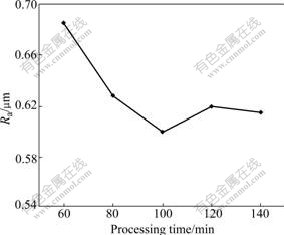
Fig.7 Influence of processing time on coating surface roughness
In the micro-arc oxidation process, the voltage continues to rise after going through the stage of the anodizing[7]. When the control voltage exceeds a certain threshold voltage value, which is 140 V for this experiment, the week area distributing in the coating is broken down because the material is failed[8], and the surface starts to distribute a large number of intensive, small, and white spark. The spark color starts to turn from white to orange with processing, and the spark is still evenly-intensive. A lot of the tiny fusing materials are generated during discharging spray to the surface, and the new coating forms after they are frozen and solidified by the electrolyte.
Because the voltage is used to control the process in the experiment, when the spark color changes in the weak area, the oxide starts to be transferred from the amorphous phase to the phase structure. At this moment, the particles and crater generated during discharging are discontinuous. When the processing time is short, for example, less than or equal to 60 min, the coating surface is rough because the fusing material is generated during discharging overlapping and melting mutually is not incomplete. With the time prolonging, the material begins to overlap and melt each other because the weak area moves and the position of the phase transition increases, therefore, the surface roughness also gradually reduces. At the same time, the current and the spark quantity starts to reduce gradually because of the processing prolonging and the thickness increasing. This further reduces the coating surface roughness. At last, the coating gradually achieves the dynamical equilibrium of the solution and the deposition, and the surface roughness starts to tend to a stable value that presents the rule of Fig.7. Not only the coating surface roughness does not change with the time increasing, but also all the other properties cannot be improved after the time more than 100 min. Therefore, 100 min is the best value of the processing time.
3.6 Influence of electrolyte temperature on surface roughness
The electrolyte temperature is quite important in the micro-arc oxidation process. Because the process produces heat that causes the electrolyte temperature to elevate, which affects the coating surface roughness via changing the processing current, the capacity of the electrolyte dissolving and freezing. As Fig.8 shows, the rule of the influence of the electrolyte temperature on the surface roughness is very similar to that of the negative final voltage. At first it reduces slowly, and then increases rapidly after achieving the minimum value of Ra 0.6 μm at 30 ℃.
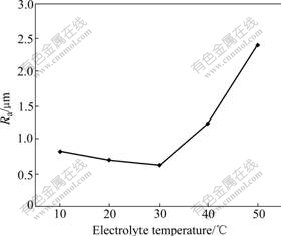
Fig.8 Influence of electrolyte temperature on coating surface roughness
When the temperature is low, for example 10 ℃, the processing current is small, and the spark is very tiny. Because the condensation of the fusing material to the particles and crater is excessively quick, they are small irregular column and do not overlap and melt mutually. Therefore, the coating surface roughness is large. With the temperature rising, they start to overlap and melt mutually because of the current and the fusing material increasing and the condensation speed reducing. And the surface roughness has the slight reduction tendency.
With the temperature elevating, more than 30 ℃, the processing current further increases and causes the energy to be enhanced. And the energy enhanced causes the reaction to be the fierce reaction. It makes the surface roughness increase because the size of the fusing material and the hole generated during discharging increases. At the same time, the enhancing current and increasing temperature make the reaction happen in the weak area, but also in slightly thick area. This further increases the surface roughness. Furthermore, increasing temperature makes the condensation speed drop and the dissolving capacity of the electrolyte improve. The surface roughness also increases because plenty of fusing materials are the amorphous phase and are dissolved. These factors cause the coating surface roughness to present the change rule that is shown in Fig.8. The best point is 30 ℃, but the mechanism needs to be further studied.
4 Conclusions
1) The coating surface roughness achieves the minimum value of Ra 0.6 μm at the positive final voltage of 540 V and the negative final voltage of 90 V.
2) Increasing frequency, and/or decreasing the duty ratio are advantage to improving the coating surface roughness.
3) The influence of the temperature on the surface roughness is similar to that of the positive final voltage and the negative final voltage. And the best value is 30 ℃.
4) Compared with other parameters, the effect of the processing time is inconspicuous, and the difference of surface roughness is less than 0.2 μm.
5) The best surface roughness can be gained by adjusting the parameters. And the coating of the best surface roughness of Ra 0.6 μm will be gained at the positive final voltage of 540 V, the negative final voltage of 90 V, the frequency of 150 Hz, the duty ratio of 27%, the processing time of 100 min, the electrolyte temperature of 30 ℃ and the electrolyte fixed conditions. Therefore, the influence of the electrolyte on the surface roughness needs more in-depth study.
Acknowledgments
The work was supported by the Innovation Fund for Small Technology-based Firms for Harbin DS Numerical Control Equipment Co., Ltd. Samples, the micro-arc oxidation equipment and chemicals were provided by the company. The authors express their thanks to the technical staffs of the company for their useful helps and advices.
References
[1] YEROKHIN A L, SNIZHKO L O, GUREVINA N L, LEYLAND A, PILKINGTON A, MATTHEWS A. Spatial characteristics of discharge phenomena in plasma electrolytic oxidation of aluminium alloy [J]. Surface and Coatings Technology, 2004, 177/178: 779-783.
[2] YEROKHIN A L, SHATROV T A, SAMSONOV V, SHASHKOV P, PILKINGTON A, LEYLAND A, MATTHEWS A. Oxide ceramic coatings on aluminium alloys produced by a pulsed bipolar plasma electrolytic oxidation process [J]. Surface and Coatings Technology, 2005, 199: 150-157.
[3] LUKIYANCHUK I V, RUDNEV V S, KURYAVYI V G, BULANOVA S B, GORDIENKO P S. Surface morphology, composition and thermal behavior of tungsten containing anodic spark coatings on aluminium alloy [J]. Thin Solid Films, 2004, 446: 54-60.
[4] PARFENOV E V, YEROKHIN A L, MATTHEWS A. Frequency response studies for the plasma electrolytic oxidation process [J]. Surface and Coatings Technology, 2007, 201: 8661-8670.
[5] CURRAN J A, CLYNE T W. Thermo-physical properties of plasma electrolytic oxide coatings on aluminium [J]. Surface and Coatings Technology, 2005, 199: 168-176.
[6] SUNDARARAJAN G, KRISHNA L R. Mechanisms underlying the formation of thick alumina coatings through the MAO coating technology [J]. Surface and Coatings Technology, 2003, 167: 269-277.
[7] WIRTZ G P, BROWN S D, KRIVEN W M. Ceramics coating by anodic spark deposition [J]. Mater Manuf Process, 1991, 6(1): 87-155.
[8] YEROKHIN A L, LYUBIMOV V V, ASHITKOV R V. Phase formation in ceramic coatings during plasma electrolytic oxidation of aluminium alloys [J]. Ceramics International, 1998, 24(1): 1-6.
(Edited by YANG Hua)
Corresponding author: PAN Ming-qiang; Tel: +86-451-86416325; Fax: +86-451-86413834; E-mail: pmqwl@163.com