J. Cent. South Univ. Technol. (2011) 18: 1924-1929
DOI: 10.1007/s11771-011-0924-y
Influence of sulfur content in raw materials on oxidized pellets
CHUN Tie-jun(春铁军), ZHU De-qing(朱德庆), PAN Jian(潘建)
School of Minerals Processing and Bioengineering, Central South University, Changsha 410083, China
? Central South University Press and Springer-Verlag Berlin Heidelberg 2011
Abstract: The influence of sulfur content in raw materials on oxidized pellets was studied. The results show that most sulfur exists in the form of elementary sulfur in pyrite cinder, and over 95% sulfur is removed in producing pyrite cinder oxidized pellets. The compressive strength of fired pellets drops from 3 186 N to 2 405 N when the ratio of pyrite cinder increases from 40% to 70% under the conditions of preheating at 900 °C for 9 min and firing at 1 230 °C for 15 min. The porosity and microstructures of fired pellets prove that the higher ratio of pyrite cinder is given, and the more holes and cracks are achieved, leading to the better reducibility index (RI) and reduction swelling index (RSI), and the lower compressive strength of fired pellets and the worse reduction degradation index (RDI).
Key words: pyrite cinder; desulfuration; compressive strength; porosity; microstructures
1 Introduction
Pyrite cinder, as a subsidiary product of sulfuric acid production by roasting of pyrite ores, occupies much land and pollutes the environment. According to the statistics, the annual production of pyrite cinder is about 15 million tons in China [1-3]. Besides, there is lots of pyrite cinder deposited for many years. The pyrite cinder consumed in the cement and other industries as auxiliary additives only accounts for less 30% of its total discharge. Besides, high iron grade pyrite cinder can be used as the materials for sintering or pelletizing in iron-making industry [4-6].
Compared with traditional iron ore concentrates, pyrite cinder possesses many disadvantages for pelletizing [7-11], such as higher sulfur content, higher bentonite dosage, lower strength and higher moisture content of green pellets and adverse firing performance. There were extensive researches on the utilization of pyrite cinder in pelletizing, such as pre-treating pyrite cinder by high press grinding roller (HPGR) before pelletizing to improve balling index, mixing magnetite concentrate with pyrite cinder to improve the firing performance of pellets, mixing low sulfur iron ores with pyrite cinder to reduce the sulfur content of materials.
In pelletizing, the pyrite cinder oxidized pellets were produced at constant ratio of pyrite cinder with magnetite concentrate, and the study discussed the effects of different temperatures and times on the desulfuration of pyrite cinder pellets [12-15]. The conclusion is drawn that most sulfur is removed and the final fired pellets can be used as the burden for blast furnace. However, there is few research about the effects of different sulfur contents in materials on the properties of pyrite cinder pellets, such as compressive strength, metallurgical performance, porosity and microstructure of fired pellets. Therefore, the influence of sulfur content in raw materials on pyrite cinder oxidized pellets was studied in this work by mixing different ratios of pyrite cinder and magnetite.
2 Materials and methods
2.1 Raw materials
The raw materials include the pyrite cinder, magnetite concentrate and bentonite, all of which were supplied by Yunfu Pyrite Company, Guangdong Province, China. Their chemical compositions are presented in Table 1. The iron grades of the pyrite cinder and magnetite are over 63%, which is suitable iron grade for pelletizing materials. The mass fraction of particles size less than 0.074 mm of pyrite cinder is 89.54%, and that of magnetite, 96.60%.
The quality of bentonite is excellent due to higher montomorillonite content of 90.5%, high swelling volume of 15.8 mL/g, water adsorption of 416% and fine size of 86.72% particle less than 0.074 mm.
Distributions of sulfur in pyrite cinder are given in Table 2. It is shown that the compositions of sulfur in pyrite cinder are elementary sulfur, sulfate and sulfide. Over 87% sulfur exists in the form of elementary sulfur which can be removed by oxidizing reaction at high temperature in producing fired pellet.
Table 1 Chemical compositions of raw materials

Table 2 Sulfur content distributions in pyrite cinder
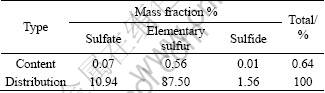
Table 3 demonstrates the sulfur content of mixing feed at different ratios. Four pyrite cinder ratios from 40% to 70% were studied, and the sulfur contents in mixing feed are 0.27%, 0.332%, 0.393% and 0.455%, respectively.
Table 3 Sulfur content of mixing feed at different ratios
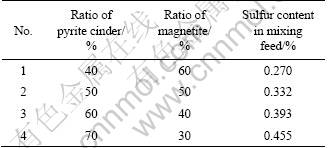
The particles morphologies of pyrite cinder and magnetite concentrate under scanning electron micro- scope (SEM) are depicted in Fig.1. It can be seen that pyrite cinder particles possess much higher porous rough surface than magnetite concentrate particles, leading to higher moisture to make green balls.
2.2 Experimental methods
The flowsheet of pelletizing tests in this work includes the traditional section, such as mixing pyrite cinder with magnetite concentrate and bentonite at a given ratio, balling in a disc pelletiser, drying in the oven and preheating and firing in a tube furnace to make fired pellets.
Green pellets were made from the mixture of pyrite cinder, magnetite and 1.8% bentonite in a disc pelletiser of 0.8 m in diameter and 0.2 m in rim depth, rotational speed at 38 r/min and inclined at 47° to the horizontal direction. Drying of green pellets was carried out in the oven at 105 °C for 2 h. Dried balls were preheated at 900 °C for 9 min and fired in a tube furnace of 50 mm in diameter and 600 mm in width. Both ends of tube were open and no air flowed into the tube furnace. The compressive strength and metallurgical performance of the fired pellets were measured to evaluate the quality of the fired pellets.
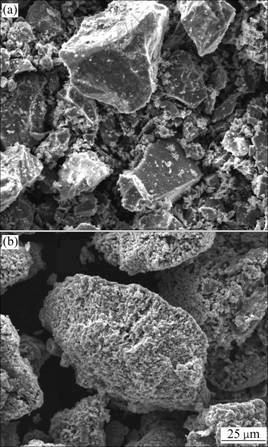
Fig.1 Morphologies of magnetite and pyrite cinder under SEM: (a) Magnetite concentrates; (b) Pyrite cinder
Compressive strength of fired pellets was tested according to the standard of ISO 4700. Apparent density and true density of fired pellets were tested by pycnometer method. The porosity (ε=1-ρ1/ρ2) was obtained by the results of apparent density (ρ1) and true density (ρ2). Metallurgy performance and chemical composition were also tested by corresponding ISO standards. Microstructures of materials and fired pellets were measured by using SEM and optical microscopy.
3 Results and discussion
3.1 Firing temperature and time
Figures 2 and 3 show the effects of firing temperature and time on the sulfur content of fired pellets and desulfuration at the ratio of pyrite cinder of 50%. The sulfur content of fired pellets decreases obviously and the desulfuration increases dramatically when the firing temperature is elevated from 1 130 °C to 1 230 °C for 15 min or the firing time is extended from 9 to 15 min at 1 230 °C. However, the sulfur content of fired pellets reduces slightly when the firing temperature is beyond 1 230 °C for 15 min or the firing time is prolonged to 18 min at 1 230 °C. Therefore, the sulfur content of fired pellets of 0.009% and desulfuration of 97.29% are obtained at the optimum conditions of firing at 1 230 °C for 15 min.
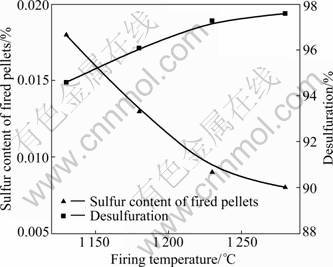
Fig.2 Effect of firing temperature on sulfur content of fired pellets and desulfuration (Preheating at 900 °C for 9 min and firing for 15 min)
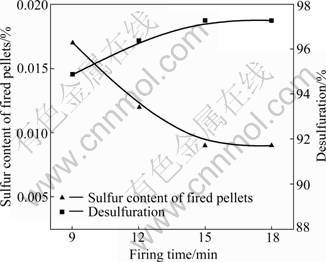
Fig.3 Elffect of firing time on sulfur content of fired pellets and desulfuration (Preheating at 900 °C for 9 min and firing at 1 230 °C)
3.2 Pyrite cinder ratios
The effects of pyrite cinder ratios on the sulfur content of fired pellets and desulfuration are shown in Fig.4. The sulfur content of fried pellets climbs from 0.005% to 0.019% as the ratio of pyrite cinder increases from 40% to 70%. Meanwhile, the desulfuration drops slightly from 98.15% to 95.82%. It can be concluded that over 95% sulfur of materials is removed when the ratio of pyrite cinder is added from 40% to 70% in producing oxidized pellets. Because sulfur exists in the form of elementary sulfur, sulfur is removed in the form of SO2 and escaped into exhaust gases.
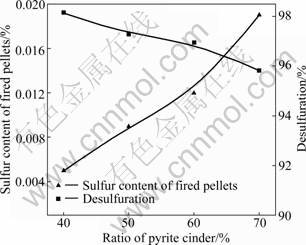
Fig.4 Effects of pyrite cinder ratios on sulfur content of fired pellets and desulfuration (Preheating at 900 °C for 9 min and roasting at 1 230 °C for 15 min)
3.3 Compressive strength of fired pellets
Figure 5 illustrates the effect of pyrite cinder ratios on the compressive strength of fired pellets. The higher the ratio of pyrite cinder is elevated, the lower compressive strength the fired pellets is obtained. The compressive strength of fired pellets drops from 3 186 N to 2 405 N when the ratio of pyrite cinder increases from 40% to 70%. Due to the fact that over 95% sulfur of materials is removed in the form of SO2 in preheating and firing stages, the cracks are produced in oxidized pellets when SO2 is removed from the inner of pellets, leading to the low compressive strength of fired pellets. The further reasons will be discussed in porosity and microstructures of fired pellets.
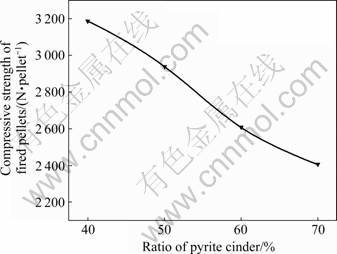
Fig.5 Effect of pyrite cinder ratios on compressive strength of fired pellets (Preheating at 900 °C for 9 min and roasting at 1 230 °C for 15 min)
3.4 Porosity of fired pellets
Table 4 demonstrates the effect of pyrite cinder ratios on porosity of fired pellets. The porosity of fired pellets increases from 9.15% to 11.69% when the ratio of pyrite cinder is enhanced from 40% to 70%. The 70% pyrite cinder ratio pellets possesses higher porosity due to higher sulfur content in materials, and most sulfur is removed in high temperature oxidizing process. The higher the porosity is, the lower the compressive strength of fired pellets is. This agrees with the results of compressive strength of fired pellets in Fig.5.
Table 4 Effect of pyrite cinder ratios on porosity of fired pellets
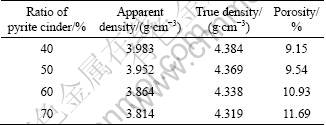
3.5 Metallurgical performance of fired pellets
It is shown in Table 5 that the fired pellets possess the superior reducibility index (RI) and reduction swelling index (RSI) and the inferior reduction degradation index (RDI) with increasing the ratio of pyrite cinder. For example, the fired pellets containing 70% pyrite cinder possess the higher RI of 68.89%, the better RSI of 7.59% and the lower RDI of 90.31%. The more the ratio of pyrite cinder is elevated, the more the pores are formed, which is shown in Table 4. The porosity of fired pellets can improve the RI and RSI. However, the RDI decreases with increasing the ratio of pyrite cinder, because of the lower compressive strength of fired pellets, which agrees with the result in Fig.5.
3.6 Microstructures of fired pellets
Morphology of surface of fired pellets at different pyrite cinder ratios under SEM can be found in Fig.6. The surface of fired pellets is porous when the ratio of pyrite is 40%, and some larger pores and slight cracks emerge with the increase of pyrite cinder ratio to 50%. As the ratio of pyrite cinder is elevated to 60%, some cracks are formed on the surface of fired pellets. The fired pellets of 70% pyrite cinder possess more cracks on the surface of fired pellets. The higher the ratio of pyrite cinder is given, the more the cracks are formed, which also agrees with the results of Table 4.
Table 5 Metallurgical performance of fired pellets at different pyrite cinder ratios
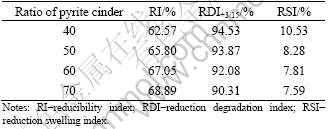
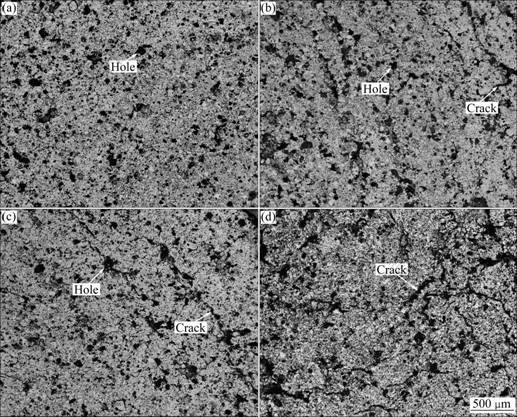
Fig.6 Surface morphologies of fired pellets at different pyrite cinder ratios under SEM: (a) 40%; (b) 50%; (c) 60%; (d) 70%
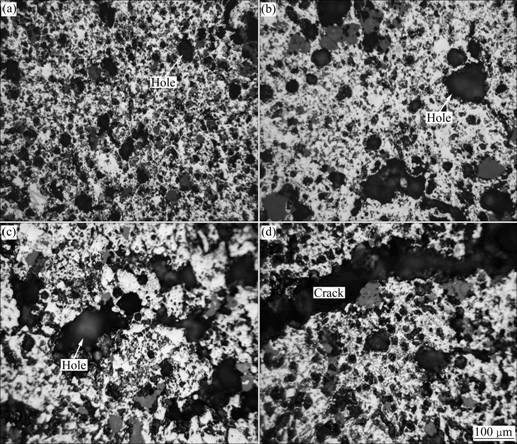
Fig.7 Inside microstructures of fired pellets at different pyrite cinder ratios under optical microscopy: (a) 40%; (b) 50%; (c) 60%; (d) 70%
Figure 7 shows the inside microstructures of fired pellets at different pyrite cinder ratios under optical microscopy. The holes and cracks in the inner of fired pellets become larger and larger when the ratio of pyrite cinder increases from 40% to 70%. The holes and cracks are obtained because the sulfur is removed from the insider of pellets to outside. The big cracks are formed with the augment of pyrite cinder ratio to 70%, which leads to the lower compressive strength of fired pellets.
4 Conclusions
1) Most sulfur exists in the form of elementary sulfur in pyrite cinder, and over 95% sulfur in raw materials is removed under the optimum firing conditions of firing at 1 230 °C for 15 min.
2) The compressive strength of fired pellets decreases, the RI and RSI become well and the RDI becomes worse when the ratio of pyrite cinder increases from 40% to 70%.
3) The porosity and microstructures of fired pellets prove that holes and cracks are formed when the sulfur is removed in the form of SO2 from inside of pellets.
References
[1] BAI Guo-hua, ZHOU Xiao-qing. Study on Pelletizing of pyrite cinder with magnetite [J]. Iron and Steel, 2009, 44(7): 7-9. (in Chinese)
[2] ZHU De-qing, LI Jian, PAN Jian. Preparation of high quality magnetite concentrate from pyrite cinder by composite pellet reduction-roasting and magnetic-separation [J]. The Chinese Journal of Nonferrous Metals, 2007, 17(4): 649-656. (in Chinese)
[3] ZENG Cai-ling. Experimental study on baking of sulphuric acid slag pellet [J]. Wisco Technology, 2006, 44(1): 29-30. (in Chinese)
[4] ZHU De-qing, LI Jian, PAN Jian. Study on pelletising of magnetite concentrate from pyrite cinder [J]. Mining and Metallurgical Engineering, 2006, 26(6): 49-52.
[5] ABDRAKHIMOV A V, ABDRAKIMOVA E S, ABDRAKHIMOV V Z. Technical properties of roof tiles made of technogenic material with pyrite cinder [J]. Glass and Ceramics, 2006, 63(3): 130-132.
[6] LIU Xin-zhong, YANG Xin-chun, DONG Feng-zhi. Researches on the balling and roasting properties of the slphuric acid dregs [J]. Metal Mine, 2002, 315(9): 51-53. (in Chinese)
[7] SUN Xiao-qing, YAO Zuo-sheng. Experimental feasibility study on production of oxidised agglomerate by utilisation of SA cinder [J]. Phosphate & Compound Fertilize, 2005, 20(6):13-15. (in Chinese)
[8] ZHU De-qing, CHEN Dong, PAN Jian. Pretreatment of pyrite cinder before pelletization by high pressure roller grinding [J]. Journal of Iron and Steel Research International, 2009, 16(s2): 345-349.
[9] YU Wei, ZHU De-qing, LI Qi-hou. Improving pelletisation of pyrite cinder by high pressure roller grinding [C]// XXV International Mineral Processing Congress. Brisbane Australia, IMPC, 2010: 213-221.
[10] HE Bin-bin, TIAN Xie-ke, SUN Yan. Recovery of iron oxide concentrate from high-sulfur and low-grade pyrite cinder using an innovative beneficiating process [J]. Hydrometallurgy, 2010, 104(2): 241-246.
[11] GU Guo-hua, WANG Hui, SUO Jun. Interfacial interaction of bio-leaching of pyrite mineral [J]. Journal of Central South University of Technology, 2008, 15(1): 49-50.
[12] BAI Guo-hua, LI Jian-chen, ZHOU Xiao-qing. Effect of damp grinding on preparation of oxidized pellet from pyrite cinders concentrates [C]// Supplemental Proceedings, Materials Processing and Properties, 139th Annual Meeting & Exhibition. Seattle, TMS, 2010: 515-523.
[13] LI Chun, LIANG Bin, ZHANG Qiang. Preparation of magnetic material of ferric from pyrite cinder [J]. Journal of rare earths, 2005, 23(2): 91-95.
[14] AIP I, DEVECI H, YAZICI E Y. Potential use of pyrite cinder as raw material in cement production: Results of industrial scale trial operations [J]. Journal of Hazardous Materials, 2009, 166(1): 144-149.
[15] CHEN Tie-jun, ZHANG Yi-min. Experiments of acid pellet using treated pyrite slag and its commercial application [J]. Research on Iron & Steel, 2005, 142(1): 1-4. (in Chinese)
(Edited by DENG Lü-xiang)
Foundation item: Project(2007k02) supported by the Technology Fund of the Land and Resources Department of Hunan Province, China
Received date: 2010-11-22; Accepted date: 2011-03-04
Corresponding author: ZHU De-qing, Professor, PhD; Tel: +86-731-88836942; E-mail: dqzhu@csu.edu.cn