
J. Cent. South Univ. (2018) 25: 1619-1627
DOI: https://doi.org/10.1007/s11771-018-3854-0

Facile synthesis of hierarchical BiOClxBr1–x solid solution with enhanced photocatalytic activity
GU Ying-ying(古映莹)1, 2, XIONG Yi-qiu(熊意球)1, 2, ZHANG Xi-xi(张茜茜)1, 2,ZHAO Li(赵莉)1, 2, ZHANG Shou-chun(张寿春)1, 2, YAN Jun(颜军)1, 2
1. School of Chemistry and Chemical Engineering, Central South University, Changsha 410083, China;
2. Hunan Provincial Key Laboratory of Efficient and Clean Utilization of Manganese Resources,Central South University, Changsha 410083, China
Central South University Press and Springer-Verlag GmbH Germany, part of Springer Nature 2018
Abstract: The hierarchical BiOClxBr1–x was synthesized by a simple solvothermal method. The samples were characterized by X-ray diffraction (XRD), field emission scanning electron microscopy (FESEM), UV-visible diffuse reflectance spectroscopy (UV-vis DRS) and Brunauer-Emmett-Teller adsorption method. Compared to pure BiOCl or BiOBr, the BiOClxBr1–x solid solution has enhanced photocatalytic degradation activity for rhodamine B. This phenomenon can be explained to the hierarchical structure, lager specific surface area and appropriate energy gap of the obtained BiOClxBr1–x solid solution. The renewability and stability of photocatalyst were determinated and a possible mechanism of photocatalytic degradation was also proposed.
Key words: bismuth oxyhalides; solid solution; hierarchical structure; photocatalytic degradation
Cite this article as: GU Ying-ying, XIONG Yi-qiu, ZHANG Xi-xi, ZHAO Li, ZHANG Shou-chun, YAN Jun. Facile synthesis of hierarchical BiOClxBr1–x solid solution with enhanced photocatalytic activity [J]. Journal of Central South University, 2018, 25(7): 1619–1627. DOI: https://doi.org/10.1007/s11771-018-3854-0.
1 Introduction
In recent years, semiconductor photocatalysis has been considered as a most promising technology to balance the problems between global serious energy and environment development [1–5]. The advantage of photocatalytic degradation is that the organics can completely decompose to CO2, water and other inorganic substances which will not induce secondary pollution [6]. Since FUJISHIMA et al [7] first reported photocatalytic splitting of water with TiO2 electrodes in 1972, numerous researchers have been focused on the synthesis of more efficient photocatalyst for various application. Unfortunately, the photocatalytic property of TiO2 has been greatly confined because TiO2 can only be activated by UV light which makes up a small portion (about 4%) in the sunlight spectrum. In order to make the best of sunlight, there are some strategies, such as microstructure modification [8], nonmetal doping [9], noble metal deposition [10], hybridization with other semiconductor [11, 12], that can be used to modify TiO2 to facilitate the absorption of visible light. Bismuth oxyhalides BiOX (X=Cl, Br, I) is a new type of semiconductor photocatalyst, which shows excellent photocatalytic performance because of their distinct intrinsic electronic structure and chemical stability [13, 14]. BiOX has a tetragonal matlockite structure consisting of [Bi2O2]2+ layers interleaved with the halogen atom layers by the Van der Waals force along the c-axis [15, 16]. The internal static electric fields are capable to polarize atoms, facilitating separation and migration of photoinduced electrons- holes pairs [17]. BiOX demonstrates excellent photocatalytic activities for various organics, and considerable effort has been devoted to preparing pure BiOX with various morphology, such as nanoparticles [18], nanobelts [19], nanosheets [20], hollow microspheres [21] and hierarchical nanoflowers [22]. Compared to individual semiconductor photocatalyst, semiconductor composites with the staggered gap, which increased light harvesting, prolonged lifetime of photoinduced electrons-holes pairs, and enhanced of chemical stability. Therefore, heterostructure hybridization is also an effective way to further improve the photocatalytic performance of BiOX, such as BiOCl/SnO2 [23], C3N4/BiOCl [24], BiOBr/ WO3 [25], BiOBr/CdS [26], BiOI/La2Ti2O7 [27] and BiOX/NaBiO3 [28], was an indispensable way to further improve the photocatalytic performance of BiOX. The formation of solid solution plays a crucial role in improving the photocatalytic performance of BiOX. Solid solution can continuously adjust the band structures of photocatalyst, therefore the absorption edge of BiOClxBr1–x may extend to the visible region [29].
There are few reports about the BiOXxY1–x (X, Y=Cl, Br, and I) solid solutions, such as BiOClxBr1–x [30], BiOClxI1–x [31], BiOBrxI1–x [32, 33]. These reports demonstrate BiOXxY1–x has superior photocatalytic performance comparing to pure that BiOX. DU et al [30] reported that the BiOClxBr1–x samples were synthesized by hydrothermal method, the BiOClxBr1–x were lamellar structure and BiOClxB1–x (Cl:Br=1) have the best photocatalytic activity. In this study, a simple solvothermal method was applied to synthesizing the three-dimensional (3D) hierarchical structure BiOClxBr1–x. The photocatalytic performance was investigated via degradation rhodamine B (RHB) under UV-vis light. The result shows that BiOClxBr1–x exhibits higher photocatalytic property than pure BiOCl or BiOBr. The structure of BiOClxBr1–x was systematically characterized. Also, the possible mechanism for photocatalytic degradation RHB was also proposed.
2 Experimental section
2.1 Synthesis of BiOClxBr1–x solid solution
All of the chemicals reagents were analytical grade (AR) and used without further purification. Deionized water was used throughout the whole experiments. The BiOClxBr1–x was synthesized via a simple solvothermal process. 4 mmol Bi(NO3)3·5H2O was dispersed into 80 mL ethylene glycol (EG) solutions, the certain stoichiometric amounts of halide source (the certain molar ratio of hexadecyl trimethyl ammonium chloride (CTAC) and cetyl trimethyl ammonium bromide (CTAB)) was added into the above solutions. Then the mixed solution was transferred into a 100 mL Teflon-lined autoclave after magnetic stirring for 1 h. Finally, the autoclave was cooled at ambient temperature after heating at 160 °C for 12 h. The final products were gathered by vacuum filtration, subsequently washed thoroughly with deionized water and absolute ethanol, and dried at 80 °C for 8 h. The obtained samples were named BiOClxBr1–x (x/1–x is refers to the molar ratio of CTAC/CTAB).
2.2 Characterization
The X-ray powder diffraction(XRD) data were analyzed on a Rigaku D/Max 2500 X-ray diffractometer with a Cu Kα radiation (λ=0.15406 nm, voltage=40 kV, current=250 mA, scanning rate=8.0° min–1). The morphologies were observed by a scanning electron microscope (FESEM, HITACHI S-4800). The specific surface was evaluated by the adsorption data in a relative pressure (P/P0) range from 0.05 to 0.3. The UV-vis diffuse reflectance spectroscopy (UV-vis DRS) were measured by a UV-visible spectrophotometer (Shimadzu UV-2600, Japan) with BaSO4 as a reference, and then transform the reflectance spectra to the absorption spectra using the Kubelka- Munk method.
2.3 Photocurrent measurements
The photocurrent responses were measured with the CHI 660E electrochemical workstation (Shanghai Chen Hua instrument Co., Ltd., China) with a standard three-electrode system. The samples were dripped onto the indium tin oxide (ITO) glass to prepared the working electrode, a platinum-plate as the counter electrode, Ag/AgCl as the reference electrode and 0.2 mol/L Na2SO4 solutions as electrolyte.
2.4 Photocatalytic activity evaluation
In the typical photocatalytic degradation process, a homemade 175 W metal halogen lamp as the UV-vis light source, with a circulating water cooling device to maintain the photocatalytic degradation temperature at the room temperature. 20 mg BiOClxBr1–x was dispersed into 100 mL RHB aqueous solution (15 mg/L) prior to the light irradiation. At the same time, the mixed solution was stirred for 30 min in the dark to achieve the adsorption/desorption equilibrium. In a certain time interval, 5 mL suspension was collected to centrifuge to get rid of the catalyst. The concentration of dye was measured (λ=554 nm) with UV-vis spectrophotometer (Shimadzu UV-2600, Japan). The RHB photocatalytic degradation rate of η was calculated followed by the following formula:
(1)
where C0 and A0 are the initial concentration and absorption spectrum value (λ=554 nm) of RHB respectively, and Ct and
At are the corresponding data after certain time under light irradiation.
The BiOClxBr1–x photocatalytic reaction kinetics of RhB was evaluated and the photocatalytic performance of BiOClxBr1–x can be described by the apparent reaction rate constant. Generally, the photocatalytic degradation data can be consistent with apparent first-order kinetics equation under low concentration [34]. The equation is described as follows:
(2)
The above C0 and C are the concentrations of RhB after photocatalytic degradation at time of 0, t, respectively, and kapp is the apparent rate constant of reaction.
3 Results and discussion
3.1 Structure and morphology analysis
The crystal structure and crystallinity of BiOClxBr1–x were determined by XRD measurements. Figure 1 shows the XRD patterns of BiOClxBr1–x. The diffraction peaks of pure BiOCl, BiOBr are corresponding to tetragonal BiOCl (JCPDS No. 06-0249) and tetragonal BiOBr (JCPDS No. 09-0393) respectively. Moreover, with the increase of Br content, the diffraction peak exhibited a tendency of shifting towards to BiOBr.
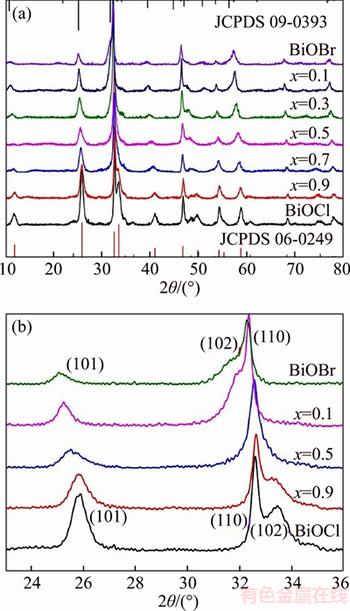
Figure 1 XRD patterns of BiOClxBr1–x (x=0, 0.1, 0.3, 0.5, 0.7, 0.9, 1) (a) and magnified XRD patterns of BiOClxBr1–x (x=0, 0.1, 0.5, 0.9, 1) (b)
The BiOClxBr1–x was similar to XRD patterns, indicating the successful synthesis of BiOClxBr1–x solid solution.
The surface morphologies of BiOClxBr1–x samples were observed by FESEM. The low- magnification FESEM image in Figure 2 displays that the sizes of BiOClxBr1–x are 400–700 nm.
The magnified FESEM images in Figure 2 indicate that all of the BiOClxBr1–x samples are hierarchical microflower, which are assembled by numerous nanosheets with a thickness of about 10 nm. These thin nanosheets stack and interact with each other, leading to the formation of three-dimensional hierarchical microflower structure. The result is consistent with our previous report [35]. Compared to pure BiOCl or BiOBr, the products were synthesized by changing the molar ratio of Cl and Br did not have a significant impact on products morphology and size. The self- assembled hierarchical microflower samples with smaller size are favorable for obtaining larger specific surface area, which can provide more active sites for photocatalytic degradation, therefore,BiOClxBr1–x are expected to show effective photocatalytic performance.
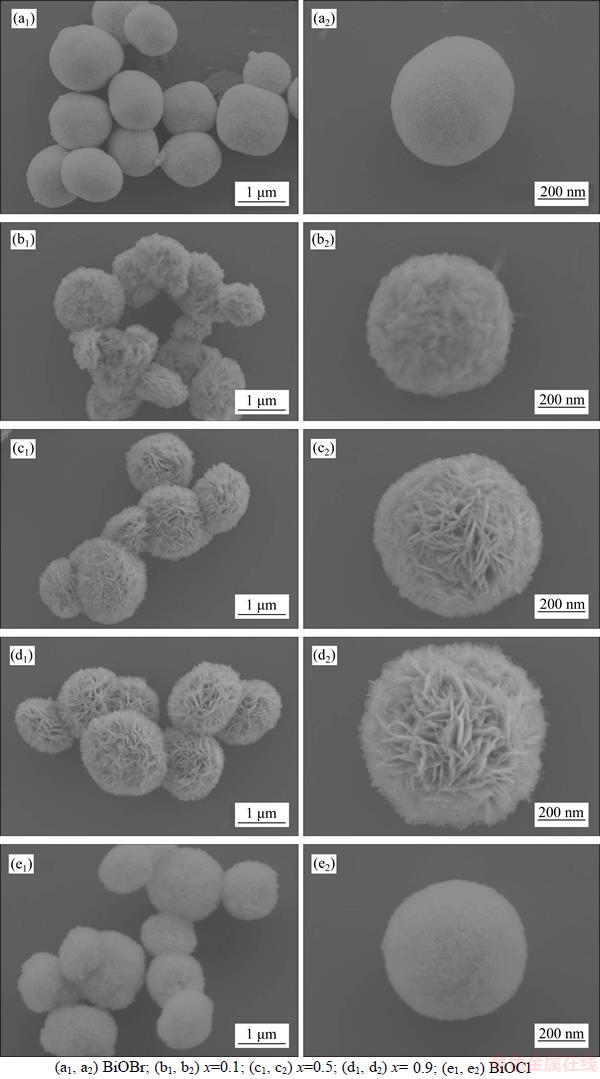
Figure 2 SEM images of BiOClxBr1–x with different values of x:
To further study the surface adsorption properties of BiOClxBr1–x, the specific surface areas of BiOClxBr1–x samples were evaluated by nitrogen adsorption analysis. The results show that the specific surface area of BiOClxBr1–x (x=0.1, 0.3, 0.5, 0.7, 0.9) solid solution ranges from 20.00 to 28.48 m2/g, while pure BiOCl, BiOBr are 17.89 and 17.55 m2/g, respectively. Obviously, the specific surface areas of BiOClxBr1-x (x=0.1, 0.3, 0.5, 0.7, 0.9) were greater than that of pure BiOCl or BiOBr. As we all know, the larger specific surface area always improves photocatalytic activity for degradation dyes, therefore, the formation of solid solution is an effective way to improve photocatalytic performance.
3.2 UV-visible diffuse reflection spectra
The optical properties of BiOClxBr1–x were detected by UV-vis absorption spectrometry.Figure 3 shows that the UV-vis absorption band edges of the BiOClxBr1–x exhibits a red shift with the increase of Br content. The energy band gaps of sample could be calculated by using the formula [36, 37]
(αhν)1/2=A(hν–Eg) (3)
where α, h, ν, A and Eg are absorption coefficient, Planck constant, light frequency, a constant and band gap energy respectively. Moreover, the plot of (Ahν)1/2 vs hν could be used to estimate the band gaps of sample. The band gaps of BiOClxBr1–x (x=0, 0.1, 0.3, 0.5, 0.7, 0.9, 1) are approximately 2.58, 2.66, 2.72, 2.83, 2.97, 3.10 and 3.23 eV. It is demonstrated that the different molar ratio of Cl/Br can tune the band gaps of BiOClxBr1–x continuously. The BiOClxBr1–x with the suitable band gaps, which could be endowed with strong visible light absorption and enhanced photocatalytic performance.
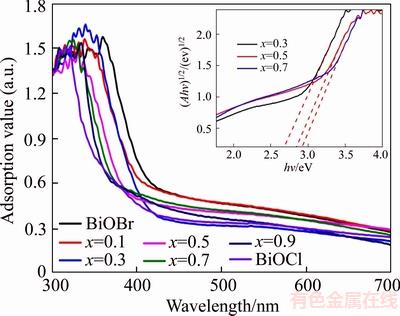
Figure 3 UV-visible diffuse reflectance spectroscopy (DRS) and plot of (Ahν)1/2 vs hν with BiOClxBr1–x
3.3 Photocatalytic activity
The photocatalytic activities of the BiOClxBr1–x were measured by photocatalytic degradation RHB under UV-vis light. As is shown in Figure 4(a), the BiOClxBr1–x demonstrated excellent photocatalytic performance after 100 min UV-vis light irradiation and the BiOCl0.9Br0.1 presented the highest photocatalytic performance and the RHB were completely removed after 60 min UV-vis light irradiation. The remove rate of RHB for BiOClxBr1–x (x=0, 0.1, 0.3, 0.5, 0.7, 0.9, 1) are 17.07%, 22.08%, 37.50%, 46.58%, 50.67%, 44.83% and 32.08% respectively after the adsorption/desorption equilibrium, and the result were in accordance with the specific surface area of BiOClxBr1–x.
As is shown in Figure 4(b), the curve of ln(C/C0) has a good linear correlation with the time of photocatalytic degradation (R2>0.98), the result indicates that the photocatalytic degradation of RHB with BiOClxBr1–x consistent with pseudo-first order reaction. The reaction rate constant for BiOClxBr1–x (x=0, 0.1, 0.3, 0.5, 0.7, 0.9, 1) are 0.0013, 0.0027, 0.0122, 0.0157, 0.0451, 0.0671 and 0.0255 min–1, respectively. Obviously, the reaction rate constant of BiOCl0.9Br0.1 is greater than those of pure BiOCl, BiOBr and other BiOClxBr1–x. Therefore, the formation of solid solution can effectively improve the photocatalytic performance of the photocatalyst. In addition, the result is consistent with the conclusion of photocatalytic degradation.
3.4 Photocurrent analysis
Photocurrent measurements were performed to further investigate photocatalytic performance. Generally, the stronger photocurrent response suggests effective separation of photo-generated electrons and holes pairs. As is shown in Figure 5, all the BiOClxBr1–x shows good photocurrent responses. When the light irradiation is turned on, the photocurrent increased drastically, while the photocurrent decreased quickly to initial value in the absence of light irradiation. In addition, the BiOCl0.9Br0.1 exhibited the greatest photocurrent responses compared with pure BiOCl or BiOBr. This indicates that BiOCl0.9Br0.1 can separate the photon-generated carrier effectively which is expected to improve the photocatalytic performance of photocatalyst.
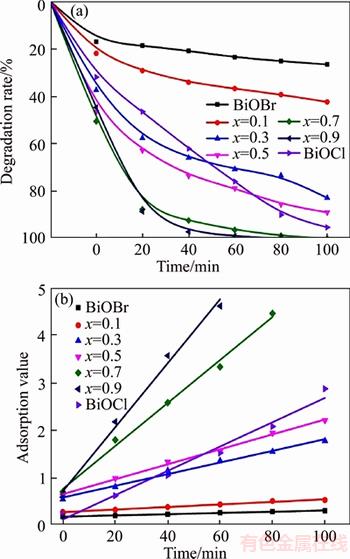
Figure 4 Photocatalytic degradation of RhB with BiOClxBr1–x (a) and pseudo-first order reaction kinetic linear curves (b)
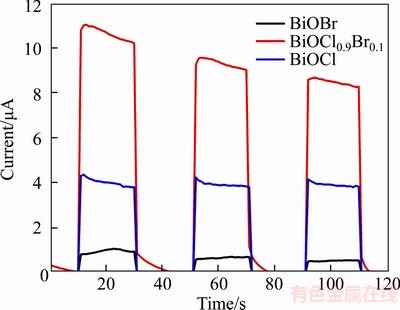
Figure 5 Photocurrents responses of BiOBr, BiOCl0.9Br0.1 and BiOCl
3.5 Possible mechanism of photocatalytic degradation
Electron (e–), hole (h+), hydroxyl radicals (·OH) and superoxide radicals (·O2–) are the primary active substances in the photocatalytic degradation of dyes [38, 39]. In this experiment, we used isopropanol (IPA), benzoquinone (BQ), potassium bromate (KBrO3) and ammonium oxalate (AO) as the scavengers to capture ·OH, ·O2–, e– and h+ respectively in order to figure out how the active substance affects the photocatalytic performance.
Figure 6 shows that the degradation rate for RHB is greatly restrained when BQ was used to be the active radical trapping agent, indicating that ·O2– is supposed to be the main active material for the photocatalytic degradation of RHB. In addition, the photocatalytic activity decreased rapidly in the presence of AO which suggests that h+ is a main active material for photocatalytic degradation process. The degradation rate was inhibited with the addition of IPA, which suggests ·OH also an active material for photocatalytic degradation. However, the addition of KBrO3 can improve the photocatalytic performance which can be ascribed to KBrO3 that can capture e– and restrain the recombination of h+ and e–.
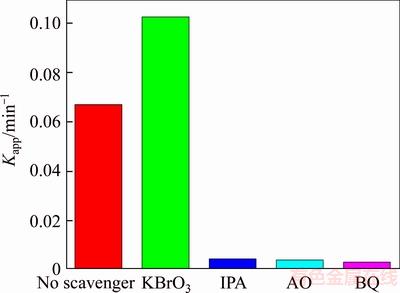
Figure 6 Active radical detection experiment for degradation RhB with BiOCl0.9Br0.1
The above results suggest that h+, ·OH and ·O2– are the dominant active materials in the photocatalytic degradation of RhB with BiOCl0.9Br0.1.
The renewability and stability of photocatalyst play an important role in actual applications. In this experiment, the BiOCl0.9Br0.1 was used to photocatalytic degradation RHB four times. As is shown in Figure 7, after four repeated runs, the degradation rate of RHB remain up to 96.04% after 100 min irradiation.
Moreover, Figure 8 shows that the cyclic photocatalytic experiment does not change the crystal structure of BiOCl0.9Br0.1. The results manifest that the BiOCl0.9Br0.1 with high stability and enduring high efficiency photocatalytic performance is the crucial for practical application in the future.
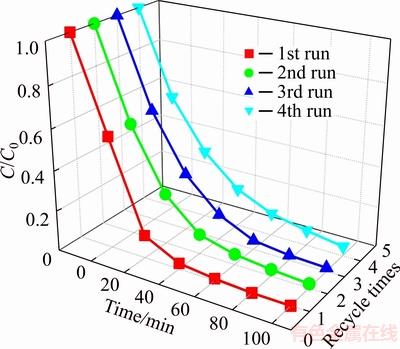
Figure 7 Recycle performance of BiOCl0.9Br0.1
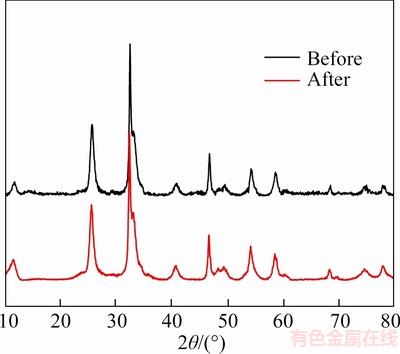
Figure 8 XRD patterns of BiOCl0.9Br0.1 before and after cyclic photocatalytic experiment
According to the above, the possible mechanism photocatalytic degradation was proposed in the Figure 9. When the BiOClxBr1–x were exposed to the UV-vis light irradiation, the excited e– were in conduction band (CB) and leaving the h+ in the valence band (VB) of BiOClxBr1–x. As is shown in the Figure 9, the excited e– in the CB of BiOBr was transferred to the CB of BiOCl quickly. At the same time, the h+ in the VB of BiOCl was transferred to the CB of BiOBr. The photon-generated e– and h+ is easier migrated to the surface of the photocatalyst subsequently, leading to a series of redox reaction for photocatalytic degradation. On the one hand, the h+ can oxidized H2O or OH– to ·OH in aqueous solution [40], the dissolved oxygen (O2) was absorbed by the surface of photocatalyst and reactive with e– to produce ·O2– [41]. On the other hand, the dye sensitization plays another important role in the photocatalytic degradation of RHB. The RhB molecules were excited by UV-vis light radiation and inject the excited e– into the CB of BiOClxBr1–x followed by a series of redox reactions.
In conclusion, the numerous nanosheets self- assembled hierarchical microspheres with the lager specific surface, suitable band gaps effective and efficiency separation of photo-generated carriers which is favorable for photocatalytic degradation. In addition, the dye sensitization can also improve the photocatalytic efficiency of BiOClxBr1–x.
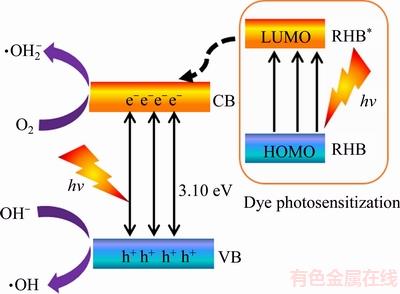
Figure 9 Mechanism of photocatalytic degradation of RHB with BiOCl0.9Br0.1
4 Conclusions
A simple solvothermal method was used to synthesize the hierarchical BiOClxBr1–x. The BiOClxBr1–x hierarchical microflower was assembled by numerous nanosheets. The BiOClxBr1–x solid solution has enhanced photocatalytic activity for rhodamine B compared with pure BiOCl or BiOBr and the BiOCl0.9Br0.1 presents the highest photocatalytic performance. The result was ascribed to the BiOClxBr1–x with hierarchical structure, larger specific surface area and appropriate energy gap. It exhibited the BiOCl0.9Br0.1 with high chemical stability and enduring high efficiency photocatalytic performance, which is crucial for practical application in the future.
References
[1] LI Xin, YU Jia-guo, LOW Jiang-xiang, FANG Yue-ping, XIAO Jing, CHEN Xiao-bo. Engineering heterogeneous semiconductors for solar water splitting [J]. Journal of Materials Chemistry A, 2015, 3(6): 2485–2534.
[2] YUAN Yu-peng, RUAN Lin-wei, BARBER J. Hetero- nanostructured suspended photocatalysts for solar-to-fuel conversion [J]. Energy & Environmental Science, 2014, 7(12): 3934–3951.
[3] KUDO A, MISEKI Y. Heterogeneous photocatalyst materials for water splitting [J]. Chemical Society Reviews, 2009, 38(1): 253–278.
[4] CHONG M N, JIN B, CHOW C W. Recent developments in photocatalytic water treatment technology: A review [J]. Water Research, 2010, 44(10): 2997–3027.
[5] CHEN Xiao-bo, SHEN Shao-hua, GUO Lin-jie. Semiconductor-based photocatalytic hydrogen generation [J]. Chemical Reviews, 2010, 110(11): 6503–6570.
[6] CHEN Cheng-chen, MA Wang-hong, ZHAO Jin-cai. Semiconductor-mediated photodegradation of pollutants under visible-light irradiation [J]. Chemical Society Reviews, 2010, 39(11): 4206–4219.
[7] FUJISHIMA A, HONDA K. Electrochemical photolysis of water at a semiconductor electrode [J]. Nature, 1972, 238(5358): 37–38.
[8] CAO Yin-hui, LI Qiu-ye, LI Chen, LI Li-jun, YANG Jian-jun. Surface heterojunction between (001) and (101) facets of ultrafine anatase TiO2 nanocrystals for highly efficient photoreduction CO2 to CH4 [J]. Applied Catalysis B: Environmental, 2016, 198: 378–388.
[9] LIN Y, HSUEH H, CHANG C. The visible light-driven photodegradation of dimethyl sulfide on S-doped TiO2: Characterization, kinetics, and reaction pathways [J]. Applied Catalysis B: Environmental, 2016, 199: 1–10.
[10] FUJIWARA K, M LLER U, PRATSINIS S E. Pd subnano-clusters on TiO2 for solar-light removal of NO [J]. ACS Catalysis, 2016, 6(3): 1887–1893.
[11] GAO You-liang, CHEN Qi-yuan, TONG Hai-xia, HU Hui-ping, QIAN Dong, YANG Ya-hui, ZHOU Jian-liang. Preparation of TiO2 photocatalyst loaded with V2O5 for O2 evolution [J]. Journal of Central South University, 2009, 16(6): 919–925.
[12] TONG Hai-xia, CHEN Qi-yuan, HU Hui-ping, YIN Zhou-lan. Light energy conversion device for photocatalyst 2.0% WO3-TiO2 with oxygen vacancies for water splitting [J]. Journal of Central South University of Technology, 2010, 17: 943–946.
[13] YE Li-qun, SU Yu-rong, JIN Xiao-li, XIE Hai-quan. Recent advances in BiOX (X=Cl, Br and I) photocatalysts: Synthesis, modification, facet effects and mechanisms [J]. Environmental Science: Nano, 2014, 1(2): 90–112.
[14] CHENG He-feng, HUANG Bai-biao, DAI Ying. Engineering BiOX (X=Cl, Br, I) nanostructures for highly efficient photocatalytic applications [J]. Nanoscale, 2014, 6(4): 2009–2026.
[15] GANOSE A M, CUFF M, BUTLER K T. Interplay of orbital and relativistic effects in bismuth oxyhalides: BiOF, BiOCl, BiOBr, and BiOI [J]. Chemistry of Materials, 2016, 28(7): 1980–1984.
[16] HENLE J, SIMON P, FRENZEL A, SCHOLZ S, KASKEL S. Nanosized BiOX (X=Cl, Br, I) particles synthesized in reverse microemulsions [J]. Chemistry of Materials, 2007, 19(3): 366–373.
[17] HUANG Hong-wei, HE Ying, LIN Zhe-shuai, KANG Lei, ZHANG Yi-he. Two novel Bi-Based borate photocatalysts: crystal structure, electronic structure, photoelectrochemical properties, and photocatalytic activity under simulated solar light irradiation [J]. The Journal of Physical Chemistry C, 2013, 117(44): 22986–22994.
[18] KANDANAPITIYE M S, GAO M, MOLTER J. Synthesis, characterization, and X-ray attenuation properties of ultrasmall BiOI nanoparticles: Toward renal clearable particulate CT contrast agents [J]. Inorganic Chemistry, 2014, 53(19): 10189–10194.
[19] LI Hai-ping, LIU Jia-ying, HU Ting-xia, DU Na, HOU Wan-guo. Synthesis of belt-like BiOBr hierarchical nanostructure with high photocatalytic performance [J]. Materials Research Bulletin, 2016, 77: 171–177.
[20] GUAN Mei-li, XIAO Chong, ZHANG Jie, FAN Shao-jun, ZHOU Min, XIE Yi. Vacancy associates promoting solar-driven photocatalytic activity of ultrathin bismuth oxychloride nanosheets [J]. Journal of the American Chemical Society, 2013, 135(28): 10411–10417.
[21] CUI Peng-chen, WANG Jia-li, WANG Zu-min, CHEN Jun, YU Ran-bo. Bismuth oxychloride hollow microspheres with high visible light photocatalytic activity [J]. Nano Research, 2016, 9(3): 593–601.
[22] JU Peng, XIANG Yu-hui, XIANG Zhen-bo, WANG Ming, HAI Xiu-xun. BiOI hierarchical nanoflowers as novel robust peroxidase mimetics for colorimetric detection of H2O2 [J]. RSC Advances, 2016, 6(21): 17483–17493.
[23] SUN Mei-li, ZHAO Qi-hai, DU Chun-fang, LIU Zhi-liang. Enhanced visible light photocatalytic activity in BiOCl/SnO2. heterojunction of two wide band-gap semiconductors [J]. RSC Advances, 2015, 5(29): 22740–22752.
[24] LEI Li-wen, JIN Hui-hui, ZHANG Qi, XU Jun, FU Zheng-yi. A novel enhanced visible-light-driven photocatalyst via hybridization of nanosized BiOCl and graphitic C3N4 [J]. Dalton Transactions, 2015, 44(2): 795–803.
[25] ZHANG Jun-lei, ZHANG Li-sha, SHEN Xiao-feng, YU Peng-fei, LIU Jian-she. Synthesis of BiOBr/WO3 p-n heterojunctions with enhanced visible light photocatalytic activity [J]. Cryst Eng Comm, 2016, 18(21): 3856–3865.
[26] GUO Yu-xi, HUANG Hong-wei, HE Ying, TIAN Na, ZHANG Tie-rui, AN Qi, ZHANG Yi-he. In situ crystallization for fabrication of a core-satellite structured BiOBr-CdS heterostructure with excellent visible-light- responsive photoreactivity [J]. Nanoscale, 2015, 7(27): 11702–11711.
[27] AO Yan-hui, WANG Ke-dan, WANG Pei-fang, WANG Cao, HOU Jun. Fabrication of novel p-n heterojunction BiOI/La2Ti2O7 composite photocatalysts for enhanced photocatalytic performance under visible light irradiation [J]. Dalton Transactions, 2016, 45(19): 7986–7997.
[28] JI Lei, WANG Hao-ren, YU Rui-min. Heterogeneous photocatalysts BiOX/NaBiO3 (X=Cl, Br, I): Photo-generated charge carriers transfer property and enhanced photocatalytic activity [J]. Chemical Physics, 2016, 478: 14–22.
[29] ZHAO Zong-yan, LIU Qing-lu DAI Wen-wu. Structural, electronic, and optical properties of BiOX1–xYx (X, Y=F, Cl, Br, and I) solid solutions from DFT [J]. Scientific Reports, 2016, 6: 31449–31460.
[30] DU Dan-dan, LI Wen-jun, CHEN Sha-sha, YOU Jin-mao, KONG De-sheng. Synergistic degradation of rhodamine B on BiOClxBr1–x sheets by combined photosensitization and photocatalysis under visible light irradiation [J]. New Journal of Chemistry, 2015, 39(4): 3129–3136.
[31] ZHANG Yang-yang, SUN Xiu-guo, LI Yan-ting. Preparation and characterization of bifunctional BiOClxIy solid solutions with excellent adsorption and photocatalytic abilities for removal of organic dye [J]. Materials Science in Semiconductor Processing, 2016, 41: 193–199.
[32] HUANG Hong-wei, LI Xiao-wei, HAN Xu, TIAN Na. Moderate band-gap-broadening induced high separation of electron-hole pairs in Br substituted BiOI: A combined experimental and theoretical investigation [J]. Physical Chemistry Chemical Physics, 2015, 17(5): 3673–3679.
[33] ZHANG Xing, WANG Chu-ya, Wang Li-Wei. WANG Wei-kang, YU Han-qing. Fabrication of BiOBrxI1-x photocatalysts with tunable visible light catalytic activity by modulating band structures [J]. Scientific Reports, 2016, 6: 22800–22809.
[34] ZHOU Xue-mei, LIU Gang, YU Jia-guo. Surface plasmon resonance-mediated photocatalysis by noble metal-based composites under visible light [J]. Journal of Materials Chemistry, 2012, 22(40): 21337–21354.
[35] GU Ying-ying, ZHAO Li, YANG Ming-yang, XIONG Yi-qiu, YAN Jun. Preparation and characterization of highly photocatalytic active hierarchical BiOX(X=Cl, Br, I) microflowers for rhodamine B degradation with kinetic modelling studies [J]. Journal of Central South University, 2017, 24(4): 754–765.
[36] PEZ R L, MEZ R G. Band-gap energy estimation from diffuse reflectance measurements on sol-gel and commercial TiO2: a comparative study [J]. Journal of Sol-Gel Science and Technology, 2011, 61(1): 1–7.
[37] ZHANG Ke-lei, LIU Cun-ming, HUANG Fu-qiang, ZHENG Chong. Study of the electronic structure and photocatalytic activity of the BiOCl photocatalyst [J]. Applied Catalysis B: Environmental, 2006, 68(3, 4): 125–129.
[38] DIMITRIJEVIC N M, VIJAYAN B K, POLUEKTOV O G. Role of water and carbonates in photocatalytic transformation of CO2 to CH4 on titania [J]. Journal of the American Chemical Society, 2011, 133(11): 3964–3971.
[39] XIN Xiao, RONG HAO, MIN Liang. One-pot solvothermal synthesis of three-dimensional (3D) BiOI/BiOCl composites with enhanced visible-light photocatalytic activities for the degradation of bisphenol-A [J]. Journal of Hazardous Materials, 2012, 233: 122–130.
[40] ZHANG Nan, LIU Si-qi, FU Xiang-zhi, XU Yi-jun. Synthesis of M@TiO2 (M=Au, Pd, Pt) core–shell nanocomposites with tunable photoreactivity [J]. Journal of Physical Chemistry C, 2011, 115(18): 9136–9145.
[41] YANG Juan, CHEN Chun-cheng, JI Hong-wei, MA Wan-hong, ZHAO Jin-cai. Mechanism of TiO2-Assisted photocatalytic degradation of dyes under visible irradiation:Photoelectrocatalytic study by TiO2-film electrodes [J]. Journal of Physical Chemistry B, 2005, 109(46): 21900– 21907.
(Edited by HE Yun-bin)
中文导读
层次结构卤氧化铋的合成及其光催化活性研究
摘要:通过简单的溶剂热法合成了层次结构的卤氧化铋,采用XRD、FESEM、UV-vis DRS、BET分别对产品的物相、形貌、光学性能以及比表面积进行了分析。相比于单组分的BiOCl 和BiOBr,BiOClxBr1–x 固溶体由于其具有精细的层次结构、更大的比表面积以及合适的能带宽度,因此对罗丹明具有更高的光催化活性。同时,本文探究了光催化剂的循环可再生性和稳定性并提出了可能的光催化反应机理。
关键词:卤氧化铋;固溶体;层次结构;光催化降解
Foundation item: Project(2016TP1007) supported by the Hunan Provincial Science and Technology Plan Project, China
Received date: 2017-02-17; Accepted date: 2017-03-28
Corresponding author: YAN Jun, PhD, Professor; Tel: +86–731-88879616; E-mail: yanjun@csu.edu.cn; ORCID: 0000-0002-6158-0614