
Grain size refinement of magnesium composite alloys by addition of B2O3
BU Le-ping(卜乐平)1,2, S. TANAKA1, M. TSUSHIDA3, S. ANDO3, H. TONDA3
1. Graduate School of Science and Technology,Kumamoto University, 2-39-1 Kurokami,
Kumamoto 860-8555, Japan;
2. Machinery and Electronic Engineering Institute, Inner Mongolia University of Agriculture, Hohhot 010018, China;
3. Department of materials Science and Engineering, Faculty of Engineering, Kumamoyo University, 2-39-1
Kurokami, Kumamoto 860-8555, Japan
Received 28 July 2006; accepted 15 September 2006
Abstract: The high performance magnesium alloy was investigated by adding B2O3 in magnesium and magnesium alloys. Experiments include adding B2O3 in Mg, Mg-Al and Mg-RE alloys, respectively, studying the effects of B2O3 on the microstructure, were studied measuring the change of grain size and microhardness of the materials, discussing the change of grain size, morphology and distribution. The results show that adding 3% or 6%(mass fraction) B2O3 in Mg can bring twinning in Mg, adding B2O3 in Mg-Al alloys and Mg-RE alloys can refine the alloy grain size. Adding 3%B2O3 in Mg-6Al alloys can refine the average grain size by about 5 μm, with the average hardness increased by 13.3% (53.3-60.4 HV0.03); adding 6%B2O3 in Mg-6Al alloys can refine the average grain size by about 13 μm, with the average hardness increased by 15.8% (53.3-61.73 HV0.03); adding 3% and 6%B2O3 into Mg-6RE alloys can refine the grain size by about 5 and 9 μm, respectively, with the average hardness decreased to HV0.03 64.66 and HV0.03 57.86, respectively from HV0.03 88.57. In the Mg-6Al alloy the content of aluminum is increased, while in the Mg-6RE alloy the content of oxygen is decreased. It can be concluded that it is beneficial to develop Mg-Al-B-O particle reinforce composite alloys , and it is feasible to develop nanometer crystallization of block material by Mg-B-O-RE.
Key words: magnesium alloys; grain size refinement; composite alloys; B2O3; particle reinforce; compo-casting
1 Introduction
In recent years, magnesium and magnesium alloys have attracted greater attention for their unique advantages. With such characteristics as lightmass, low-gravity and high-strength characteristics, etc, Mg and Mg alloys are utilized in a wider range of fields. At present, the development and utilization of Mg alloy play an increasingly important part in such fields as electronics, transportation and aviation and aerospace. Mg and composite alloys with high properties draw increasing interest today. Accordingly, the purpose of this research is to find ways to improve or enhance the mechanical properties of the Mg alloys, providing some preliminary materials for the further study of a fodder merely.
As the lightest structural alloys, magnesium alloys offer good combination of mechanical properties, corrosion resistance, cast ability and heat treatment properties[1-2]. Ceramic particle/short fiber reinforced magnesium alloy matrix composites seem to be a conventional combining technique. Casting process is the useful technique for the preparation of lightmass alloy matrix composites because of easy treatment and high performance. Compo-casting process is the stir casting with reinforcement under the semi-solid state, which has low combustibility and oxidation. As magnesium alloy has high activity, compo-casting is a suitable process of magnesium alloy matrix composites. On the other hand, the kinds of reinforcement affect the mechanical properties of the composites strongly[3-4]. In this research, the same compo-casting process was adopted on electric resistance furnace, adding B2O3 into magnesium and Mg-RE and Mg-Al alloys.
2 Experimental
The chemical compositions of Mg, Al, RE (MM) and the studied B2O3 are listed in Tables 1-4.
Table 1 Chemical composition of magnesium(mass fraction, %)

Table 2 Chemical composition of aluminum (mass fraction, %)

Table 3 Chemical composition of RE (mass fraction, %)

Table 4 Chemical composition of B2O3 (mass fraction, %)

The experimental device was shown in Fig.1. A stirrer (SUS304 make) was installed on the top of the electric resistance furnace, which can move up and down(300-500 mm), and turn around (0-1 600 r/min). The crucible was made of steel tube of SS41 (120 mm extent, inside diameter 62.5 mm, wall thickness 5 mm) fused with steel plank of SS41 (5 mm ply). The crucible was put in the electric resistance furnace and argon was applied into the crucible.
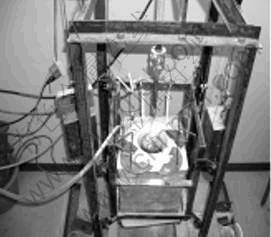
Fig.1 Photo of test device
Two semicircular covers of electric resistance furnace and two semicircular covers of crucible are provided, all made of steel plank of SS41 (5 mm ply) so that the stirrer can move up and down and the experimental materials can be added conveniently. The thermocouple was installed along side both the electric resistance furnace and the crucible. The steel model was adopted (inside diameter 25 mm, inside height 140 mm).
The experimental procedures are as follows: 1) put the selected experimental materials (Mg, Mg-RE and Mg-Al) in the crucible, respectively; 2) lowered the
paddle type blade of the stirrer until it approached the surface of materials in the crucible; 3) placed the two semicircular covers of crucible and the two semicircular covers of electric resistance furnace, and applied argon into the crucible (0.02 mL/min); 4) heated up the electric resistance furnace, temperature of the test materials in the crucible must reach 953 K within 35-45 min when heating; 5) kept the temperature at 953 K for 1 min, then added B2O3 particle promptly, this operation must be completed within around 3 min; 6) after adding B2O3, lowered the paddle type blade of the stirrer until it was about 3 mm above the inside bottom of the crucible, started the stirrer and maintained stirring by paddle type blade at 800 r/min for 20 min until the molten or semi-solid composite was compounded; 7) turned off the stirrer, the pouring temperature for the steel mould was about 1 053 K; 8) examined the specimen by optical microscope to observe the grain size change caused by B2O3 addition, measured the hardness and micro-hardness of the alloys.
3 Results and analysis
The results of XRD analysis show the main oxide and boride in Mg-xB2O3 are MgO and boron. Fig.2 shows the microstructures of Mg-xB2O3. When 2.5%, 3%, 6% and 12%B2O3 is added respectively into Mg metal, many twins are produced in each Mg metal. Many ribbon shaped straps are twins, which shows that the grain size in certain Mg alloys may become smaller after adding B2O3.
The results of XRD analysis show the main intermetallic oxide and boride in Mg-6Al-xB2O3 are Mg17Al12, MgO and boron, respectively. Fig.3 shows the microstructures of Mg-6Al-xB2O3. The horizontal direction of Fig.3 from left to right shows the microstructures of the same sample at 5 and 10 mm from the center, respectively, the vertical direction of Fig.3 from top to bottom shows the microstructures of the column sample, top of Mg-6Al, bottom of Mg-6Al, top of Mg-6Al-3B2O3, bottom of Mg-6Al-3B2O3, top of Mg-6Al-6B2O3 and bottom of Mg-6Al-6B2O3, respectively. From Fig.3, it is not difficult to notice significant changes in the grain size (size, morphology and distribution) when 0, 3% and 6% B2O3 is added respectively into Mg-6Al alloy.
The results of XRD analysis show the main intermetallic oxide and boride in Mg-6RE-xB2O3 are Mg12RE, La2O3, MgO, LaB4 and LaB6, respectively. Fig.4 show the microstructures of Mg-6RE-xB2O3. Fig.4 shows the same part of the sample as in Fig.3. it is obvious to see that significant changes have occurred in the grain size (size, morphology and distribution) when 0, 3% and 6% B2O3 is added respectively into Mg-6RE alloy.
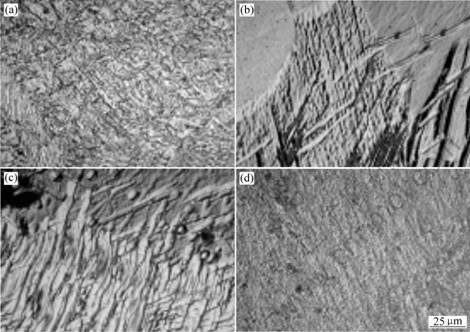
Fig.2 Microstructures of Mg-xB2O3: (a) Mg-2.5B2O3; (b) Mg-3B2O3; (c) Mg-6B2O3; (d) Mg-12B2O3
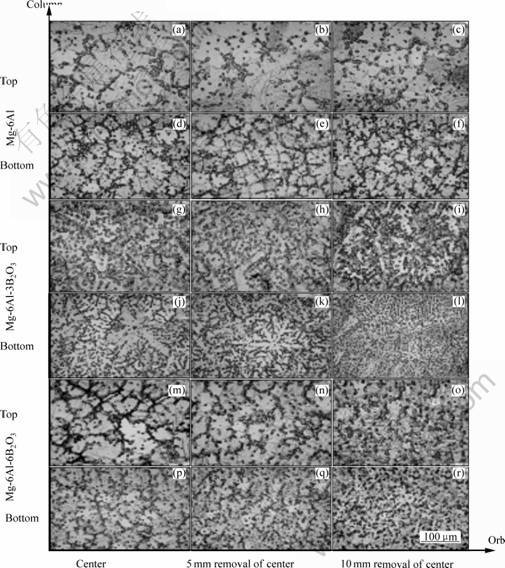
Fig.3 Microstructures of Mg-6Al-xB2O3
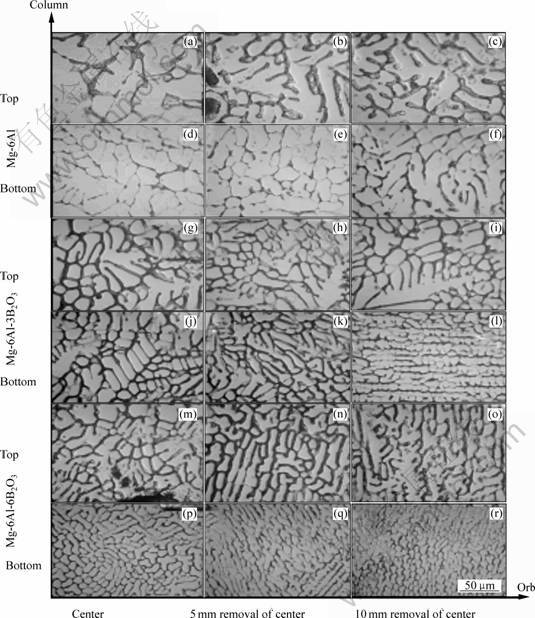
Fig.4 Microstructures of Mg-6RE-xB2O3
When 2.5%, 3%, 6% and 12% B2O3 was added respectively into Mg, many twins are produced in each alloy. Fig.5 shows the change of grain size in Mg-6Al and Mg-6RE alloys when B2O3 is added. Figs.5(a)-(c) show the change of Mg-6Al-xB2O3 grain size under different positions, respectively, and Figs.5(d)-(f) show the change of Mg-6RE-xB2O3 grain size under different positions, respectively. Excluding the effects of diathermancy, cooling rate and cooling mode, it can be seen that by adding 3% and 6% B2O3 respectively, the average grain size in Mg-6Al alloys is decreased by 5 and 13 μm, and by adding 3% and 6%B2O3, respectively, the average grain size in Mg-RE alloys is decreased by 5 and 8 μm.
A close observation of Figs.2-4 shows the change of grain morphology and distribution in sample of Mg-xB2O3, Mg-6Al-xB2O3 and Mg-6RE-xB2O3, respectively. When B2O3 is added in pure Mg, many twins are produced in its organizations. When 3% and 6%B2O3 are added into pure Mg, the ribbon shaped strap of twins is thicker and bigger. When 3%B2O3 is added into Mg-6Al alloy, the grain form turns into spinal projection shape from that of polyhedron. The exiguous intermetallic, oxide, boron and boride granule are dispersions distributed in matrix and crystal bound, respectively. When 6%B2O3 is added into the alloy, the grain form is polyhedron, but the diameter of polyhedron decreases. The size of the intermetallic, oxide, boron and boride granule is bigger than that when 3%B2O3 is added into the alloy.
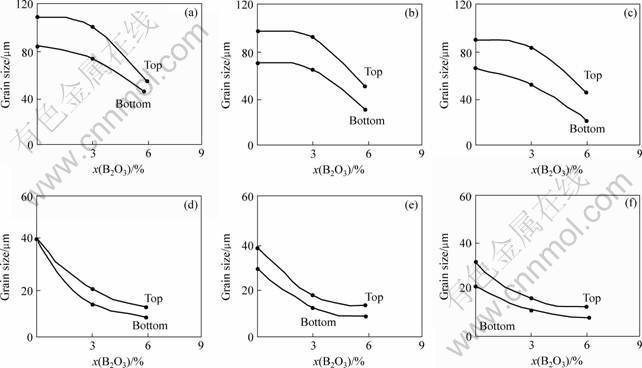
Fig.5 Change of grain size: (a) Mg-6Al-xB2O3(Center); (b) Mg-6Al-xB2O3(5 mm removal from center); (c) Mg-6Al-xB2O3(10 mm removal from center); (d) Mg-6RE-xB2O3(Center); (e) Mg-6RE-xB2O3(5 mm removal from center); (f) Mg-6Al-xB2O3(10 mm removal from center)
The grain form of Mg-6RE alloy is dendrites. When 3%B2O3 is added into the alloy, the grain form is dendrites(decreased, diminished) + spinal projection
(a few)+anomalous ball. The exiguous intermetallic, oxide, boron and boride granule are dispersions distributed in matrix and crystal bound, respectively. When 6%B2O3 is added into the alloy, the grain form is dendrites(decreased more, diminished)+spinal projection (mass) +anomalous ball (mass, diminished). The mighty exiguous intermetallic, oxide, boron and boride granule are dispersions distributed in matrix and crystal bound, respectively.
Fig.6 shows the micro-hardness of samples Mg-xB2O3, Mg-6Al-xB2O3 and Mg-6RE-xB2O3. Adding B2O3 into pure Mg does not cause significant change in the hardness. In Mg-Al system, the average hardness is increased by 13.3% (HV0.03 53.3-60.4) by adding of 3%B2O3. But by adding 6%B2O3, the average hardness is increased only by 15.8% (HV0.03 53.3-61.73). In Mg-RE system, a new phenomenon arises that the average hardness decreases to HV0.03 64.66 and 57.86 from HV0.03 88.57 after adding 3% or 6%B2O3, respectively. In the Mg, Mg-6RE and Mg-6Al alloys, Mg-6RE displays the largest difference in hardness, while Mg-6Al the smallest. After adding 3% or 6% B2O3 into alloys respectively, the hardness difference of Mg-6Al-xB2O3 is greater than that of Mg-6RE-xB2O3. This result suggests that the content of Al will be increased by adding B2O3 in Mg-Al alloy. It can be expected that adding 2%- 3%B2O3 into Mg-9-13Al alloys can reduce the grain size, and greatly increase its hardness.
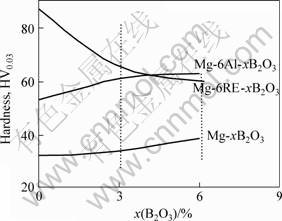
Fig.6 Change of microhardness with content of B2O3 in Mg composite alloys
4 Discussion
Mg-3%(or 6%) B2O3 is metal matrix particle reinforce composite material. The primary microstructures are Mg (α) matrix+MgO (granule)+B (granule). The slight increase of its hardness is caused by twins, MgO and B. Because the twin bound is usually an interface of a simpler kind in crystal inside structure, the interface energy of twins is lower than the crystal bound energy, only about 0.1-0.5 times as high compared to the crystal bound energy. The micro-hardness of MgO and B granule is greater than that of Mg(α) matrix, but MgO and B granule are distributed in crystal bound of Mg(α) matrix, thus lessening to some extent aberration of matrix crystal bound. Thereby, adding B2O3 into pure Mg can not cause significant change in hardness.
Mg-6Al-3% or 6%B2O3 is alloy matrix particle reinforce composite material. The primary micro- structures are Mg(α) matrix+Mg17Al12(β) intermetallic+ MgO granule+B granule. The increase of hardness of Mg-6Al-3B2O3 is greater. This is mainly because the grain size of matrix is decreased, the grain form is spinal projection, and a larger number of exiguous intermetallic, oxide, boron and boride granule are dispersions distributed on matrix, respectively. The increase of hardness of Mg-6Al-6B2O3 is smaller. This is mainly because although the grain size of matrix is decreased, the grain form is polyhedron, when the unknown MgAlBO granule content increases, the content of Mg17Al12(β) intermetallic decreases, and the larger intermetallic, thicker oxide, boron and boride granule are dispersions distributed in matrix and crystal bound, respectively. This result suggests again that the content of Al is increased when adding B2O3 in Mg-6Al alloys.
Mg-6RE-3% or 6%B2O3 is alloy matrix particle reinforce composite material. The primary micros- tructures are Mg(α) matrix+Mg12RE(β) intermetallic+ La2O3 granule+MgO granule+LaB4 and LaB6 granule. The hardness of Mg-6RE-3B2O3 is decreased. This is mainly because though the grain size of matrix is decreased, the grain form is dendrites (decreased, diminished)+spinal projection (a few) +anomalous ball, and when the contents of LaB4, LaB6 and La2O3 granule increase, the content of Mg12RE(β) intermetallic decreases, and a greater number of the exiguous intermetallic, oxide, boron and boride granule are dispersions distributed on the crystal bound respectively, thus relatively lessening the aberration of matrix crystal bound. Meanwhile, when the granule shaped Mg12RE, La2O3, MgO, LaB4 and LaB6 come into contact with one another, complex microstructures and pores are likely to appear. The decease in hardness of Mg-6RE-6B2O3 is greater than that of Mg-6RE-3B2O3. This is mainly because though the grain size of matrix is smaller, the grain form is dendrites (more decreased, diminished)+ spinal projection(mass)+anomalous ball(mass, diminish- ed). This result suggests that: 1) the content of oxygen will be reduced when adding B2O3 in Mg-RE alloys; 2) in Mg-6RE-xB2O3 matrix particle reinforce composite material, the solidification rate will be increased, which may produce nanometer crystallization of block material or non-crystallization of block.
5 Conclusions
1) Adding 3% or 6%B2O3 in Mg can bring twinning in Mg; adding B2O3 in Mg-Al alloys and Mg-RE alloys can refine the alloy grain size; adding 3%B2O3 in Mg-6Al alloys can refine the average grain size by about 5 μm, with the average hardness increased by 13.3% (HV0.03 53.3-60.4); adding 6%B2O3 in Mg-6%Al alloys can refine the average grain size by about 13 μm, with the average hardness increased by 15.8% (HV0.03 53.3-61.73); adding 3% and 6%B2O3 into Mg-6RE alloys respectively can refine the grain size by about 5 and 9 μm respectively, with the average hardness decreased to HV0.03 64.66 and HV0.03 57.86 respectively from Hv0.03 88.57.
2) In the Mg-6Al alloy the content of aluminum is increased, while in the Mg-6RE alloy the content of oxygen is decreased.
3) It is beneficial to develop Mg-Al-B-O particle reinforce composite alloys, and it is feasible to develop the nanometer crystallization of block material by Mg-B-O-RE.
References
[1] ZHANG Z, COUTURE A, LUO A. Microstructure evolution during solidification of AZ91D magnesium alloy in semisolid[J]. Scripta
Mater, 1998, 38: 45.
[2] L? Yi-zhen, WANG Qu-dong, ZENG Xiao-qin, DING Wen-jiang, ZHAI Chun-quan, ZHU Yan-ping. Effects of rare earths on the microstructure, properties and fracture behavior of Mg-Al alloys[J]. Mater Sci Eng A, 2000, A278: 66-76.
[3] SASAKI G, YOSHIDA M, YANAGISAWA O, FUYAMA N, FUJII T. Mechanical properties and microstructure of Al18B4O33/Mg alloy composites prepared by compo-casting[J]. Materials Science Forum, 2003, 419-422: 777-782.
[4] MOTEGI T, YANO E, TAMURA Y, SATO E. Clarification of grain refining mechanisms of superheat-treated Mg-Al-Zn alloy castings[J]. Materials Science Forum, 2000, 350-351: 191-198.
(Edited by CHEN Wei-ping)
Corresponding author: BU Le-ping. E-mail: 041d9012@gsst.stud.kumamoto-u.ac.jp; buleping123@yahoo.com