J. Cent. South Univ. Technol. (2008) 15(s1): 534-539
DOI: 10.1007/s11771-008-416-x

Thermal relaxation and mechanical relaxation of rice gel
DING Yu-qin(丁玉琴), ZHAO Si-ming(赵思明), XIONG Shan-bai(熊善柏)
(College of Food Science and Technology, Huazhong Agricultural University, Wuhan 430070, China)
Abstract: Rice gel was prepared by simulating the production processes of Chinese local rice noodles, and the properties of thermal relaxation and mechanical relaxation during gelatinization were studied by differential scanning calorimetry (DSC) measurement and dynamic rheometer. The results show that during gelatinization, the molecular chains of rice starch undergo the thermal relaxation and mechanical relaxation. During the first heating and high temperature holding processes, the starch crystallites in the rice slurry melt, and the polymer chains stretch and interact, then viscoelastic gel forms. The cooling and low temperatures holding processes result in reinforced networks and decrease the viscoelasticity of the gel. During the second heating, the remaining starch crystallites further melt, the network is reinforced, and the viscoelasticity increases. The viscoelasticity, the molecular conformation and texture of the gel are adjusted by changing the temperature, and finally construct the gel with the textural characteristics of Chinese local rice noodle.
Key words: rice gel; rheological properties; thermal relaxation; mechanical relaxation
1 Introduction
Chinese rice noodle is one of the typical starchy gelatinous food. Different processing parameters of rice noodles result in different quality properties[1-2]. The production process of rice noodle is known as starch gelatinization. At limited moisture content and certain temperature, the starch granules in rice slurry imbibed water and swelled, the crystallites were destroyed, the birefringence was lost, and the amylose inside the granules leached out into the continuous aqueous phase simultaneously[3-5]. Some outmost chains of amylopectin stretched during the granules swelling. On cooling, leached amylose chains and stretched amylopectin chains made up the continuous gel phase filled with the dispersed phase of swollen starch granules. During the gelatinization process, three-dimensional network structures were formed because of the interaction and entanglement of the starch molecular chains, and the skeletons of the gel were attributed to the swollen starch granules mainly containing amylopectin molecules. Both the continuous phase (three-dimensional network) and swollen gelatinized granules contributed the viscoelasticity of the gel.
The characteristics of starchy gel were strongly influenced by heating temperature[6-8], heating time[6, 8-9], heating rate[6], and so on. The processed characteristics[10-11], mouth sensation[10] and texture properties[10-11] of the starchy gelatinous food depended heavily upon the viscoelasticity and strength of the gels. However, the mechanism is still unclear. During gelatinization the properties of thermal relaxation[12] and mechanical relaxation[13] can demonstrate the mechanism of gelatinization.
In this paper, the production process of a Chinese rice noodles which undergo two heating processes is simulated for gel preparation, and the properties of thermal relaxation and mechanical relaxation during rice gelatinization are studied by differential scanning calorimetry (DSC) measurement and dynamic rheometer. The mechanism of gelatinous food process is discussed, and this study would provide theories to the process of rice gelatinous food.
2 Experimental
2.1 Materials
Rice (yuchimi) was kindly donated by Hunan Jinjian Cereals Co. Ltd. The rice sample was analyzed for moisture 12.31%, protein 10.14%, fat 2.66%, and blue value 0.40[14].
2.2 Methods
2.2.1 Rice slurry preparation
The rice was washed with tap water three times. Rice/water mixtures samples were prepared by mixing rice with tap water at mass ratio of 1?1, and then soaked for 16 h at 40 ℃ to make rice granules absorb water and swell adequately. The rice was rinsed twice with tap water and then milled with water (the mass ratio of rice to water was 1?2) by a refiner (YU8022, Electronic and Machinery Co. Ltd., Heibei, China), and then screened through a 100 mesh sieve. The rice slurry was adjusted to achieve a moisture content of 75%.
2.2.2 DSC measurements
30 μL rice slurry was put into standard DSC aluminum pans which were afterwards hermetically sealed. The samples were scanned against a blank (empty pan) by using a DSC (204F1 Phoenix, NETZSCH instruments Co. Ltd., Tebau, Germany). The temperature was controlled as a Chinese rice noodle process (see Fig.1).
The process was divided into 5 stages. Stage 1 was the first heating process, during which the temperature increased from room temperature (30 ℃) to 90 ℃ at heating rate of 12 ℃/min. Stage 2 was the high temperature holding process, during which the temperature was maintained at 90 ℃ for 20 min. Stage 3 was the cooling process, during which the temperature declined from 90 ℃ to 40 ℃ in 5 min. Stage 4 was the low temperature holding process, during which the temperature was maintained at 40 ℃ for 20 min. Stage 5 was the second heating process, during which the temperature was raised to 90 ℃ in 5 min.
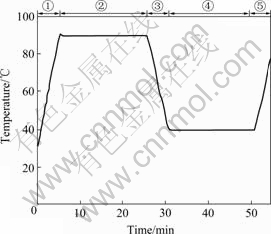
Fig.1 Temperature change in rice slurry gelatinization
In this study, the onset temperature of endothermic peak was determined by the triangular method, and the maximum ordinate of the peak corresponds to the peak temperature[15]. The glass transition temperature (tg) and the range of the glass transition temperature were determined by tangential method[16-17].
2.2.3 Dynamic rheological properties test
Dynamic rheological properties during heating and cooling cycles of rice gelatinization were measured with a dynamic rheometer (AR 500, TA Co., Ltd., Manchester, U.K.). A 40 mm parallel steel plate geometry with a l mm gap was used. 5 μL rice slurry was put onto the parallel plate and the sample was surrounded by a liquid paraffin to prevent drying. For time and temperature sweep tests, the samples were scanned for each stage (see Fig.1) at a constant frequency of 0.795 s-1. The dynamic rheological properties of the final prepared sample were also measured for running a frequency sweep test at a constant temperature 25 ℃, over a frequency range of 0-100 s-1.
3 Results and discussion
3.1 Thermal relaxation of rice slurry gelatinization
Fig.2 illustrates the thermal relaxation of the rice slurry during heating by DSC determination.
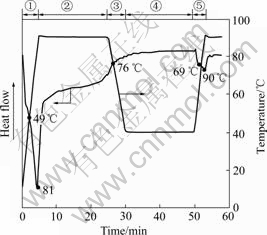
Fig.2 Thermal properties of rice slurry gelatinization: ① First heating process; ② Temperature holding process; ③ Cooling process; ④ Low temperature holding process; ⑤ Second heating process
An endothermic peak is observed in stage 1, whose onset and peak temperatures are 70 ℃ and 81 ℃ respectively (Fig.2). This is attributed to the melting of the starch crystallites and the occurrence of gelatinization of starch[18]. The difference in gelatinization temperature may be influenced by starch purity, amylose to amylopectin ratio, crystalline to amorphous ratio, and molecular structure of amylopectin, and so on[6, 19]. Many studies have reported the different temperatures of rice starch gelatinization[1-2, 6, 8]. HORMDOK and NOOMHORM[1] reported that a main endotherm accompanying gelatinization of purified rice starch, containing a very low quantity of protein (40 mg/100 g) and lipids (20 mg/100 g), exhibiting onset temperature (to) of 68 ℃, and the top temperature (tp) of 73 ℃. The observed to and tp of purified rice starch were lower than those reported in this study. It is important to note that in this study, starch granules contain more protein and lipid than the purified rice starch. The protein and lipid combined with starch in rice slurry cause the rice starch to absorb water slowly and also to gelatinize, resulting in the increase in starch gelatinization temperature.
Thereafter, the endothermic flow does not return to the thermal state of raw rice slurry, indicating that rice slurry has undergone a glass transition. The range of glass transition temperature is 40-58 ℃, and the glass transition temperature (tg) is 49 ℃. The glass transition is known as a second order transition which is a change of state but not phase. The glass transition, as a dynamic process accompanying the changes of stiffness, hardness and viscoelasticity of food material, affects the molecular mobility, physical stability and processability of the food components.
During the high temperature holding process (Stage 2), the temperature stayed at 90 ℃ for 20 min. As the holding time prolongs, the heat flux increases slightly without any peak or step observed, suggesting that the thermal state is not changed obviously in this stage.
During the cooling process (Stage 3), an exothermic step is observed at the temperature ranging from 88 ℃ to 64 ℃ in the thermal flow curve. This suggests that the gel has experienced a process of glass transition, and the glass transition temperature is approximately 76 ℃. This exothermic is resulted from the freezing of molecular chains and the decrease in the heat motion caused by cooling.
Stage 4 is the low temperature holding process. The heat flux flow nearly does not change, suggesting that the thermal state does not change during the stage.
Stage 5 is the second heating process. Similar to the first stage, an endothermic peak and a step are observed. This indicates that the melting of crystallites and the occurrence of the glass transition. The onset and peak temperature of endothermic peak are 73 and 90 ℃, respectively. The endothermic peak is resulted from the further gelatinization of the incomplete gelatinized starch during the first heating process (Stage 1). The cooling process (Stage 3) leads to the freezing of molecular chains, whereas the subsequent heat treatment, which supplies energy to the molecular chains, makes the molecule chains relax, thus, results in glass transition. The range of glass transition temperature was 59-73 ℃, and tg was 69 ℃ which is lower than the peak temperature of crystallites melting (90 ℃), indicating that more energy is needed for the crystallites melting than that for the glass transition.
3.2 Mechanical relaxation of rice slurry gelatinization
Relevant rheological parameters are obtained by dynamic rheological tests including storage modulus (G′), loss modulus (G″) and loss tangent (tand = G″/G′). The storage modulus (G′), reflecting the elasticity of the gel, is caused by the formation of polymer chains (such as starch chain) network in the gel. The loss modulus (G″), reflecting the viscosity of the gel, is caused by the relative displacement of polymer chains. Loss tangent (tand) is a measure of the relative contribution of viscous components to the mechanical properties of the material, indicating the viscoelasticity character of the gel. Fig.3 shows the mechanical relaxation characteristics of rice slurry gelatinization. It can be seen from Fig.3(a) that the first heating stage (30-90 ℃) lasts 180 s. At the earlier period of heating (0-158 s), G′ and G″ are 0.4-0.8 Pa and 1.0-1.7 Pa, respectively, and the increases in G′ and G″ are slow before 60 ℃. However, tand is 2.0-2.6. Both low values of G′ and G″ indicate that the water in rice slurry is free before gelatinization, and the relative displacement of crystalline starch molecule is weak. The values of tand reaches the maximum peak values of 2.6 and 2.5 at 44 ℃ and 51 ℃, respectively. The temperature at which the mechanical relaxation occurs in this stage corresponds to a slightly thermal relaxation at a temperature around 50 ℃ (Fig.2). This mechanical relaxation is resulted from the relaxation of the secondary molecular chains, and therefore the glass transition occurs at tg = 49 ℃. When the glass transition occurred, the mobility of molecular chains increased. Thus, the occurrence of the glass transition at approximately 49 ℃ was before the starch gelatinization at approximately 70 ℃, and caused the increase in tand.
Between 63 ℃ and 65 ℃, G′ and G″ increase greatly, indicating the great increase in elasticity and viscosity of the sample. This may be due to the melting of starch crystallites, the stretching and softening of molecular main chains, the increase in the combining of polymer molecules and water, and the formation of rice gel. Thereafter, G′ reaches the peak value of 41.370 kPa approximately at 80 ℃, and G″ reaches its maximum value of 7.773 kPa at 73 ℃ before G′, indicating that the starch molecular chains stretch, followed by the forming of network structures. It is agreed with the previous reports that upon heating, G′ increases suddenly after a certain temperature is reached and reaches a maximum value. However, HAGENIMANA et al[20] reported that the maximum values of G′ (G′max) and their corresponding temperatures (tG′, max) are different mainly depending on the amount of amylose content. For example, JYM rice starch which has high amylose content (more than 25%) shows the highest tG′, max during heating, whereas NM rice starch which has low amylose content (less than 2%) shows the lowest tG′, max at both the same starch concentrations. However, further heating can lead to a decrease in G′ value[6, 20]. After 80 ℃ and 73 ℃, G′ and G″ value decrease dramatically. The decrease in G′ can be due to the melting of the remaining crystalline region or the disentanglement of the amylopection molecules in the swollen starch granules that resulted in the granules becoming softer[6, 20]. The step of glass transition is not observed obviously in the DSC curve (see Fig.2), possibly because it is covered by the endothermic peak at 80 ℃.
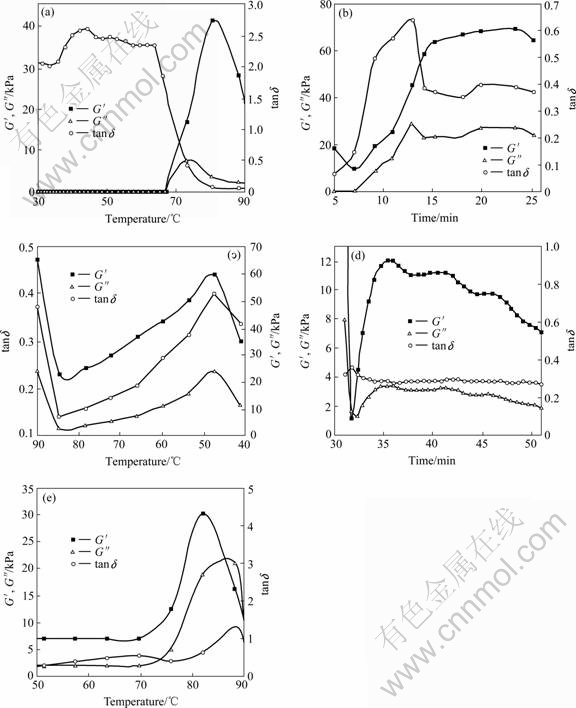
Fig.3 Rheological properties of rice slurry gelatinization (ω=0.795 s-1): (a) First heating (t=0-5 min); (b) High temperature holding at 90 ℃; (c) Cooling (t=25-30 min); (d) Low temperature holding at 40 ℃; (e) Second heating (t=50-55 min)
During 60-80 ℃ tand is down to about 0.1. Starch is the main composition of dry matter of rice. And normally, starch contains 20%-30% amylose and 70%-80% amylopectin. During the gelatinization, the starch granules irreversibly swell, the crystallites disappear, the ordered molecular structures are destroyed, and the amylose inside the granules leaches out, amylose-amylose or amylose-amylopectin outermost branches interact and entangl, resulting in forming the elastic three-dimensional gel networks[5, 21]. And the swelling of starch granules results in an increase in viscosity. The decline of tand indicates that more cross-linked networks are constructed. However, the forming of linked network of molecular chains leads to the decrease in relative displacement resistance, and consequently results in the decrease in G″ and tand as well.
In the high temperature holding process, the temperature was kept at 90 ℃ for 20 min (see Fig.3(b)). G′ increases gradually and reaches a maximum value of 69.970 kPa at about 23 min. At about 13 min, G″ and tand approach the maximum values of 29.500 kPa and 0.7, respectively. Compared to the end of the first stage (see Fig.3(a)), G′, G″ and tand increase. This suggests that the high temperature holding process causes some low molecular mass amylopectin to leach out and stretch, which interacts and entangles with amylose to strengthen the network, and also softens the starch granules, and thus, leading to the increase in elasticity and viscosity of the gel. However, the percentage of elastic component of the gel decreases, and the percentage of viscous component increases, resulting in the increase in tand. In this process, the change of the thermal properties of the sample is not obvious from DSC curve.
In the cooling process, the temperature declines from 90 ℃ to 40 ℃ during 5 min (see Fig.3(c)). At the initial stage of the cooling, starch molecular chains begin to freeze, the amount of hydrated starch decreases, and thermal motion reduces and the glass transition occurs (see Fig.2), giving rise to the falling of G′ and G″ in Fig.3(c). With the temperature declines further, the gel network shrinks, the strength increases, and inner consumption of molecular motion increases, leading to the increase in G′ and G″. When the temperature approaches 48 ℃, G′, G″ and tand reach their maximum values of the cooling process, 59.640 kPa, 23.900 kPa and 0.4, respectively. With the temperature continues to decline, the molecular chains freeze further, and the water-holding capability decreases, resulting in the falling down of G′, G″ and tand. Compared with the last stage (stage 2, Fig.2(b)), G′, G″ and tand values are lower at the end of cooling process, indicating that the elasticity and viscosity of gel fall down, and the proportion of the elastic component increases relatively.
The temperature maintains at 40 ℃ for 20 min in the low temperature holding process (see Fig.3(d)). At the initial period of this stage, G′ and G″ keep on declining dramatically, and then increase, and respectively reach the maximum values of 12.110 kPa and 3.450 kPa at 35 min. However, a nearly constant tand value of 0.3 is observed in this period. And compared with the cooling process (see Fig.3(c)), both G′ and G″ become smaller, suggesting that this stage causes further decrease in elasticity and viscosity of gel. During the process of this stage, two weak peaks of G′ and G″ at 40 min and 46 min are observed, showing two weak mechanical relaxations, which is resulted from the frozen of small motion units of the secondary molecular chains, and the decrease in water-holding capability and the loss of the hydrated linkages of the network structures. However, no thermal relaxation is observed in this stage (see Fig.2).
In the second heating process, the temperature rises from 40 ℃ to 90 ℃ during 5 min (see Fig.3(e)). G′, G″ and tand approach their peak values of 30.240 kPa, 21.200 kPa and 1.3, respectively at 82, 88 and 88 ℃. This is probably due to the further melting of remaining starch crystallites, the stretching of frozen molecular chains, and the occurrence of glass transition which is supported by the thermal relaxation curve (see Fig.2). The occurrence of peak value of G′ is before G″, suggesting that more energy is needed for softening the linear molecular chains than for changing the network. Compared with the low temperature holding process (Stage 4), after the second heating, G′ and G″ increase, indicating the increase in elasticity and viscosity of the gel. Moreover, tand also increases. The reason for the increase in G′ may be that as a result of the increase of temperature, the crystallites which constitute the cross linkage of network melted, and the linear molecular chains stretch and soften. Compared with the value at the end of the first heating, G″ and tand value at the end of the second heating process is higher. However, G′ value is lower, suggesting that after cooling, the low temperature is held and the second heating processes, the elasticity of the gel decreases, and viscosity increases, and the proportion of the elastic structure to viscous structure is adjusted, and the polymer structures are rearranged.
3.3 Frequency sweep of rice gel
In Fig.4, G′ shows negative value in the low frequency area, and the maximum value appears around 38 s-1. And in this low frequency area, d>90? suggests a big hysteresis, indicating the stiffness and viscous of food materials. After 40 s-1, G′ turns to be positive gradually, and shows its peak value 360 Pa at 97 s-1. However, G″ shows its peak values 293 Pa and 458 Pa at 29 s-1 and 97 s-1 before G′. While d shows its peak value 106? and 54? at 17 s-1 and 97 s-1, respectively. This suggests that two mechanical relaxations are involved in the rice gel structure at low and high frequency area, respectively, in the range of frequency studied. Low frequency is close to the frequency of human chewing, and the mechanical relaxation indicates the mouth sensation of the gel, such as toughness, softy, and viscosity. The final G′, G″ and tand of the gel (25 ℃, 50 s-1) are measured as 67 Pa, 208 Pa and 2.1, respectively. G′ and G″ are lower than those of the end of the second heating stage. However, tand is higher, indicating that the strength, elasticity and viscosity of the rice gel in the ambient temperature are weaker than the gel which is just prepared. The elasticity contributes more viscosity.
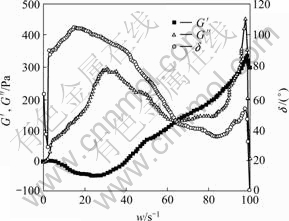
Fig.4 Frequency sweep curves of rice gel (25 ℃)
4 Conclusions
1) The thermal relaxation and the mechanical relaxation reflect the thermal history and the changes of viscoelasticity during the forming processes of the rice gel from raw slurry.
2) At certain moisture and temperature, molecular chains of the rice starch undergo thermal relaxation and mechanical relaxation, which reflects the gelatinization processes including the stretching of the starch molecular chains and the construction of three-dimensional network of the gel.
3) Different heating and cooling processes adjust the strength, viscoelastcity and molecular structure of gel, and the proportion of network to linear molecule, and improve the mechanical properties, and finally, construct the gel which possesses the textural characteristics of Chinese local rice noodle.
4) The characteristics of thermal relaxation and mechanical relaxation can be simultaneously measured by the combined DSC and dynamic rheometer, to better explain the mechanism of rice gelatinization.
References
[1] HORMDOK R, NOOMHORM A. Hydrothermal treatments of rice starch for improvement of rice noodle quality[J]. Journal of Food Science and Technology, 2007, 40(10): 1723-1731.
[2] LU Z H, LI L T, MIN W H, WANG F, TATSUMI E. The effects of natural fermentation on the physical properties of rice flour and the rheological characteristics of rice noodles[J]. International Journal of Food Science and Technology, 2005, 40: 985-992.
[3] FREDRIKSSONA H, SILVERIOB J, ANDEMON R, ELIASSON A C, AMAN P. The influence of amylose and amylopectin characteristics on gelatinization and retrogradation properties of different starches[J]. Carbohydrate Polymers, 1998, 35: 119-132.
[4] KEETELS J A M, VLIET T V, WALSTRA P. Gelation and retrogradation of concentrated starch systems (Ⅰ): Gelation[J]. Food Hydrocolloids, 1996, 10: 343-353.
[5] RING S G. Some studies on starch gelation[J]. Starch, 1985, 37: 80-83.
[6] AHMED J, RAMASWAMY H S, AYAD A, ALLI I. Thermal and dynamic rheology of insoluble starch from basmati rice[J]. Food Hydrocolloids, 2008, 22(3): 278-287.
[7] NAYOUF M, LOISEL C, DOUBILIER J L. Effect of thermomechanical treatment on the rheological properties of crosslinked waxy corn starch[J]. Journal of Food Engineering, 2003, 59: 209-219.
[8] RATNAYAKE W S, JACKSON D S. A new insight into the gelatinization process of native starches[J]. Carbohydrate Polymers, 2007, 67: 511-529.
[9] HSU S, LU S, HUANG C. Viscoelastic changes of rice starch suspensions during gelatinization[J]. Food Chemistry and Toxicology, 2000, 65(2): 215-220.
[10] CHEN Z, SAGIS L, LEGGER A, LINSSEN J P H, SCHOLS H A, VORAGEN A G J. Evaluation of starch noodles made from three typical chinese sweet-potato starches[J]. Food Chemistry and Toxicology, 2002, 67(9): 3342-3347.
[11] SINGH N, SINGH J, SODHI N S. Morphological, thermal, rheological and noodle-making properties of potato and corn starch[J]. Journal of the Science of Food and Agriculture, 2002, 82: 1376-1383.
[12] YOSHIDA H. Relationship between enthalpy relaxation and dynamic mechanical relaxation of engineering plastics[J]. Thermochimica Acta, 1995, 266: 119-127.
[13] LOURDIN D, COLONNA P, BROWNSEY G J, NOEL T R, RING S G. Structural relaxation and physical ageing of starchy materials[J]. Carbohydrate Research, 2002, 337: 827-833.
[14] American Association of Cereal Chemists. Approved methods of the AACC (10th Ed.). St.Paul, MN, 2000.
[15] MATVEEV Y I, SOEST J J G, NIEMAN C, WASSERMAN L A, PROTSEROV V A, EZERNITSKAJA M, YURYEV V P. The relationship between thermodynamic and structural properties of low and high amylose maize starches[J]. Carbohydrate Polymers, 2001, 44(2): 151-160.
[16] BRAKE N C, FENNEMA O R. Glass transition values of muscle tissue[J]. Journal of Food Science, 1999, 64(1): 10-15.
[17] MESTE M L, CHAMPION D, ROUDAUT G, BLOND G, SIMATOS D. Glass transition and food technology: A critical appraisal[J]. Journal of Food Science, 2002, 67(7): 2444-2458.
[18] ROLEE A, MESTE M L. Thermomechanical behavior of concentration starch-water preparation[J]. Cereal Chemistry, 1997, 74(5): 581-588.
[19] GUNATATNE A, HOOVER R. Effect of heat-moisture treatment on the structure and physicochemical properties of tuber and root starches[J]. Carbohydrate Polymers, 2002, 49: 425-437.
[20] HAGENIMANA A, PU P P, DING X L. Study on thermal and rheological properties of native rice starches and their corresponding mixtures[J]. Food Research International, 2005, 38: 257-266.
[21] TESTER R F, MORRISON W R. Swelling and gelatinization of cereal starches (Ⅰ): Effects of amylopectin, amylose and lipids[J]. Cereal Chemistry, 1990, 67: 551-557.
(Edited by LONG Huai-zhong)
Received date: 2008-06-25; Accepted date: 2008-08-05
Corresponding author: XIONG Shan-bai; Tel: +86-27-87288375; E-mail: xiongsb@mail.hzau.edu.cn