
J. Cent. South Univ. (2018) 25: 506-515
DOI: https://doi.org/10.1007/s11771-018-3755-2

Microstructure, mechanical properties, and milling performance of arc-PVD AlTiN–Cu and AlTiN/AlTiN–Cu coatings
CHEN Hao(陈浩)1, CHEN Kang-hua(陈康华)1, XU Yin-chao(徐银超)1, 2,PAN Chen-xi(潘晨曦)1, YI Ji-yong(易继勇)1, ZHU Chang-jun(祝昌军)1
1. State Key Laboratory of Powder Metallurgy, Central South University, Changsha 410083, China;
2. Zhuzhou Cemented Carbide Cutting Tools Co. Ltd., Zhuzhou 412000, China
Central South University Press and Springer-Verlag GmbH Germany, part of Springer Nature 2018
Abstract: AlTiN, AlTiN–Cu and AlTiN/AlTiN–Cu coatings were prepared on WC–6%Co substrates by cathode arc evaporation deposition technology. Two kinds of nitrogen pressures were used to deposit both AlTiN–Cu and AlTiN/AlTiN–Cu coatings. Surface and cross-sectional morphologies of films were observed by scanning electron microscopy (SEM). Crystal structure of films was analyzed by X-ray diffraction (XRD) and X-ray photoelectron spectroscopy (XPS). Hardness and adhesion of films were measured by nano-indentation and nano-scratch tester. Cutting tests were performed under milling conditions during wet machining of TC4 alloy. The results show that with addition of Cu, more droplets occur on AlTiN coating surface, but the grain size of it is refined, and the hardness decreases but the toughness is improved. Under higher N2 pressure, the defects on the surface of AlTiN–Cu and AlTiN/AlTiN–Cu coatings diminish, and the hardness of them is enhanced, while the adhesion is reduced. Compared to AlTiN coated cemented carbide tool, the lifetimes of AlTiN–Cu and AlTiN/AlTiN–Cu coated tools under the same N2 pressure are improved by 11% and 24%, respectively.
Key words: coated cemented carbide; AlTiN–Cu; AlTiN/AlTiN–Cu; N2 pressure; milling
Cite this article as: CHEN Hao, CHEN Kang-hua, XU Yin-chao, PAN Chen-xi, YI Ji-yong, ZHU Chang-jun. Microstructure, mechanical properties, and milling performance of arc-PVD AlTiN–Cu and AlTiN/AlTiN–Cu coatings [J]. Journal of Central South University, 2018, 25(3): 506–515. DOI: https://doi.org/10.1007/s11771-018-3755-2.
1 Introduction
Titanium alloys are widely employed in aerospace, defense and medical industries for their unique combination of properties like lightweight, high-strength at elevated temperature, corrosion resistance and wear resistance. However, titanium alloy is also one of difficult-to-machine materials due to low thermal conductivity, low elastic modulus and high chemical affinity, resulting in high cutting temperature, rapid flank wear and consequent short lifetime of cutting tool [1–4].
AlTiN is one of the most popular hard coatings used for high-speed machining application to improve the performance and lifetime of cutting tool because of its superior hardness and oxidation resistance. But the high internal of AlTiN coating is still a serious drawback to be overcome [5, 6]. Recently, nanostructured films have been attracting much attention for their super-high hardness and superior wear resistance [7]. There are two main kinds of hard nanocomposite films, nc-MeN/hard phase (a-Si3N4, a-TiB2, etc) and nc-MeN/soft phase (Cu, Ni, Y, etc). Studies have shown that copper is very ductile and nonreactive to nitrogen. Besides, it is useful for refining the polycrystalline structure and turning the internal compressive stress of the hard coatings as well [8, 9]. LEU [10] had deposited TiAlN/Cu multilayered coating and found that the coating showed a lower hardness of about 24 GPa (30 GPa for TiAlN) but a higher elastic modulus of about 361 GPa (348 GPa for TiAlN). FOX-RABINOVICH [11] comprehensively studied the structure, properties and wear performance of AlTiN/Cu nano-multilayered coating during cutting of Ni-based Inconel 718 super-alloy and found that the enhanced lubricating property and the reduced thermal condition of the coating ensure multiple channels of energy dissipation during friction, resulting in significantly improved lifetime of cutting tool under unstable condition of attrition wear.
In this work, AlTiN, AlTiN–Cu and AlTiN/AlTiCuN coatings were prepared by cathode arc evaporation deposition technology. Microstructure, mechanical properties, and milling performance of coatings were characterized to study the influence of soft metal Cu and N2 pressure.
2 Experimental
AlTiN, AlTiN–Cu and AlTiN/AlTiCuN coatings were synthesized on WC–6%Co substrates by cathode arc evaporation deposition technology. Ti33Al67 and (Ti33Al67)97Cu3 targets manufactured by a powder metallurgical process were used to prepare AlTiN and AlTiN–Cu coatings, respectively. Both targets were used together to prepare AlTiN/AlTiN–Cu coating. Deposition parameters used for preparation of investigated coatings are listed in Table 1.
Table 1 Deposition parameters used for preparation of investigated coatings
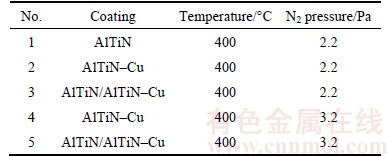
The surface and cross-sectional morphologies of coatings were observed using scanning electron microscope (SEM). The composition of coatings was analyzed by energy dispersive spectrometer (EDS). XRD and XPS were used together to determine the crystal structure of coatings. The hardness and adhesion of coatings were measured by CSM nano-indentation and nano-scratch tester.
Cutting tests were performed under milling conditions during wet machining of TC4 alloy. Cutting data for the experiments performed are presented in Table 2. The maximal flank wear of coated tools was measured using an optical microscope.
3 Results and discussion
3.1 Microstructure and chemical composition of coatings
Surface micrographs of all coatings are shown in Figure 1 and element contents of them are listed in Table 3. Cu contents of AlTiN–Cu and AlTiN/ AlTiN–Cu coatings under different N2 pressure are nearly the same, indicating that Cu contents of coatings are not affected by N2 pressure. It is clear that the surface morphologies of all coatings are plat and uniform with some droplets on them. However, the surface droplets of AlTiN coating become more and larger with addition of Cu because (Ti33Al67)97Cu3 target is easier to evaporate compared to Ti33Al67 target for its higher thermal conductivity (the thermal conductivity of Cu (401 W/(m·K)) is higher than Al (237 W/(m·K)) and Ti (15.24 W/(m·K)) and lower melting point (the melting point of Cu (1080 °C) is lower than Al67Ti33 (1454 °C)) [12]. Due to lower content of Cu, AlTiN/AlTiN–Cu multilayered coating has less droplets on the surface. With rising of N2 pressure, fewer and smaller droplets can be found on the surface of both AlTiN–Cu and AlTiN/AlTiN–Cu coatings. It is associated with the formation of a compound layer with higher fusion point on the surface of cathodes and accelerated movement of cathode spots, resulting in reduction of target emission [13, 14].
Cross-sectional micrographs of coatings are shown in Figure 2. The thicknesses of all coatings are measured as nearly 3 μm. The crystallites of AlTiN film are typical columnar. With adding of Cu, the columnar crystals disappear and the grain sizes decrease. According to previous study, it can be attributed to the fact that the Cu exists in the boundaries of AlTiN crystallites, preventing the growth of columnar grains [15]. No lamellar structure is observed in cross-sectional morphology of AlTiN/AlTiN–Cu coating for its modulation period is too tiny to be observed through SEM or continuous growth of AlTiN crystallites leads to unobvious lamellar structure. With increasing of N2 pressure, the cross-sectional micrographs of AlTiN–Cu and AlTiN/AlTiN–Cu coatings remain almost unchanged.
Table 2 Cutting data for experiments performed

Figure 1 SEM images of surface morphologies of coatings:
Table 3 Element content of coatings
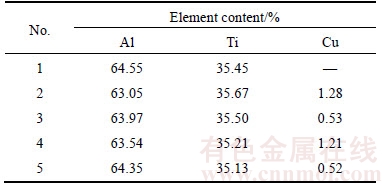
The XRD patterns of all coatings are shown in Figure 3. It is found that all films exhibit a face- centered cubic (fcc) structure. Two main peaks occur in the pattern of AlTiN film, at around 2θ=37.08° and 2θ=43.45°, corresponding to AlTiN (111) and AlTiN (200) which compared to TiN shift to higher 2θ caused by the decreasing in TiN lattice parameter since the Ti is replaced by Al with smaller atomic size. With addition of Cu, the diffraction peak of (111) almost disappear and the peak of (200) is most prominent, revealing that the preferred orientation of coating changes from (111) to (200). Besides, the diffraction peaks become wider with adding of Cu, indicating that the grain sizes of coatings are refined. With increase of N2 pressure, a peak of AlTi3N (200) appears most likely for the target-poisoning, which may change the composition of local target surface [16]. No peak of Cu can be found in XRD patterns of all samples and it can be deduced that Cu exists either as nanocrystallines in a too low concentration to be detected by XRD, or as an amorphous phase, or alternatively in a dispersed form [17].
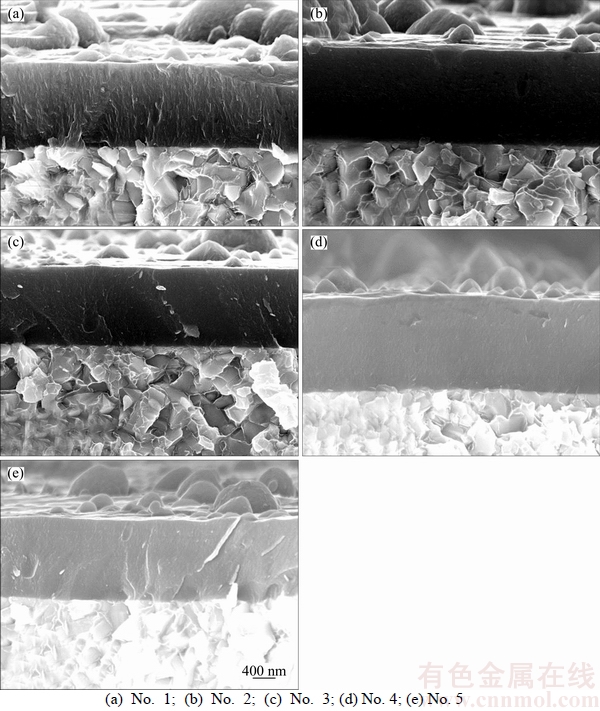
Figure 2 SEM images of cross- sectional morphologies of coatings:
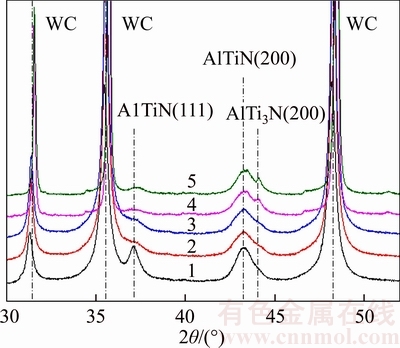
Figure 3 XRD patterns of coatings 1–5
Figure 4 illustrates the XPS spectra taken in the Al 2p, Ti 2p, N 1s and Cu 2p energy regions for AlTiN–Cu film deposited at 2.2 Pa. After the peak fitting by XPSSPEAK41, it is found that the Al 2p peak is composed of two components at the binding energy of 74.2 eV and 73.3 eV, corresponding to Al–N bonds and Al–Ti bonds in complex nitride, respectively. For the Ti 2p spectra, the peak at a binding energy of 462.2 eV corresponds to Ti–N bonds and the peak at a binding energy of 456.9 eV corresponds to Ti–Al bonds in complex nitride. The N1s peak could be resolved into two components, one of which at the binding energy of 397.1 eV is consistent with TiN and the other at a binding energy of 396.2 eV is accordant with AlN, demonstrating the formation of solid solution (Al, Ti)N. The peaks found in the Cu 2p region at the binding energy of 932.8 eV and 952.9 eV correspond to Cu 2p3/2 and Cu 2p1/2 of copper in pure metal, separately, declaring that the ability of Cu to form nitrides is weak and consequently Cu is just incorporated in the coating as metal. It turned out that combined with XRD and XPS analysis the Cu exists as metallic phase in AlTiN–Cu and AlTiN/AlTiN–Cu coatings.
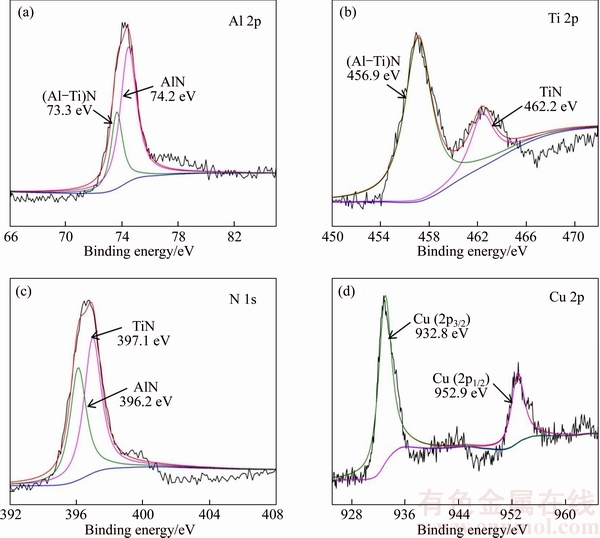
Figure 4 XPS core-level spectra taken in Al 2p (a), Ti 2p (b), N 1s (c) and Cu 2p (d) of AlTiN–Cu film deposited at 2.2 Pa
3.2 Mechanical properties
Loading–unloading curves of all coatings are shown in Figure 5 and nano-indentation data of them are listed in Table 4. All the load– displacement curves exhibit similar trends. AlTiN proves the highest hardness and modulus among all coatings, while with addition of Cu, the hardness and modulus of it decrease together. The decline of hardness can be accounted for three reasons. Firstly, the covalent energy gap (Eh=Kd–2.5) is reduced because of decreased interatomic distance (d) caused by less content of Al. Secondly, Cu exits as soft metallic phase with low hardness. Finally, more droplets emerge on the coating surface, providing more sources of cracks initiation [18]. Compared to AlTiN–Cu coating, AlTiN/AlTiN–Cu multilayered coating expresses higher hardness due to lower content of Cu and fewer defects. Moreover, the hardnesses of both AlTiN–Cu and AlTiN/AlTiN–Cu coatings are improved with increscent N2 pressure. There are two main reasons. Firstly, under higher N2 pressure, more hard nitride phase will be generated in the coating. Secondly, the coating under higher N2 pressure shows less defects and better surface morphology. The ratio of HIT/E is a good indicator of coating toughness and AlTiN–Cu and AlTiN/AlTiN–Cu coatings deposited at 2.2 Pa with lower HIT/E exhibit better wear resistance.
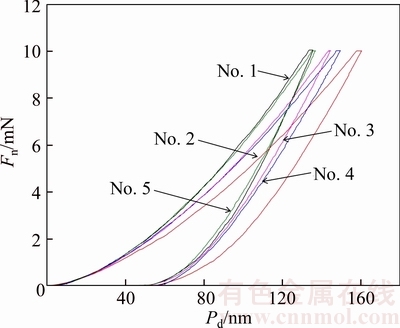
Figure 5 Loading-unloading curves of coatings 1-5 (Fn, loading force measured; Pd, pressure depth)
Table 4 Nano-indentation data of coatings
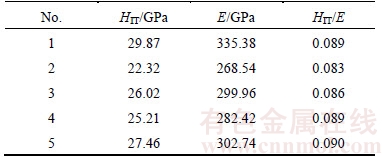
Typical nano-scratch profile of AlTiN coating is shown in Figure 6. Lc1 and Lc2 correspond to the appearance of first crack and complete peeling of the film, respectively. Nano-scratch data of all coatings are listed in Table 5. Generally, Lc1 is used to represent the adhesion strength of coating [19]. Compared to AlTiN coating, the Lc1 of AlTiN–Cu coating is higher, indicating that adhesion strength of coating is enhanced with addition of Cu. However, AlTiN/AlTiN–Cu multilayered coating shows worse adhesion possibly due to material mismatch between AlTiN and AlTiN–Cu. With rise of N2 pressure, the Lc1 of coatings goes down rapidly because under higher N2 pressure, the random collision is increased and the kinetic energy of the incident ions is decreased, thus, the energy that ions need to migrate on growing film surface is reduced, consequently more defects related to vacancies form [18].
3.3 Milling tests
The wear curves of coated cemented carbide cutting tools are shown in Figure 7, which exhibit the basic characteristic of curves obtained during normal metal cutting. According to the variation in tool wear, the coating tool wear process can be divided into three stages, the initial wear stage, the stable wear stage and the sharp wear stage. In the first stage and the third stage, tool wear increases rapidly with cutting length, while in the second stage, the wear band width increases gradually [20]. The rake and flank face morphologies of failed coated tools are shown in Figures 8 and 9, respectively.
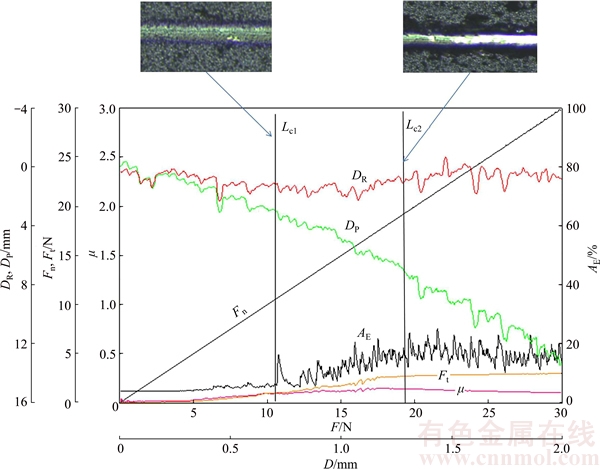
Figure 6 Typical nano-scratch profile of AlTiN coating (Fn, loading force measured; Ft, shear force; μ, friction coefficient; AE, acoustic emission signal; DP, penetration depth; DR, residual depth; D, scratch length; F, loading force)
Table 5 Nano-scratch data of all coatings
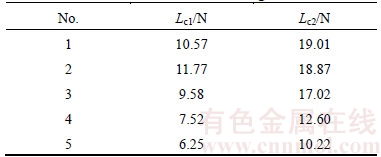
In this study, the lifetime of coated tool is evaluated by the cutting length when the flank wear of tool is approaching 0.2 mm. It is found that compared to AlTiN coated tool, the lifetimes of AlTiN–Cu, AlTiN/AlTiN–Cu coated tools deposited at the same N2 pressure are improved by 11% and 24%, respectively. As shown in Figure 8, an obvious wear groove can be found in the rake face of AlTiN coated tool (Figure 8(a)), but the rake face of AlTiN–Cu coated tool (Figure 8(b)) is relatively plat and the rake face of AlTiN/AlTiN–Cu coated tool (Figure 8(c)) is nearly intact. From the flank face morphologies (Figure 9), the AlTiN coated tool (Figure 9(a)) shows most serious wear; while the AlTiN–Cu and AlTiN/AlTiN–Cu coated tools (Figures 9(b) and (c)) display improvement of attrition. The major feature of milling is that within the cutting zone, the interaction with environment is limited, resulting in diminished ability to form protective tribo-films (alumina-like). Nevertheless, lack of oxygen within the cutting zone may not prevent formation of lubricious Cu-content tribo-films [11]. Therefore, the Cu existing in the boundary of AlTiN grain can form lubricious tribo-films during milling TC4, which alleviate abrasion of AlTiN–Cu and AlTiN/AlTiN–Cu coated tools, resulting in improving the cutting time of them.
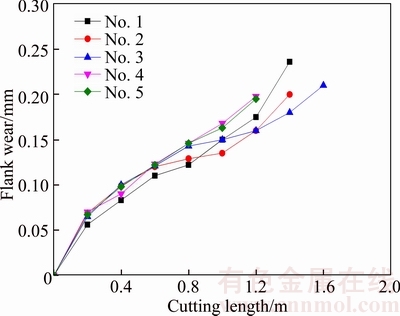
Figure 7 Flank wear as a function of cutting length for tools with coatings 1–5
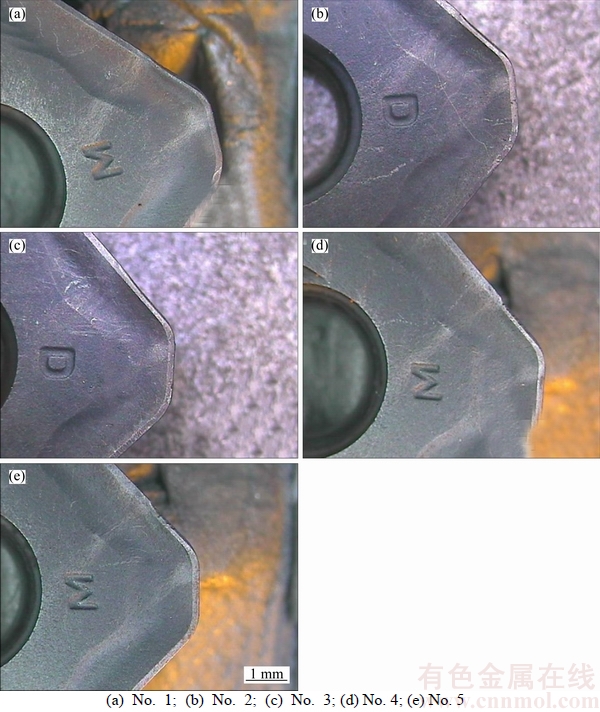
Figure 8 Rake face morphologies of failed tools with different coatings:
Figure 9 Flank face morphologies of failed tools with different coatings:
However, the lifetimes of AlTiN–Cu and AlTiN/AlTiN–Cu coated tools under higher N2 pressure show slight decline. And as shown in Figures 8(c), (d) and Figures 9(c), (d), both the rake wear and flank wear of them are worse. It is mainly caused by their poor adhesion, which leads to the fact that they are easier to peel off under the action of large cutting force during milling TC4.
4 Conclusions
1) With addition of Cu, more droplets arise on the surface of AlTiN coating while the grain size of it is refined. The preferred orientation of AlTiN coating changes from (111) to (200) with adding of Cu and Cu is incorporated in the coating as metal.
2) Under the same N2 pressure, the hardness of both AlTiN–Cu and AlTiN/AlTiN–Cu coatings is lower than that of AlTiN coating, but the adhesion strength of AlTiN–Cu coating is intensive while the adhesion of AlTiN/AlTiN–Cu coating is subdued. Besides, AlTiN–Cu and AlTiN/AlTiN–Cu coatings exhibit better toughness.
3) With increase of N2 pressure, the defects of both AlTiN–Cu and AlTiN/AlTiN–Cu coatings are ameliorative and the hardness of them is enhanced but the adhesion of them is reduced.
4) Compared to AlTiN coated tool, the lifetimes of AlTiN, AlTiN/AlTiN–Cu coated tools are improved by 11% and 24%, respectively, while with rise of N2 pressure, the lifetimes of them go down.
References
[1] EZUGWU E O. Key improvements in the machining of difficult-to-cut aerospace superalloys [J]. International Journal of Machine Tools & Manufacture, 2005, 45(12, 13): 1353–1367. DOI: 10.1016/j.ijmachtools.2005.02.003.
[2] BIKSA A, YAMAMOTO K, DOSBAEVA G, VELDHUIS S C, FOX-RABINOVICH G S, ELFIZY A, SHUSTER L S. Wear behavior of adaptive nano-multilayered AlTiN/MexN PVD coatings during machining of aerospace alloys [J]. Tribology International, 2010, 43(8): 1491–1499. DOI: 10.1016/j.triboint.2010.02.008.
[3] ZHU Xiao-shuo, FU Yu-dong, LI Zi-feng, LENG Ke. Wear resistance of TC4 by deformation accelerated plasma nitriding at 400 °C [J]. Journal of Central South University, 2016, 23(11): 2771–2776. DOI: 10.1007/s11771-016-3339-y.
[4] GHANI J A, CHE H C H, HAMDAN S H, SAIDA Y M, TOMADI S H. Failure mode analysis of carbide cutting tools used for machining titanium alloy [J]. Ceramics International, 2013, 39(4): 4449–4456. DOI: 10.1016/j.ceramint.2012. 11.038.
[5] MAYRHOFER P H, MITTERER C, HULTMAN L, CLEMENS H. Microstructural design of hard coatings [J]. Progress in Materials Science, 2006, 51(8): 1032–1114. DOI: 10.1016/j.pmatsci.2006.02.002.
[6] Fox-Rabinovich G S, Kovalev A I, AGUIRRE M H, BEAKE B D, YAMAMOTO K, VELDHUIS S C, ENDRINO J L, WAINSTEIN D L, RASHKOVSKIYB A Y. Design and performance of AlTiN and TiAlCrN PVD coatings for machining of hard to cut materials [J]. Surface & Coatings Technology, 2009, 204(4): 489–496. DOI: 10.1016/j.surfcoat.2009.08.021.
[7] WANG X Q, ZHAO Y H, YU B H, XIAO J Q, LI F Q. Deposition, structure and hardness of Ti–Cu–N hard films prepared by pulse biased arc ion plating [J]. Vacuum, 2011, 86(4): 415–421. DOI: 10.1016/j.vacuum.2011.08.015.
[8] DOSBAEVA G K, VELDHUIS S C, ELFIZY A, FOX-RABINOVICHG, WAGG T. Microscopic observations on the origin of defects during machining of direct aged (DA) Inconel 718 superalloy [J]. Journal of Materials Engineering & Performance, 2010, 19(8):1193–1198. DOI: 10.1007/ s11665-009-9587-3.
[9] GULERYUZ C G, KRZANOWSKI J E, VELDHUIS S C, FOX-RABINOVICH G S. Machining performance of TiN coatings incorporating indium as a solid lubricant [J]. Surface & Coatings Technology, 2009, 203(22): 3370–3376. DOI: 10.1016/j.surfcoat.2009.04.024.
[10] LEU M S, LO S C, WU J B, LI A K. Microstructure and physical properties of arc ion plated TiAlN/Cu thin film [J]. Surface & Coatings Technology, 2006, 201: 3982–3986. DOI: 10.1016/j.surfcoat.2006.08.001.
[11] FOX-RABINOVICH G S, YAMAMOTO K, AGUIRRE M H, CAHILL D G, VELDHUIS S C, BIKSA A, DOSBAEVA G, SHUSTER L S. Multi-functional nano-multilayered AlTiN/Cu PVD coating for machining of Inconel 718 superalloy [J]. Surface & Coatings Technology, 2010, 204(15): 2465–2471. DOI: 10.1016/j.surfcoat.2010.01.024.
[12] MIYANO R, KIMURA K, IZUMI K, TAKIKAWAH, SAKAKIBARA T. Preparation of metal nitride and oxide thin films using shielded reactive vacuum arc deposition [J]. Vacuum, 2000, 59: 159–167. DOI: 10.1016/S0042- 207X(00)00266-9.
[13] HARRIS S G, DOYLE E D, WONG Y C, MUNROE P R, CAIRNEY J M, LONG J M. Reducing the macroparticle content of cathodic arc evaporated TiN coatings [J]. Surface & Coatings Technology, 2004, 183(2): 283–294. DOI: 10.1016/j.surfcoat.2003.08.086.
[14] MIYANO R, FUJIMURA Y, TAKIKAWA H, SAKAKIBARA T. Cathode spot motion in vacuum arc of zinc cathode under oxygen gas flow [J]. IEEE Transactions on Plasma Science, 2001, 29(5): 713–717. DOI: 10.1109/ 27.964460.
[15] ZHANG L, MA G J, LIN G Q, HAN K C, MA H. Synthesis of Cu doped TiN composite films deposited by pulsed bias arc ion plating [J]. Nuclear Instruments & Methods in Physics Research, 2014, 320: 17–21. DOI: 10.1016/ j.nimb.2013.12.001
[16] GACHON J C, ROGACHEV A S, GRIGORYAN H E, ILLARIONOVA E V, KUNTZ J J, KOVALEV D Y, NOSYREV A N, SACHKOVA N V, TSYGANKOV P A. On the mechanism of heterogeneous reaction and phase formation in Ti/Al multilayer nanofilms [J]. Acta Materialia, 2005, 53(4): 1225–1231. DOI: 10.1016/j.actamat.2004.11. 016.
[17] POLYCHRONOPOULOU K, DEMAS N G, GIBSON P N, REBHOLZC,POLYCARPOU A A. Effect of Cu content on the structure, and performance of substoichiometric Cr–N coatings [J]. Tribology Letters, 2010, 38(1): 57–68. DOI: 10.1007/s11249-009-9572-x.
[18] CAI Fei, ZHANG Shi-hong, LI Jin-long, CHEN Zhong, LI Ming-xi, WANG Lei. Effect of nitrogen partial pressure on Al–Ti–N films deposited by arc ion plating [J]. Applied Surface Science, 2011, 258(5): 1819–1825. DOI: 10.1016/j.apsusc.2011.10.053.
[19] FENG Chang-jie, HU Xiang-hua, JIANG Yuan-fei, ZHAO Qing. Effects of Cu addition on microstructure and adhesion properties of Ti-Al-N nanocomposite films deposited by magnetron sputtering [J]. Advanced Materials Research, 2013, 652–654: 1751–1754. DOI: 10.4028/www.scientific. net/AMR.652-654.1751.
[20] WANG C Y, XIE Y X, QIN Z, LIN H S, YUAN Y H, WANG Q M. Wear and breakage of TiAlN- and TiSiN-coated carbide tools during high-speed milling of hardened steel [J]. Wear, 2015, 336–337: 29–42. DOI: 10.1016/j.wear.2015. 04.018.
(Edited by YANG Hua)
中文导读
AlTiN、AlTiN–Cu和AlTiN/AlTiN–Cu涂层的微观结构、力学性能及铣削性能
摘要:采用阴极弧蒸发沉积技术在WC–6%Co基体上制备AlTiN、AlTiN–Cu和AlTiN/AlTiN–Cu涂层,采用2种氮气压力制备 AlTiN–Cu和AlTiN/AlTiN–Cu涂层:2.2 Pa和3.2 Pa。利用扫描电镜观测涂层的表面形貌和截面形貌,结合X射线衍射谱和X射线光电子能谱确定涂层成分和晶体结构,利用纳米压痕划痕仪测定涂层的硬度和膜基结合力。在相同条件下,进行湿式铣削TC4试验,对比研究5种涂层刀具的铣削性能。研究结果表明,添加Cu后,AlTiN涂层表面液滴增多,晶粒细化,硬度降低但耐磨性提高。当N2压力由2.2 Pa增大到3.2 Pa时,AlTiN–Cu和AlTiN/AlTiN–Cu涂层晶粒尺寸细化,硬度提高但是膜基结合力下降。在相同N2压力下,AlTiN–Cu和AlTiN/AlTiN–Cu涂层刀具铣削寿命分别较AlTiN涂层刀具的提高11% 和 24%。
关键词:涂层硬质合金;AlTiN–Cu;AlTiN/AlTiN–Cu;N2压力;铣削
Foundation item: Project(2014ZX04012011) supported by Major National Science and Technology Projects, China; Project(51327902) supported by the National Natural Science Foundation of China
Received date: 2016-06-16; Accepted date: 2017-12-20
Corresponding author: CHEN Kang-hua, PhD, Researcher; Tel: +86–731–88830714; E-mail: khchen@mail.csu.edu.cn