J. Cent. South Univ. Technol. (2008) 15: 334-338
DOI: 10.1007/s11771-008-0063-2

Preparation and characterization of nanometer-sized barium titanate powder by complex-precursor method
SHI Ai-hua(石爱华)1, YAN Wen-bin(颜文斌)1, LI You-ji(李佑稷)1, HUANG Ke-long(黄可龙)2
(1. School of Chemistry and Chemical Engineering, Jishou University, Jishou 416000, China;
2. School of Chemistry and Chemical Engineering, Central South University, Changsha 410083, China)
Abstract: A new complex-precursor method was proposed to prepare nanometer-sized BaTiO3 powder. Firstly, [Ti2O(O2)2(ta)2]4- complex ions were prepared by the reaction of H2O2, Ti4+ and ta3- (ta=[C6H6O6N]3-) with a desirable amount of surface active agent, and then the Ba2[Ti2O(O2)2(ta)2]·2H2O precursor was obtained by reaction between [Ti2O(O2)2(ta)2]4- and Ba2+. Finally, the precursor was annealed at 800 ℃ for 2 h to obtain BaTiO3 powder. The morphology, the particle size distribution, the purity and the molar ratio of Ba to Ti of BaTiO3 powder were investigated systematically by TEM, XRD, IR, Raman and chemical analysis, respectively. The results show that the BaTiO3 powders with the grain size of about 40 nm have a tetragonal crystalline structure at room temperature and a spherical morphology.
Key words: barium titanate; complex-precursor method; synthesis; nanometer-sized powder
1 Introduction
Barium titanate is considered an interesting material for the electronic ceramics because of its excellent electrical properties, such as high permittivity, low dielectric loss, ferroelectricity, piezoelectricity and electrical insulation. BaTiO3-based materials are widely used in electronic industry for the application of thermistors, multilayer ceramic capacitors(MLCCS), memory materials, and so on. Recently, with the development and the demand of portable electronic devices, ultrafine BaTiO3 powders less than 100 nm have been required to make progressive MLCCS with high densification and high capacity[1-6]. BaTiO3 is conventionally obtained by solid-state reaction(SSR) between barium carbonate (BaCO3) and titania (TiO2) at 1 200 ℃. However, BaTiO3 powders prepared by SSR method always consist of non-uniform and sub-micrometer sized coarse particles, and even impurities[7-11]. The distribution of particle size is not easy to be controlled, and the grinding process to reduce the size of the particles always results in chemical contamination. The need for nanometer-sized and pure powders has led to the development of many alternative chemical routes, such as thermal decomposition of co-precipitation, molten salt method, sol-gel technique, hydrothermal synthesis, and low-temperature aqueous synthesized(LTAS) method[6-13]. However, to our knowledge, the complex-precursor method for the preparation of BaTiO3 nanoparticles has not been reported.
In this study, a new complex-precursor method based on oxalic acid precipitation was proposed. This technique owns the following advantages: the whole process, with a simple experimental set-up and electrolyte system, can be performed under mild conditions (ambient temperature and atmosphere pressure). On the other hand, the synthesized BaTiO3 exhibits uniform and fine particle sizes with a narrow size distribution.
2 Experimental
2.1 Preparation of Ba2[Ti2O(O2)2(ta)2]·2H2O precursor
BaCl2?2H2O was used as a source of Ba and TiCl4 was used as a source of Ti in the present work. 200 mL solution containing 20 g nitrilotriacetic acid (N(CH2COOH)3, noted by H3ta) and a little surfactant was mixed with 80 mL TiCl4 solution under stirring. Then 10 mL H2O2 was added into the mixture solution. The solution color changed from achromatism to deep red. The mixed solution was stirred for 10 min to produce [Ti2O(O2)2(ta)2]4- complex ions. Then 24 g BaCl2?2H2O was added into the above mixture solution. After BaCl2?2H2O was dissolved in the solution, NH3?H2O was dropped into the solution to adjust pH value to about 2.5 and stirred for further 30 min. The obtained mixture solution was stewed, filtrated and washed with deionized water to eliminate Cl- ions. Then the mixture was washed with a desirable amount of absolute ethanol, and subsequently dried in a temperature range of 100-120 ℃. As a result, Ba2[Ti2O(O2)2- (ta)2]·2H2O precursor was obtained. The element content (mass fraction, %) of the precursor was analyzed and calculated to be C (17.4), H (1.5), N (3.4) and C (17.5), H (1.4), N (3.5), respectively.
The obtained precursor was annealed at 800 ℃ for 2 h to obtain nanometer-sized BaTiO3 powder. The main reaction processes can be suggested as follows:
2TiCl4+2H3ta+2H2O2+3H2O→
H4[Ti2O(O2)2(ta)2]·2H2O+8HCl (1)
H4[Ti2O(O2)2(ta)2]·2H2O+2BaCl2→
Ba2[Ti2O(O2)2(ta)2]+4HCl+2H2O (2)
Ba2[Ti2O(O2)2(ta)2]+O2→BaTiO3+CO+CO2+NO2+H2O
(3)
2.2 Characterization of precursor and BaTiO3 powder
The complex precursors were analyzed by FT-IR spectrum (Impact 400, US) via KBr pressed disc method. DTA/TG (WCT-2C, Netzsch STA) analysis was performed by using alumina as the reference material. The crystalline structure was identified by X-ray diffraction analysis (Y-2000, Bruker, Germany) with Cu Kα as radiation at tube voltage of 30 kV, pipe flow of 20 mA and scanning speed of 0.2 (?)/s. The morphology and particle size of the prepared BaTiO3 samples were observed by a transmission electron microscope(TEM) (JEM-1000X, Japan).
2.3 Purity analysis of BaTiO3 powder
The purity of the prepared BaTiO3 powder was investigated by analyzing the molar ratio of Ba to Ti in the powder with chemical analysis method. A small quantity of BaTiO3 powder was dissolved into concentrated hydrochloric acid, and then H2O2 complex compound titanium (Ⅳ) and (NH4)2SO4 (1 mol/L) were added. The mixture was filtrated, washed and annealed to obtain BaSO4. The content of BaO was calculated by the obtained BaSO4. The concentrated H2SO4 (30 mL), the concentrated HCl (40 mL) and (NH4)2SO4 (10 g) were added into filtrate, then the mixture was boiled to remove H2O2. Subsequently, Ti(Ⅳ) was deoxidized to Ti(Ⅲ) by aluminum piece with titration by using iron ammonium sulfate as standard solution. The content of Ti was calculated and converted into content of TiO2. The content of BaTiO3 is equal to the sum of TiO2 and BaO content. So the molar ratio of Ba to Ti can be calculated.
3 Results and discussion
3.1 Analysis of annealing process
The element analysis of precursor shows that the experimental value of the synthesized compound is consistent with the theoretical value, indicating that the intermediate product is Ba2[Ti2O(O2)2(ta)2]·2H2O. The infrared spectrum of the complex compound precursor is shown in Fig.1. The antisymmetric stretching vibration of complex compound carboxyl is present at 1 588 cm-1, corresponding to its symmetric stretching vibration at 1 418 cm-1. Compared with the shaking frequency of free nitrilotriacetic acid, it is found that the shaking frequency of carboxyl shifts to lower frequency, implying that the coordination exists between nitrilotriacetic acid and titanium atom by carboxyl. The O—O bond in superoxide radicle of complex compound shows stretching vibration at 875 cm-1. Meanwhile, symmetric and antisymmetric stretching vibrations of Ti(O2) are at 602 and 520 cm-1, respectively. The above spectra are in agreement with the reported IR spectrum of titanium superoxide[14-16].
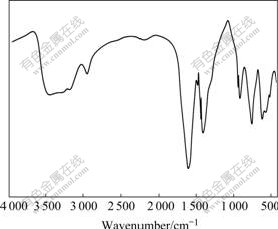
Fig.1 FT-IR spectrum of Ba2[Ti2O(O2)2(ta)2]·2H2O precursor
The synthetic reaction of complex compound precursor and the related thermal decomposition processes are complicated. The analytical results of thermogravimetry and differential thermal analysis of complex compound precursor are shown in Fig.2. It is found that the synthetic reaction of peroxo tris(hydro- xycarbonylmethyl)amine precursor includes three main processes, namely, water loss, decomposition of organic complex compound and formation of BaTiO3. The precursor shows one endothermic peak at 97 ℃ due to water loss, and one endothermic peak at 675 ℃ properly for the phase change of formed complex oxides[17-19]. The actual productive rate (53.8%) is basically consistent with the theoretical productive rate (54.3%), which indicates that the precursor was completely decomposed. The experimental results show that precursor is basically decomposed by annealing at 700 ℃. By increasing the temperature, the grain size of BaTiO3 is decreased and then increased, and grain size reaches the minimum when the temperature is 800 ℃. Setting the calcination temperature as 800 ℃, the grain size of BaTiO3 is gradually decreased and then increased with prolonging the heating time, and reaches the minimum and the maximum at 2 and 3 h, respectively. In order to prepare BaTiO3 powder with the smallest size and decompose the precursor entirely, the heating process for precursor is chosen as 800 ℃ for 2 h in this work.
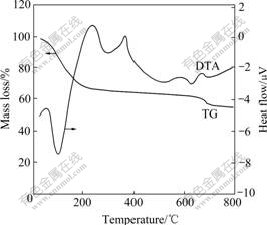
Fig.2 TG-DTA diagrams of Ba2[Ti2O(O2)2(C6H6O6N)2]·2H2O precursor
3.2 Crystal structure and morphology of BaTiO3 powder
Fig.3 shows the typical XRD pattern of BaTiO3 powders annealed at 800 ℃ for 2 h. In Fig.3, only peaks assigned to BaTiO3 are observed, revealing that the product is pure BaTiO3. Splitting peak for (2 0 0)/(0 0 2) (2θ≈45?), (210)/(201) (2θ≈51?), (112)/(211) (2θ≈56?), (202)/(220) (2θ≈66?), (202)/(220) (2θ≈66?) and (103)/ (301)/(310) (2θ≈75?) imply that the primary phase is
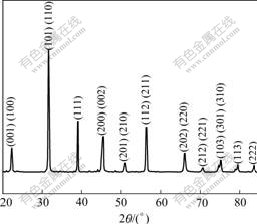
Fig.3 X-ray diffraction pattern of nano-BaTiO3 powders
tetragonal perovskite structure. The mean particle size of the BaTiO3 powders is evaluated from the broadening line of X-ray diffraction peaks by the Scherrer equation:
(4)
where D is the primary particle size, λ is the wavelength of Cu Kα radiation (0.154 nm), θ is the diffraction angle and β is the full wave at half maximum(FWHM) of the peak. The mean particle sizes of the calcined BaTiO3 at 800 ℃ are calculated to be about 36 nm.
According to the crystallography, Raman active modes for tetragonal BaTiO3 (P4mm) are 4E (TO+LO)+ 3A1(TO+LO)+1B1(TO+LO). Fig.4 shows the Raman spectrum of BaTiO3 calcined at 800 ℃. These absorption peaks at 276, 304, 523 and 718 cm-1 are observed. Thus, the Raman spectra confirm that the structure of BaTiO3 particles is tetragonal phase, which is in good agreement with the result of XRD pattern.
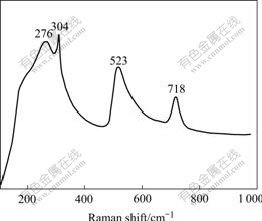
Fig.4 Raman spectrum of BaTiO3 powder
One of the TEM images of the prepared BaTiO3 powders is shown in Fig.5. The TEM image shows that the average size of BaTiO3 is about 40 nm with spherical shape and uniformity. This is in accordance with the calculated size (36 nm) from XRD pattern. Compared with the previous researches, the experimental results indicate that the surfactant has evident effect on particle size and morphology in reaction system. As shown in Fig.6, the possible reason is that the surfactant changes the surface charge distribution of the deposition ions in solution, and then a mini-reaction environment is formed. The special environment has the capacity to separate the ions from each other. So the Ba2[Ti2O(O2)2(C6H6O6N)2]·2H2O precursor is not reunited to a certain degree. The surface performances of BaTiO3 are evidently improved and agglomeration is obviously induced.
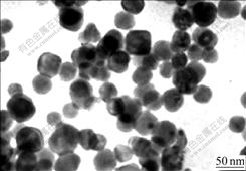
Fig.5 TEM image of nano-BaTiO3 powder
3.3 Physical properties of BaTiO3 particles
The molar ratio of Ba to Ti of the prepared BaTiO3 powder is 1.000±0.005 with a purity of 99.9%, which reaches the primary standard of high purity barium titanate for electronic industry (HG/T3587-1999).
The BaTiO3 particles obtained under the optimum synthesis condition were cold isostatically pressed, sintered and measured for the physical properties. The density of the BaTiO3 ceramics sintered at 1 200 ℃ for 2 h is 5.86 g/cm3, which means 97.6% of the theoretical
density. The dielectric constant measured in the ceramics is 4 800 at room temperature and is much higher than that of hydrothermally prepared BaTiO3 powders[20]. The significantly high value of dielectric constant may be mainly due to uniform nano-sized particles distribution with low aggregation.
4 Conclusions
1) BaCl2?2H2O, TiCl4, nitrilotriacetic acid and surfactant are used as raw materials to prepare BaTiO3 nano-powders by a complex-precursor method. High- purity nanometer-sized BaTiO3 powders can be synthesized by the complex-precursor method.
2) The complex-precursor method can be used as an alternative technique for preparation of nanometer-sized BaTiO3 powders. The potential advantages of this technique are simple setup and mild conditions.
3) The average size of prepared BaTiO3 powders is about 40 nm. The density of the BaTiO3 ceramics prepared by the synthesized BaTiO3 powders is 5.89 g/cm3, and the dielectric constant measured in the ceramics is about 4 800 at room temperature.
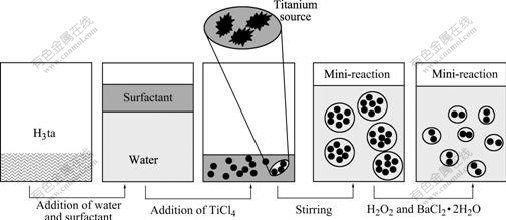
Fig.6 Synthesis mechanism of Ba2[Ti2O(O2)2(ta)2] ·2H2O precursor
References
[1] MI De-wei, ZHOU Yu-xin, WU Yuan, ZHANG Peng-he. Advance progress for preparation of nm-sized barium titanate [J]. Chemical Engineer, 2005, 120(9): 26-28. (in Chinese)
[2] ZHOU Xiao-yuan, GU Hao-shuang, LI Wei-yong. Effect of doped Mn on piezoelectric properties of (Na0.5Bi0.5)0.92Ba0.08TiO3 lead-free ceramics [J]. Journal of Central South University of Technology, 2005, 12(3): 266-268.
[3] WANG J Q, KUWABARA M. Electrophoretic deposition of BaTiO3 films on a Si substrate coated with conducting polyaniline layers [J]. Journal of the European Ceramic Society, 2008, 28(1): 101-108.
[4] SU Tao-long, ZHUANG Zhi-qiang. Adsorption property of citrate dispersant on BaTiO3 particles in aqueous solution [J]. Journal of Central South University of Technology, 2007, 14(2): 202-205.
[5] LEE W H, SU C Y. Improvement in the temperature stability of a BaTiO3-based multilayer ceramic capacitor by constrained sintering [J]. Journal of the American Ceramic Society, 2007, 90(10): 3345-3348.
[6] WANG Li-qiu, LIU Liang, XUE Dong-feng, KANG Hong-min, LIU Chang-hou. Wet routes of high purity BaTiO3 nanopowders [J]. Journal of Alloys and Compounds, 2007, 440(1/2): 78-83.
[7] QUAN Xue-jun, PU Chang-liang. Progress on preparation of barium titanate powders [J]. Materials Review, 2002, 16(6): 45-47,67. (in Chinese)
[8] WADA S, TSURUMI T, CHIKAMORI H, NOMA T, SUZUKI T. Preparation of nm-sized BaTiO3 crystallites by a LTDS method using a highly concentrated aqueous solution [J]. Journal of Crystal Growth, 2001, 229(4): 433-439.
[9] KEITARO M, TAKIO N, NAZRUL M I, YUKIYA H, HIROMICHI H. Rapid synthesis of BaTiO3 nanoparticles in supercritical water by continuous hydrothermal flow reaction system [J]. Journal of Crystal Growth, 2008, 310(10): 2584-2589.
[10] KWON S W, YOON D H. Effects of heat treatment and particle size on the tetragonality of nano-sized barium titanate powder [J]. Ceramics International,2007, 33(7): 1357-1362.
[11] GALLAGHER P K, SCHREY F, DIMACELLO F V. Preparation of semiconducting titanates by chemical methods [J]. Journal of American Ceramic Society, 1963, 46(8): 359-365.
[12] QUAN Xue-jun, LI Da-cheng. Preparation of ultrafine barium titanate powder by a modified oxalate method [J]. Journal of Sichuan University: Engineering Science, 2001, 33(4): 78-81. (in Chinese)
[13] LUO Jian-hui. The preparation of electronic and ceramic barium titanate powder [J]. Materials Review, 1993, 15(5): 51-53. (in Chinese)
[14] ZHOU Zhao-hui, HONG Qi-ming, DENG Yuan-fu. Pure Ti-based mixed oxides prepared from the thermal decompositions of molecular precursors of peroxo complexes [J]. Acta Chimica Sinica, 2004, 62(24): 2379-2385. (in Chinese)
[15] JORDAN K D, BURROW P D. Studies of the temporary anion states of unsaturated hydrocarbons by electron transmission spectroscopy [J]. Accounts of Chemical Research, 1978, 11(2): 341-348.
[16] DAKANALI M, RAPTOPOULOU M C, TERZIS A, LAKATOS A, BANYAI I, KISS T, SALIFOGLOU A. A novel dinuclear species in the aqueous distribution of aluminium in the presence of citrate [J]. Inorganic Chemistry, 2003, 42(2): 252-254.
[17] EHRE D, LYAHOVITSKAYA V, LUBOMIRSKY I. The influence of the Ti/Ba ratio on the formation of pyroelectric and piezoelectric quasi-amorphous films of BaTiO3 [J]. Journal of Materials Research, 2007, 22(10): 2742-2746.
[18] KOYAMA M, MURAKAMI S, IWAJI N, TAKEBE H, KUWABARA M. The state and optical properties of crystalline BaTiO3 nanoparticles dispersed in various suspension media [J]. Key Engineering Materials, 2007, 350(38): 51-54.
[19] ALVARADO E, TORRES-MARTINEZ L M, FUENTES A F, QUINTANA P. Preparation and characterization of MgO powders obtained from different magnesium salt and the mineral dolomite [J]. Polyhedron, 2000, 19(22/23): 2345-2351.
[20] JUNG Y J, LIM D Y, NHO J S, CHO S B, RICHARD E R, BYEONG W L. Glycothermal synthesis and characterization of tetragonal barium titanate [J]. Journal of Crystal Growth, 2005, 274(42): 638-652.
(Edited by YANG Hua)
Foundation item: Project(06JJ50150) supported by the Hunan Provincial Natural Science Foundation of China
Received date: 2007-12-10; Accepted date: 2008-03-18
Corresponding author: YAN Wen-bin, Professor; Tel: +86-743-8563911; E-mail: jishouyanwenbin@163.com