
Electrochemical performance of carbon nanotube-modified
LiFePO4 cathodes for Li-ion batteries
CHEN Zhao-yong(陈召勇), ZHU Hua-li(朱华丽), ZHU Wei(朱 伟),
ZHANG Jian-li(张建利), LI Qi-feng(李奇峰)
College of Physics and Electronic Science, Changsha University of Science and Technology,
Changsha 410114, China
Received 29 October 2009; accepted 11 January 2010
Abstract: Carbon nanotubes (CNTs) and acetylene black (AB) were dispersed synchronously or separately between LiFePO4 (LFP) particles as conducting agents during the course of manufacture of LiFePO4 cathodes. The morphology and electrochemical performances of as-prepared LiFePO4 were evaluated by means of transmission electron microscopy (TEM), charge-discharge test, electrochemical impedance spectroscope (EIS) and cyclic voltammetry (CV). CNTs contribute to the interconnection of the isolated LiFePO4 or carbon particles. For the CNTs-modified LiFePO4, it exhibits excellent performance in terms of both specific capacity and cycle life. The initial discharge capacity is 147.9 mA?h/g at 0.2C rate and 134.2 mA?h/g at 1C rate, keeping a capacity retention ratio of 97% after 50 cycles. The results from EIS indicate that the impedance value of the solid electrolyte interface decreases. The cyclic voltammetric peak profiles is more symmetric and spiculate and there are fewer peaks. CNTs are promising conductive additives candidate for high-power Li-ion batteries.
Key words: LiFePO4; carbon nanotubes; cyclic voltammetry (CV); electrochemical impedance spectroscopy (EIS); Li-ion batteries
1 Introduction
Olivine LiFePO4 (LFP) is one of the most promising cathode candidates for Li-ion batteries, especially in electric vehicles, hybrid electric vehicles and so on. It has many advantages, such as cheapness, environment friendliness, safety with good stability[1]. So, it has attracted much attention all over the world in the recent decade. Unfortunately, it suffers from a serious defect of so much low intrinsic electric conductivity, which has become a huge obstacle to its extensive application. Two main methods are employed to improve its electric conductivity, one is to coat carbon on the surface of LiFePO4 particles, another is to introduce other metal ions into the crystal lattice of LiFePO4[2-7]. The former was identified to improve its conductivity, but perhaps this method only improves the conductivity between the LiFePO4 particles, which does not really improve the intrinsic electric conductivity of LiFePO4 particles[8-10]. And the latter method obviously improves the intrinsic conductivity, but much work is still required to explore.
On going research works still mainly focus on improving the rate performance of LFP[11-13]. Carbon nanotubes have surprisingly high electrical conductivity in the axial direction, and because of their special structure, many free electrons are distributed on the surface of the nanotubes, which can move freely. Carbon nanotubes (CNTs)[14-18] and carbon fibers[19-20] are adopted to improve the high-power performances of LiFePO4 or LiCoO2 cathodes. CNTs were coated on the surface of LiFePO4 by a hydrothermal method followed by heat treatment during the course of the preparation of cathode [14-15], or dispersing separately into LiFePO4 particles as conductive agent to make positive electrode slurry [16-18]. In this work, LiFePO4 is prepared by a simple solid state reaction, then CNTs and acetylene black (AB) are dispersed synchronously or separately between LiFePO4 particles as conductive agent during the course of manufacture of LiFePO4 cathode slurry. Cyclic voltammetry (CV) and electrochemical impedance spectroscope (EIS) are employed to understand the reason for this effect. It is expected that carbon nanotubes, with more free electrons on their surface, could remarkably improve the rate performance of the cathode.
2 Experimental
Lithium iron(Ⅱ) phosphate (LiFePO4, LFP) was prepared by a solid state reaction. The stoichiometric masses of CH3COOLi, NH4H2PO4, Fe(CH3COO2)2 and sucrose(C12H22O11)(all chemicals of 99% purity) were thoroughly mixed and ground for 10 h in steel ball mill with alcohol as dispersing medium. The as-prepared mixture was heated at 60 ℃ to evaporate the alcohol. Then after carefully regrinding, the mixture was calcined at 700 ℃ for 24 h in a nitrogen atmosphere. The molar ratio of Li:Fe:P was designed to be 1:1:1, and as a result of ICP-AES combined with chemical analysis, which was 1.08:1.00:1.02.
The positive electrode consisted of 80% as-prepared compounds and 10% conductive agent and 10% polytetrafluoroethylene (PTFE) as a binder, and metal Al was used as the current collector. The conductive agent was acetylene black (AB), or a combination of acetylene black and carbon nanotubes (CNTs) in the ratio of 1:1 by mass, or pure carbon nanotubes. (The cathode with CNTs and AB added as the conductive agent is called CNTs-modified LiFePO4, and that with AB is called non modified LiFePO4 in this paper). The electrolyte solution was 1.0 mol/L LiPF6/EC+DMC[V(EC):V(DMC)=1:1]. Lithium metal foil was used as the counter electrode during electrochemical measurements. All cells were assembled in an argon-filled glove box. The electrochemical tests were carried out in a Land-BTL10 fully automatic program test instrument using the voltage range of 2.50-3.90 V at room temperature, with the testing rates of 0.2C and 1C (1C-rate corresponds to a current density of 170 mA/g over a period of 1 h). The morphology of the CNTs-modified LFP composites was studied by transmission electron microscopy (TEM, JEOL JEM3010). The contents of elements were detected by inductively coupled plasma atomic emission spectrometry (ICP-AES, IRIS Advantage 1000) and chemical analysis method. The electrochemical impedance spectroscopy (EIS) of the cells was measured in the frequency range of 1 mHz-100 kHz. The cyclic voltammetry (CV) of the cells was carried out at a scan rate of 0.01 mV/s between 2.5 and 4.3 V. EIS and CV analysis were both carried out using a CHI660B electrochemical work station (Chenhua, Shanghai)
3 Results and discussion
The X-ray diffraction pattern of LiFePO4 material is shown in Fig.1. All diffraction lines can be attributed to the orthorhombic olivine type phase LiFePO4 (PDF NO. 81-1173), and nearly no impurity was detected.
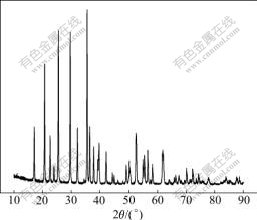
Fig.1 XRD pattern of LiFePO4
The as-prepared LiFePO4 combined with acetylene black and carbon nanotubes was dispersed by a magnetic stirring for 2 h in the suspended PTFE solution to obtain the CNTs-modified LiFePO4 cathode. The TEM images of LiFePO4 are shown in Fig.2. It can be observed from Figs.2(a) and (b) that amorphous carbon is wrapped on the surface of the as-prepared LiFePO4 particles, even so, a large amount of voids could still be found between the LiFePO4 and carbon particles, which is harmful to higher conductivity; and in images of CNTs-modified LiFePO4 (Figs.2(c) and (d)), LiFePO4 particles are dispersed in the network consisting of carbon nanotubes and amorphous carbon. There are always some voids between these particles if CNTs are not added. The structure of nanotubes contributes to the form of network, which can interconnect the isolated particles, so that the electrons can be readily transmitted to the sites where redox reactions take place. For this reason, CNTs-modified LiFePO4 have large current charge-discharge capacity as it will be shown and discussed in the following section.
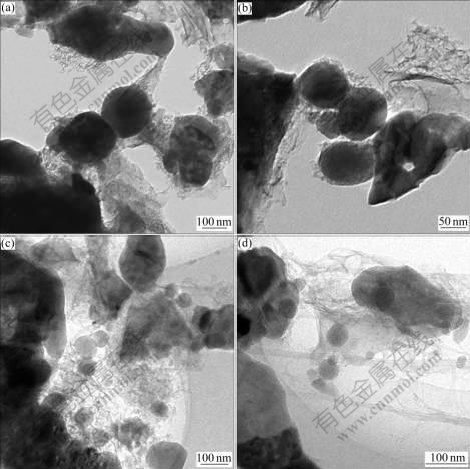
Fig.2 TEM images of LiFePO4: (a, b) As-prepared LiFePO4; (c, d) CNTs-modified LiFePO4
Fig.3 shows the electrochemical cycling performance of the CNTs-modified LiFePO4 or not at 0.2C for 10 cycles and 1C for 100 cycles. At 0.2C rate, the cathodes reach the reversible capacity of 141.7, 147.9 and 141.2 mA?h/g for LFP+AB, LFP+CNTs+AB and LFP+CNTs, respectively. They all exhibit good cycling performance during the first 10 cycles. At the rate of 1C, the cathodes reach the reversible capacity of 120.1, 134.2 and 130.1 mA?h/g for LFP+AB, LFP+CNTs+AB and LFP+CNTs, respectively. After 50 cycles, the cathodes maintain 104.9, 130.7 and 110.8 mA?h/g with the capacity retainability of 87%, 97% and 85%, respectively. LFP+CNTs+AB cathode has the best cycling performance, keeping around 120 mA?h/g at the 100th cycle.
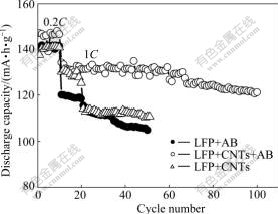
Fig.3 Electrochemical cycling performance of LiFePO4 cathode at various rates
Many free electrons concentrate on the surface of the carbon nanotubes, which contributes to the electrical conductivity of the CNTs-modified LiFePO4. In the CNTs-modified LiFePO4, the LiFePO4 particles are dispersed in the network of CNTs and AB. Due to this phenomenon, electrons are transmitted to the electrochemical reaction sites, where Fe2+ is oxidized to Fe3+ reversibly. The results show that CNTs-modified LiFePO4 exhibit much higher discharge capacity and excellent cycle stability at different discharge rates. The discharge capacity decreases sharply for the non CNTs-modified LiFePO4, especially at the discharge rate of 1C.
To compare the effect of CNTs on the electrochemical performance of the LFP cathode in more detail, the electrochemical impedance measurements and cyclic voltammetry were carried out. Fig.4 shows the electrochemical impedance spectra of CNTs-modified and non modified LiFePO4 during the charge and discharge process at the rate of 0.2C. The shapes of EIS of CNTs-modified and non modified LiFePO4 are similar, which consist of a depressed semicircle at high frequency and a straight line at low frequency. The values of the cross point of the semicircle on the horizontal axes represent the charge transfer resistance. The straight line represents the Warburg impedance, which is associated with the lithium-ion diffusion in the inner of LiFePO4 particles. The charge transfer resistance will now be thoroughly discussed, which has a close relationship between composition and conductivity of the interface. In the case of non modified LiFePO4, in spite of the states of charge, the impedance effectively does not change during charging. There is a very small difference between the discharge process and the charge process, and the impedance gradually increases with the process of discharge, although the value of increase is not comparable with the whole impedance. The slow increase with discharge could originate from an unstable interface, in which the side reactions continue to take place. However, in another case of CNTs-modified LiFePO4, the charge transfer resistance at high frequency drops sharply in all the states of charge compared with non modified LiFePO4, and it seems that the state of charge has no obvious effect on the charge transfer resistance. It is obvious that the carbon nanotubes have played an important role in accelerating the transfer of charge. There is also a sudden increase of the charge transfer resistance from the state of charge to that of discharge. An extraordinarily large difference is observed in the state of discharge. With the process of discharge, the charge transfer resistance decreases gradually. It is probably the enhanced electronic conductivity and better contact efficiency between the isolated particles of CNTs-modified LiFePO4 cathode that decrease the resistance originating from charge transfer. So, the drop of resistance favors the improved performances, which gives the main explanation for the excellent performance of CNTs-modified-LiFePO4 at high rate.
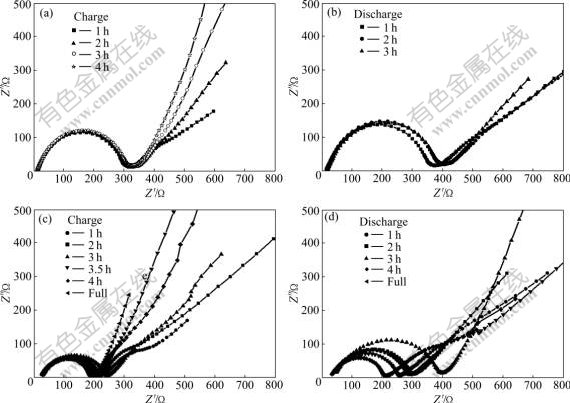
Fig.4 Electrochemical impedance spectra of cathodes: (a) Non modified LiFePO4; (b) Non modified LiFePO4; (c) CNTs-modified LiFePO4; (d) CNTs-modified LiFePO4
Fig.5 shows the cyclic voltammetry profiles of CNTs-modified and non modified LiFePO4 cathodes in the third cycle at 0.1 mV/s and 2.5-4.3 V. They both exhibit a pair of redox peaks around 3.4 V vs Li+/Li, and the anodic and cathodic peaks correspond to the two-phase charge-discharge reaction of the Fe2+/Fe3+ redox couple. In addition, there are small anodic peaks above 3.8 V in the scanning curve of non modified LFP, the reason for this is still unknown at present. The peak profiles of CNTs-modified LFP cathode are more symmetric and spiculate, indicating a smaller conductivity restriction than that for non modified LFP cathode. The well-defined peaks and smaller value of potential interval of CNTs-modified LFP show the enhancement of electrode reaction reversibility. This result is in agreement with the electrochemical cycling performance of the LiFePO4 cathode. In CNTs-modified LiFePO4, the interconnected network is formed, and the conducting environment of lithium ion is higher, so during the scanning process, the transmission impedance of lithium ion and electrons decreases.
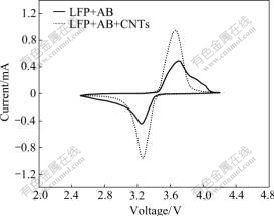
Fig.5 Cyclic voltammetry profiles of cathodes in 3rd cycle at 0.1 mV/s and 2.5-4.3 V
4 Conclusions
1) Carbon nanotubes (CNTs) and acetylene black (AB) were used as conducting agents during the course of manufacture of LiFePO4 cathodes, and the performances of the cells were evaluated. The cells exhibit performances superior to those not containing CNTs in terms of both high-rate (1C) performances and cycle life with the initial discharge capacity of 147.9 mA?h/g at 0.2C rate and 134.2 mA?h/g at 1C rate, keeping a capacity retention ratio of 97% after 50 cycles.
2) Results from AC impedance indicate that the impedance values for the solid electrolyte interface between the CNTs-modified LFP cathode and electrolyte solution decrease.
3) In the cyclic voltammograms, the peak profiles of CNTs-modified LFP cathode are more symmetric and spiculate than those of non modified LFP, and there are no other peaks observed in the case of CNTs-modified LiFePO4, which are present in the case of non modified LiFePO4. Overall, CNTs are promising candidates for conducting agents of high-power Li-ion batteries.
References
[1] Padhi A K, Nanjundaswamy K S, Goodenough J B. Phospho-olivines as positive-electrode materials for rechargeable lithium batteries [J]. Journal of the Electrochemical Society, 1997, 144: 1188-1193.
[2] HU Guo-rong, GAO Xu-guang, PENG Zhong-dong, DU Ke, TAN Xian-yan, LIU Yan-jun. Influence of Ti4+ doping on electrochemical properties of LiFePO4/C cathode material for lithium-ion batteries [J]. Transactions of Nonferrous Metals Society of China, 2007, 17(2): 296-300.
[3] WU Ling, WANG Zhi-xing, LI Xin-hai, LI Ling-jun, ZHENG Jun-chao, GUO Hua-jun, LIU Jiu-qing, Preparation of Ti+4-doped LiFePO4 by precursor-doping and room temperature reduction via ball-milling [J]. Journal of Central South University (Science and Technology), 2009, 40(2): 288-293. (in Chinese)
[4] CHEN Han, YU Wen-zhi, HAN Shao-chang, XU Zhong-yu. Preparation and electrochemical properties of LiFePO4/C composite with network structure for lithium ion batteries [J]. Transactions of Nonferrous Metals Society of China, 2007, 17(5): 951-956.
[5] LIU Xu-heng, ZHAO Zhong-wei. Effects of carbon source and iron source on preparation and performance of LiFePO4/C [J]. The Chinese Journal of Nonferrous Metals, 2008, 18(3): 541-545.
[6] Delacourt C, Wurm C, Laffont L, Leriche J B, Masquelier C. Electrochemical and electrical properties of Nb- and/or C-containing LiFePO4 composites [J]. Solid State Ionics, 2006, 177(3/4): 333-341.
[7] Liu Hui, Xie Jing-ying. Synthesis and characterization of LiFe0.9Mg0.1PO4/nano-carbon webs composite cathode [J]. Journal of Materials Processing Technology, 2009, 209(1): 477-481.
[8] Chung S Y, Bloking J T, Chiang Y M. Electronically conductive phospho-olivines as lithium storage electrodes [J]. Nature Materials, 2002, 1: 123-128.
[9] Wang De-yu, Li Hong, Shi Si-qi, Huang Xue-jie, Chen Li-quan. Improving the rate performance of LiFePO4 by Fe-site doping [J]. Electrochim Acta, 2005, 50(14): 2955–2958.
[10] Chen Zhao-yong, Zhu Hua-li, Ji Shan, Fakir R, Linkov V. Influence of carbon sources on electrochemical performances of LiFePO4/C composites [J]. Solid State Ionics, 2008, 179: 1810-1815.
[11] Kang B, Ceder G. Battery materials for ultrafast charging and discharging [J]. Nature, 2009, 458: 190-193.
[12] Huang Yang-hui, Ren Hai-bo, Yin Sheng-yu, Wang Yun-hong, Peng Zheng-he, Zhou Yun-hong. Synthesis of LiFePO4/C composite with high-rate performance by starch sol assisted rheological phase method [J]. Journal of Power Sources, 2010, 195(2): 610-613.
[13] Liu Hui, Xie Jing-ying, Wang Ke. Synthesis and characterization of nano-LiFePO4/carbon composite cathodes from 2-methoxyethanol–water system [J]. Journal of Alloys and Compounds, 2008, 459(1/2): 521-525.
[14] JIN Bo, JIN En-mei, PARK Kyung-Hee, GU Hal-Bon. Electro- chemical properties of LiFePO4-multiwalled carbon nanotubes composite cathode materials for lithium polymer battery [J]. Electrochemistry Communications, 2008, 10(10): 1537-1540.
[15] XU Jing, CEHN Gang, LI Xin. Electrochemical performance of LiFePO4 cathode material coated with multi-wall carbon nanotubes [J]. Materials Chemistry and Physics, 2009, 118(1): 9-11.
[16] LIU Yun-jian, LI Xin-hai, GUO Hua-jun, WANG Zhi-xing, PENG Wen-jie, YANG Yong, LIANG Ru-fu. Effect of carbon nanotube on the electrochemical performance of C-LiFePO4/graphite battery [J]. Journal of Power Sources, 2008, 184(2): 522-526.
[17] LI Xin-lu, KANG Fei-yu, BAI Xin-de, SHEN Wan-ci. A novel network composite cathode of LiFePO4/multiwalled carbon nanotubes with high rate capability for lithium ion batteries [J]. Electrochemistry Communications, 2007, 9(4): 663-666.
[18] SHEEM K, LEE Y H, LIM H S. High-density positive electrodes containing carbon nanotubes for use in Li-ion cells [J]. Journal of Power Sources, 2006, 158(2): 1425-1430.
[19] Thorat I V, Mathur V, Harb J N, Wheeler D R. Performance of carbon-fiber-containing LiFePO4 cathodes for high-power applications [J]. Journal of Power Sources, 2006, 162(1): 673-678.
[20] Bhuvaneswari M S, Bramnik N N, Ensling D, Ehrenberg H, Jaegermann W. Synthesis and characterization of carbon nano fiber/LiFePO4 composites for Li-ion batteries [J]. Journal of Power Sources, 2008, 180(1): 553-560.
Foundation item: Project(06B002) supported by Scientific Research Fund of Hunan Provincial Education Department of China; Project(09JJ3092) supported by Hunan Provincial Natural Science Foundation of China; Project(2008FJ3008) supported by the Planned Science and Technology Project of Hunan Province of China
Corresponding author: CHEN Zhao-yong, Tel: +86-731-85258234; Fax: +86-731-85258217; E-mail: chenzhaoyongcioc@126.com
DOI: 10.1016/S1003-6326(09)60187-4
(Edited by LI Xiang-qun)