
Effect of Yb addition on precipitation and microstructure of Al-Cu-Mg-Ag alloys
XIAO Dai-hong(肖代红)1, 2, HUANG Bai-yun(黄伯云)2
1. State Key Laboratory of Powder Metallurgy, Central South University, Changsha 410083, China;
2. Laboratory of Material Physics, Zhengzhou University, Zhengzhou 450052, China
Received 15 July 2007; accepted 10 September 2007
Abstract: Al-Cu-Mg-Ag alloys with different Yb contents were prepared by ingot metallurgy and thermomechanical treatment. The effect of Yb addition on the precipitation and microstructure of the alloys was investigated using mechanical properties testing, optical microscopy, differential scanning calorimetry(DSC), scanning electron microscopy(SEM) and transmission electron microscopy(TEM). The results show that adding 0.10%-0.35% Yb accelerates the aging hardening process, increases the maximum hardness and the tensile strength of the extruded alloys from room temperature to 300 ℃. Trace Yb element addition refines the grains of the casting alloys from 85 μm to 30 μm, decreases the precipitation temperature of Ω phase. Moreover, the addition of Yb into Al-Cu-Mg-Ag alloy decreases the precipitates size, and improves the density and the thermal stability of Ω phase between 200 ℃ and 300 ℃.
Key words: aluminum alloy; Yb addition; precipitation; microstructure; mechanical properties
1 Introduction
Current understanding of structure—properties relationship in age hardenable Al alloys suggests that optimization of grain structure and the presence of a fine and uniform distribution of both shearable and nonshearable precipitates in the matrix are important in obtaining a balanced set of mechanical properties[1-2].
The addition of Ag has proved to have a more remarked effect than Fe and Ni on the high-temperature strength of Al-Cu-Mg alloys with high Cu-to-Mg molar ratios (e.g. 10?1)[3-4]. The addition of Ag not only enhances the hardening response but also totally changes the precipitation processes. In particular, it promotes the formation of a fine and uniform dispersion of hexagonal-shaped plate-like precipitates on {111} planes of the matrix, but at the expense of the precipitation of θ′(Al2Cu) usually observed in the Al-Cu-Mg alloys[3-7]. It is the fine and uniform distribution of the new precipitate (designated as Ω) and its good thermal stability that lead to superior strength of the alloys at temperatures up to 250 ℃[8-10].
The structure of Ω phase has been a subject of some studies. At first, it was proposed to be a θ-Al2Cu phase, with a monoclinic unit cell[11]. Later, it was described as being hexagonal[3], or orthorhombic[12]. More recently, it was suggested that the structure of Ω phase is tetragonal with a=b=0.606 6 nm and c=0.496 nm based on convergent beam electron diffraction results[13]. It should be mentioned that although several models were proposed for the structure of Ω phase, the differences among them are not significant[14].
Rare earth elements in conventional casting aluminum alloys have shown beneficial effects on melting and solidification. These elements reduce the contents of gases and some impurities, and the spacing between secondary dendrite arms. It was reported that the Ce content affected the grain size and the mechanical properties of Al-Cu-Mg-Mn-Ag alloys[15]. The Yb addition improved the mechanical properties of Al-Zn-Mg-Cu-Zr alloys. It was also reported that the Yb addition affected the precipitation and mechanical properties of Al-0.06% (molar fraction) Sc alloys[16].
Compared with that for transition metals, there is little information available for the effect of rare earth Yb ddition on the microstructure and properties of Al-Cu-Mg-Ag alloys. The present research was thus designed to examine such an effect with a view of establishing the potential for enhancing the mechanical properties of these alloys.
2 Experimental
Table 1 lists the normal compositions of the present alloys with Yb additions of 0.0%, 0.10% and 0.35% (mass fraction). The alloys were melted by induction heating. The temperature of alloy melt was kept between 720 ℃ and 750 ℃. The melt was degassed by adding C2Cl6 for 10-15 min, and then cast into iron moulds to produce billets of 200 mm in length and 60 mm in diameter. The billets were homogenized at 490 ℃ for 48 h, followed by air cooling to room temperature, and then hot extruded to bars of 15 mm in diameter at 380 ℃. Subsequently, the bars were solution treated at 525 ℃ for 10 h, water quenched, and finally aged for different periods of time between room temperature and 180 ℃.
Table 1 Nominal compositions of present alloys (mass fraction, %)
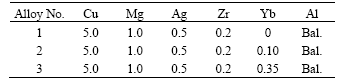
Tensile tests were performed at 25 ℃ and 300 ℃ at a strain rate of about 10-4 s-1 on a Shimadzu AG-100KN machine. The specimens used were those peak-aged and had a gauge diameter of 5 mm and a gauge length of 25 mm. In the tests at high temperatures, the specimens were held at these temperatures for about 20 min before loading to achieving thermal equilibration in the furnace. The mechanical results reported in this study are the mean values of at least two specimens.
Optical microscopy studies were carried out in a LEICA MEF4A/M type microscope. Transmission electron microscopy(TEM) was applied to characterize the microstructure of the alloys after peak aging. TEM samples were electro-polished, in a 70% ethanol and 30% nitric acid solution at -30 ℃, by using twin-jet equipment operated at 20 V.
3 Results
Fig.1 shows the grain structures of the present alloys in the as-cast state. The average grain sizes of alloys 1, 2 and 3 are about 85, 35 and 30 mm, respectively. Adding 0.10%Yb or 0.35%Yb obviously refines the grain size of the casting alloy. Furthermore,
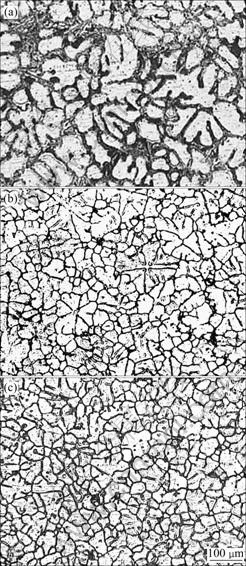
Fig.1 Grain structures of as-cast alloys: (a) Alloy 1; (b) Alloy 2; (c) Alloy 3
there is grain boundary segregation in the alloys with Yb. Energy dispersive X-ray(EDX) analysis (Fig.2) shows that the segregated phase is rich in Yb. These microstructural features, including the grain boundary segregates and grain size, do not show significant modification after solution treatment at 525 ℃ and subsequent aging at 180 ℃.
Fig.3 shows the variation of hardness with aging time at 180 ℃ for present alloys containing Yb. Different behavior is observed because of the effect of Yb addition on the aging response when compared with the Yb-free alloy. The hardening response of the alloy is enhanced by the addition of Yb. In particular, the maximum hardness increases from about HV161 for the Yb-free alloy to HV181 for that containing 0.10%Yb. However, the maximum hardness is reduced to about HV176 when the
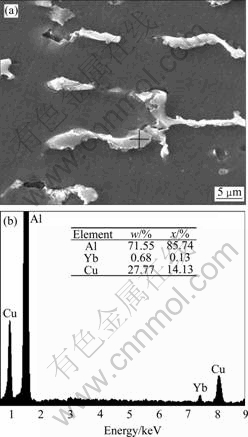
Fig.2 SEM image (a) and EDX pattern (b) of as-cast alloy 3
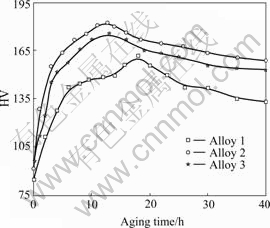
Fig.3 Hardness curves of present alloys after aging at 185 ℃
Yb content is further increased to 0.35% (Fig.3). Meanwhile, the peak-aging time for the Yb-free alloy is about 18 h, but that for Yb containing alloys is about 12 h. Furthermore, alloy 1 shows two aging stages before reaching the maximum hardness, but with Yb addition, there is only one hardening stage.
Fig.4 represents differential scanning calorimetry (DSC) traces for alloys 1 and 2 after solution treatment. It can be seen that Yb addition shifts the trace (peak P)
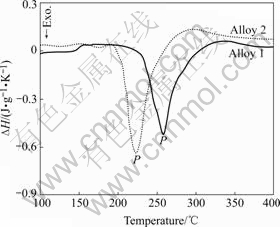
Fig.4 DSC traces for alloys 1 and 2 (heating rate 10 ℃/min)
position due to the face that the exotherm associated with precipitation of Ω phase occurs at different temperatures for different alloys. When the Yb addition is increased from 0 to 0.10%, the peak P shifts to a lower temperature. This suggests that adding Yb might promote the precipitation reaction.
Tensile testing was made at temperatures up to 300℃. The results are shown in Fig.5. At room temperature, the tensile strength is increased from 506 MPa to 545 MPa when the Yb content is increased up to 0.10%. However, it is increased only slightly when the Yb content is increased from 0.10% to 0.35%. The changing tendency of tensile strength with Yb content at high temperatures up to 300 ℃ is similar to that at room temperature. At 300 ℃, the tensile strength of alloys 2 and 3 is over 230 MPa, but that for alloy 1 is only 192 MPa.
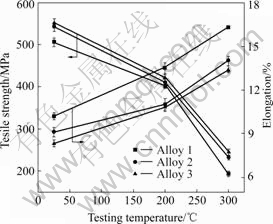
Fig.5 Mechanical properties of present alloys after peak aging at 180 ℃
Meanwhile, with increasing Yb content in the present alloys, the elongation is decreased. The elongation of alloys 2 and 3 is generally lower than that of alloy 1. In the testing range between room temperature and 300 ℃, the elongation of alloy 1 is increased from 10.2% to 16.3%, that for alloy 2 is increased from 9.1% to 14%, and that of alloy 3 from 8.3% to 13.4%. Since these data are the mean values of at least two specimens, and the error associated with the measurement is generally low, it could be concluded that increasing Yb content increases the elevated temperature strength but slightly decreases the elongation of Al-Cu-Mg-Ag alloys.
The microstructures of the present alloys under different conditions were investigated by TEM. Fig.6 shows the TEM images of alloys 1 and 3 after peak aging at 180 ℃. It is confirmed that the lath- or needle-shaped Ω phase that forms on the {111}α planes is the dominant precipitate in the present alloys. Meanwhile, a certain amount of θ′ phase as platelets on the {001}α plane is also observed. Nevertheless, the densities of Ω and θ′ phases in alloys 3 are higher than those in alloy 1. The Ω precipitate in alloy 3 is about 3-7 nm thick with aspect ratios up to 100, but is about 7-25 nm thick with aspect ratios up to 200 in alloy 1.
To further understand the difference in intrinsic feature of the Ω phase at high temperatures, alloys 1 and 3 were thermally exposed at 300 ℃ for 100 h. TEM results (Fig.6) show that in alloy 3, the Ω and θ′ precipitates are coarsened. However, the Ω precipitates in alloy 1 are all transformed to the θ phase. This observation indicates that the thermal stability of the Ω phase in alloys with Yb addition is better than that without Yb.
4 Discussion
The present work shows that the addition of Yb affects the microstructure development in the Al-Cu-Mg-Ag alloy in various ways. Firstly, with the addition of Yb, the size and density of the predominant hardening phases of Ω and θ′ are changed in the present alloys after peak-aging at 180 ℃. According to Fig.6, the density of these phases is higher and the size is smaller in alloy 3 with Yb than in alloy 1 without Yb. Secondly, with the addition of Yb, the hardening process is somehow altered. This is shown by the existence of a short period during which the hardness remains almost unchanged before reaching the peak for the Yb-free alloy. But such a period is not observed for the Yb-containing alloy (Fig.3). This may be due to the fact that Yb addition tends to retard the GP zone stage, and enhances Ω formation. Thirdly, the Yb addition affects the thermal stability of Ω phase (Fig.6). This is verified by the observation that after exposure at 300 ℃, there are Ω, θ′ phases in alloy 3, but there is only θ phase in alloy 1.
The present work also shows that the addition of Yb affects the mechanical behavior of the Al-Cu-Mg-Ag alloy. For example, both the hardness and tensile strength are increased with Yb addition (Figs.3 and 5). This may be a result of the enhanced precipitate strengthening of the finer and denser Ω and θ′ phases with Yb addition.
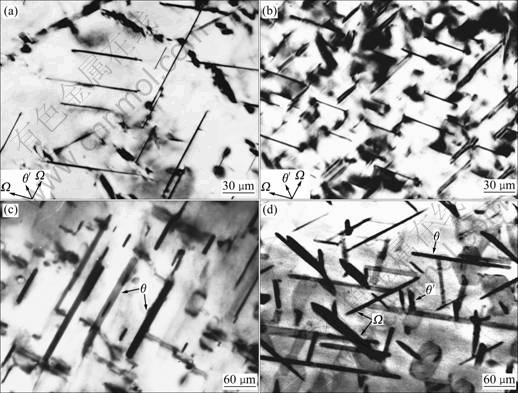
Fig.6 TEM images of alloys 1(a, c) and 3 (b, d) exposed at room temperature (a, b) and 300 ℃ for 100 h (c, d)
Additionally, the higher strength at high temperatures may further be related to higher thermal stability of Ω phase with the addition of Yb (Fig.6).
The dominant strengthening phases of the present alloys are Ω and the small amount of θ′ phases (Fig.6), and their composition is Al2Cu[4]. The Ω phase shows thermal stability at temperatures up to 200 ℃[8]. However, this phase coarsens, and its thermal stability decreases due to the redistribution of Ag and Mg and the diffusion of Cu over 200 ℃[17]. With the Yb addition in the Al-Cu-Mg-Ag alloy, the diffusion of Cu may be decreased, the coarsening rate of the Ω phase may be reduced, and the elevated temperature strength may thus be increased at high temperature (e.g. 300 ℃).
With the addition of Yb, the tensile strength is apparently increased for the present Al-Cu-Mg-Ag alloy. Although it has not been tested, with such addition, the creep resistance of the alloy could also be improved. This is because there is indication for the increase of thermal stability of Ω phase in the presence of Yb. Thus, the present alloys containing Yb may be used at higher temperatures than conventional Ce-free Al-Cu-Mg-Ag alloys.
5 Conclusions
1) Addition of Yb up to 0.35% to an Al-Cu-Mg-Ag alloy refines the grain structure, induces the precipitation of finer and denser Ω and θ′ phases when compared with the Yb-free case.
2) The addition of Yb improves the thermal stability of Ω phase in Al-Cu-Mg-Ag alloy. This may promote the service temperature of the conventional Al-Cu-Mg-Ag base alloys.
3) Yb addition accelerates the aging hardening process, increases the maximum hardness and the tensile strength of the extruded alloys from room temperature to 300 ℃.
References
[1] VASUDEVAN K, DOHERTY R D. Grain boundary ductile fracture in precipitation hardened aluminum alloys [J]. Acta Metall, 1987, 35(6): 1193-1216.
[2] ZHANG Kun, LIU Zhi-yi, FENG Chun. Effect of small addition of silver on microstructure and mechanical properties of high-zinc super-high strength aluminum alloy [J]. The Chinese Journal of Nonferrous Metals, 2005, 15(1): 116-122. (in Chinese)
[3] POLMEAR I J. The effects of small addition of silver on the aging of some aluminum alloys [J]. Trans Metall Soc AIME, 1964, 230(10): 1331-1339.
[4] POLMEAR I J, COUPER M J. Design development of an experimental wrought aluminum alloy for use at elevated temperatures [J]. Metall Trans, 1988, A19(4): 1027-1031.
[5] MUDDLE B C, POLMEAR I J. Precipitate Ω phase in Al-Cu-Mg-Ag alloys [J]. Acta Metall Mater, 1989, 37(93): 777-789.
[6] RING S P, SAKURAI T. Origins of hardening in aged Al-Cu-Mg-(Ag) alloys [J]. Acta Mater, 1997, 45(9): 3731-3744.
[7] SONG Min, CHEN Kang-hua, HUANG Lan-ping. Precipitation and growth dynamics of precipitates in Al-Cu-Mg-(Ag) alloy during aging [J]. Trans Nonferrous Met Soc China, 2006, 16(8): 1313-1319.
[8] ABIS S, MENGUCCI P, RIONTINO G. Influence of Si additions on the ageing process of an Al-Cu-Mg-Ag alloy [J]. Phil Mag, 1994, A70: 851-860.
[9] POLMEAR I J, HUTCHINSON C R, FAN X, PENNYCOOK S. On the origin of the high coarsening resistance of Ω plates in Al-Cu-Mg-Ag alloys [J]. Acta Mater, 2001, 49(14): 2827-2841.
[10] SHIFLET G J,
GABKE B M, SHIFLET G J, STARKE Jr. Alloy development for the enhanced stability of Ω precipitates in Al-Cu-Mg-Ag alloys [J]. Metall Mater Trans, 2006, 37(4): 1091-1105.
[11] AULD J H. Structure of a metastable precipitate in an Al-Cu-Mg-Ag alloy [J]. Acta Crystallogr, 1972, A28(S4): 98-105.
[12] SCOTT V D, KERRY S, TRUMPER R L. Nucleation and growth of precipitates in Al-Cu-Mg-Ag alloys [J]. Mater Sci Technol, 1987, 3(10): 827-835.
[13] GARGE A, HOWE M J. Convergent-beam electron diffraction analysis of the Ω phase in an Al-4.0Cu-0.5Mg-0.5Ag alloy [J]. Acta Metall, 1991, 39(8): 1939-1948.
[14] XIAO D H, CHEN Kang-hua, SONG Min. Effect of cerium addition on precipitation and microstructure of Al-Cu-Mg-Mn-Ag alloys [J]. The Chinese Journal of Nonferrous Metals, 2007, 17(5): 669-675. (in Chinese)
[15] CHEN Kang-hua, FANG Hua-chan, ZHANG Zhuo, HUANG Lan-pin. Effect of Yb additions on microstructures and properties of high strength Al-Zn-Mg-Cu-Zr alloys [J]. Mater Sci Forum, 2007, 546/549: 1021-1026.
[16] MARSHA E V, DAVID C D. Nanoscale precipitation and mechanical properties of Al-0.06at.% Sc alloys microalloyed with Yb or Gd [J]. J Mater Sci, 2006, 41(23): 7814-7823.
[17] HUTCHINSON C R, FAN X, PENNYCOOK S J, SHIFLET G J. On the origin of the high coarsening resistance of Omega plates in Al-Cu-Mg-Ag alloys [J]. Acta Mater, 2001, 49(14): 2827-2841.
(Edited by YANG Bing)
Foundation item: Project(20070410986) support by Postdoctoral Research Foundation of China; Project(ZMPL07SW0002) support by Laboratory of Material Physics of Zhengzhou University, China
Corresponding author: XIAO Dai-hong; Tel: +86-731-8836773; E-mail: daihongx@mail.csu.edu.cn