Trans. Nonferrous Met. Soc. China 24(2014) 1890-1897
Effect of initial pH on chalcopyrite oxidation dissolution in the presence of extreme thermophile Acidianus manzaensis
Chang-li LIANG1,2, Jin-lan XIA2,3, Zhen-yuan NIE2,3, Shui-jing YU1, Bao-quan XU1
1. Faculty of Resource and Environment Engineering, Jiangxi University of Science and Technology, Ganzhou 341000, China;
2. School of Minerals Processing and Bioengineering, Central South University, Changsha 410083, China;
3. Key Laboratory of Biometallurgy of Ministry of Education of China, Central South University, Changsha 410083, China
Received 21 June 2013; accepted 5 November 2013
Abstract: The influence of initial pH on the chalcopyrite oxidation dissolution at 65 ℃ was investigated by bioleaching and cyclic voltammetry experiments, and the oxidation products were investigated by XRD and Raman spectroscopy. Bioleaching results show that chalcopyrite dissolution rate increases with the decrease of the initial pH in chemical leaching, while the influence of initial pH on bioleaching is on the contrary. The presence of Acidianus manzaensis does not promote chalcopyrite dissolution under initial pH 1.0, which mainly results from serious inhibition of high acidity to the growth of Acidianus manzaensis. Electrochemical experiments results show that anodic oxidation currents of electrolyte with or without Acidianus manzaensis both increase with the increase of initial pH, and covellite and sulfur are detected on the electrode surface. The results confirm that chalcopyrite dissolution in chemical leaching is under the combined action of oxidation and non-oxidation of proton, with conversion of chalcopyrite to covellite and elemental sulfur.
Key words: chalcopyrite; bioleaching; Acidianus manzaensis; initial pH; oxidation dissolution
1 Introduction
Bioleaching technology has been successfully applied to the extraction of copper from low-grade secondary copper sulfide chalcocite. However, bioleaching of chalcopyrite, the most abundant primary copper sulfide, has not been successful on a commercial scale, which is mainly due to the low leaching kinetics and copper recovery by typical mesophiles [1]. Bioleaching of chalcopyrite with thermophiles may effectively promote the dissolution of chalcopyrite [2].
It is well known that chalcopyrite is acid-soluble, and it dissolves under the oxidation action of Fe3+ and proton attack via polysulfide mechanism. Therefore, pH is an important influence factor for chalcopyrite bioleaching. Some researchers reported that chalcopyrite leaching rate increases with the increase of acidity in chemical leaching [3,4]. While,
et al [5] and
[6] found that high acidity hampers the dissolution of chalcopyrite in chemical leaching. The influence of pH in chalcopyrite bioleaching is more complex than in chemical leaching, which affects not only the dissolution of chalcopyrite, but also the oxidation activity and growth of microorganisms.
Chalcopyrite (bio) leaching can be looked upon as electrochemical anodic oxidation and cathodic reduction reactions, thus it can be studied by electrochemical methods. It was reported that chalcopyrite anodic oxidation currents increase with the increase of pH [7-9]. However, ZHU et al [10] suggested that the current of the redox reaction increases with the decrease of pH. The influence of initial pH on chalcopyrite bioleaching and anodic oxidation is still unclear.
In the present study, the influence of initial pH on chalcopyrite oxidation dissolution at 65 °C was investigated by (bio) leaching and cyclic voltammetry experiments, and the solid products formed during bioleaching and on the electrode surface were studied by XRD and Raman spectroscopy, respectively.
2 Experimental
2.1 Chalcopyrite concentrate
Chalcopyrite concentrate used in this study was the same as that by HE et al [11], and the particle size of sample was less than 75 μm.
2.2 Strain and culture medium
The strain A. manzaensis YN-25 used in this study was isolated by the Key Laboratory of Biometallurgy of Ministry of Education of China, Changsha, and the characteristics of the strain were described by DING et al [12]. A. manzaensis YN-25 was cultured in 9K basal medium supplemented with 0.02% (w/v) yeast extract, and it was gradually adapted to 0.5% (w/v) chalcopyrite for several months before using in bioleaching experiments. The cells were harvested by filtration (through 5 μm filter membrane) and centrifugation (10000 r/min) for 30 min, and the cell pellets were washed twice with sulfuric acid (0.1 mol/L) and suspended in sterilized fresh medium.
2.3 Leaching experiments
All leaching experiments were carried out in 250 mL Erlenmeyer flasks containing 100 mL sterilized medium at the pulp density of 2%. Chemical leaching experiments were conducted at three pH values (1.0, 1.5 and 2.0). The conditions of bioleaching were taken as those of chemical leaching except A. manzaensis and 0.02% (w/v) yeast extract was provided. The initial cell concentration in all the bioleaching experiments was 1×107 mL-1. The cultures were incubated at a high-temperature water bath rotary shaker at 125 r/min and 65 °C. Triplicate experiments were performed under identical conditions. The water evaporation and the loss due to sampling for analyses were compensated with sterilized fresh medium.
Cell number was determined by blood cell counting chamber. The concentrations of total iron and copper ions were determined by atomic absorption spectrophotometry (AAS), and the concentrations of ferrous ions were determined by titration with potassium dichromate. The pH values of leaching solution were measured with pH meter (PHS-3C). Redox potential was measured with Pt electrode, using calomel electrode (Hg/Hg2Cl2) as reference. The preparation of samples for XRD analysis was performed as that by XIA et al [13].
2.4 Electrochemical experiments
The preparation of electrode, electrolyte, reagents, electrochemical cell and electrochemical workstation were the same as those in our previous work [14]. The 9K basal medium was used as the electrolyte and its pH was adjusted to conducted values with 1 mol/L sulfuric acid before electrochemical experiments. The temperature of electrochemical measurement was maintained at 65 °C with a thermostat.
Open circuit potential (OCP) of the massive chalcopyrite electrodes was measured, which was 0.422 V (vs SHE) after reaching its steady state for 30 s. Cyclic voltammetry experiment was performed at a potential sweep rate of 20 mV/s, scan was initiated from the OCP in positive direction, without stirring the electrolyte solution. All potentials in this work were measured (vs SHE).
Potentiostatic oxidation modification of the electrode at 0.607 V for 4 min, and preparation of the electrode sample for Raman spectroscopy analysis were performed as those in our previous work [14].
2.5 Mineral morphology and composition analyses
XRD and Raman spectroscopy analyses of the anodic oxidation products formed on the massive chalcopyrite electrode surface were performed as those by XIA et al [13]. Microscopic structure of chalcopyrite massive electrode was analyzed by Leica DMRXE automatic image analyzer (German).
3 Results and discussion
3.1 Influence of pH on chalcopyrite bioleaching
The characteristics of chalcopyrite chemical leaching under different initial pH (1.0, 1.5 and 2.0) are shown in Fig. 1. It can be seen from Figs. 1(a), (c) and (e) that the copper ion concentration reached 2.12, 0.26 and 0.23 g/L after 10 d leaching under the initial pH of 1.0, 1.5 and 2.0, respectively. The decrease of the initial pH from 2.0 to 1.0 increased the copper ion concentration from 0.23 to 2.12 g/L. The results were in accordance with those by
et al [4]. Chalcopyrite dissolution rate increases with the decrease of the initial pH for the dissolution of chalcopyrite in chemical leaching, which could be mainly under proton attack as shown in the following reaction (1). Some researchers also suggested that there might be a parallel, non-oxidative dissolution process (reaction 2) [15]. The dissolution of chalcopyrite in chemical leaching could thus be under the combined action of oxidative and non-oxidative mechanisms.
CuFeS2+4H++O2
Cu2++2S0+Fe2++2H2O (1)
CuFeS2+4H+chemical
Cu2++Fe2++2H2S (2)
As shown in Fig. 1, pH values in chemical leaching were all kept increasing for the dissolution of chalcopyrite, indicating that it is a proton consumption processing. It can be seen from Fig. 1 that the redox potential values at pH 1.5 and 2.0 both increased at the initial stage of chemical leaching, and then decreased slowly. It is noteworthy that the initial redox potential at pH 1.0 was obvious higher than the other two cases for higher pH. The redox potential at initial pH 1.0 decreased rapidly in the first two days, which could be resulted from the rapid formation of ferrous ions during chalcopyrite dissolution in the first two days.
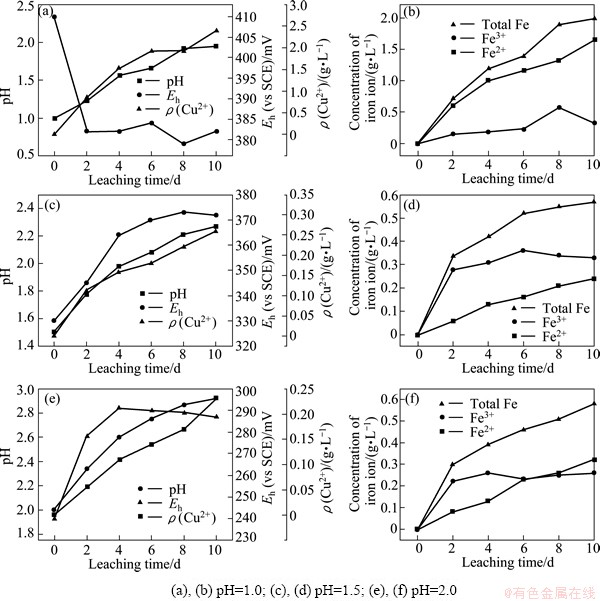
Fig. 1 Influence of initial pH on chalcopyrite chemical leaching at 65 °C
The bioleaching results under different initial pH are shown in Fig. 2. As shown in the Fig. 2, the presence of A. manzaensis effectively enhanced the dissolution of chalcopyrite under initial pH 1.5 and 2.0, and the copper ion concentrations increased from 0.23 and 0.26 g/L to 4.1 and 4.3 g/L, respectively. However, the presence of A. manzaensis just increased the copper ion concentration from 2.12 to 2.42 g/L when the initial pH was 1.0. Chalcopyrite bioleaching rate increased with the increase of the initial pH, and the optimum initial pH is 2.0. The presence of A. manzaensis almost did not promote chalcopyrite dissolution, indicating that chalcopyrite dissolution under initial pH 1.0 is mainly under the action of proton rather than the oxidation of A. manzaensis.
Figure 2 also shows that the lag phase of A. manzaensis in pH 1.0 was obvious longer than that in pH 1.5 or 2.0, and the maximum cell number 3×107 mL-1 was also lower compared with the other two cases. The results indicated that initial pH 1.0 has serious inhibition to the growth of A. manzaensis, which should be mainly responsible for the poor leaching effect [12]. The promotion of proton attack on chalcopyrite dissolution was counteracted by the inhibition on the growth of A. manzaensis. RUAN et al [16] studied heap bioleaching of secondary sulfide copper ores at low pH and high ferric concentration, and they found that the dissolution of sulfide copper ores was mainly under the chemical leaching rather than bioleaching when the pH was about 1.0.
To determine the intermediate products formed during the chalcopyrite (bio) leaching, (bio) leaching residues were analyzed by XRD, and the spectra are shown in Fig. 3. As shown in Fig. 3(a), some elemental sulfur was formed during chemical leaching of chalcopyrite under initial pH 1.0. This confirmed that the dissolution of chalcopyrite in chemical leaching was according to reaction (1).
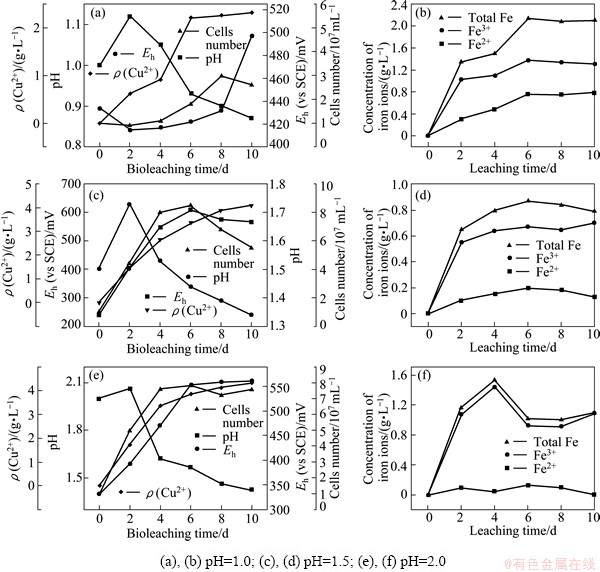
Fig. 2 Influence of initial pH on chalcopyrite bioleaching by Acidianus manzaensis at 65 °C
Besides elemental sulfur and chalcopyrite, jarosite was found in all the bioleaching residues, elemental sulfur and jarosite were also detected in our previous work [17]. It is noteworthy that the amount of jarosite in chalcopyrite bioleaching under initial pH 1.0 was far lower than that at the other two pH values, indicating high acidity inhibition to the formation of jarosite, which is in accordance with results of KLAUBER [18].
3.2 Influence of pH on anodic oxidation reactions of chalcopyrite massive electrode in the absence or presence of A. manzaensis
The effects of initial pH on the anodic oxidation reactions of chalcopyrite massive electrode in the absence or presence of A. manzaensis are shown in Figs. 4(a) and (b), respectively.
It is clearly that two intervals can be identified. The shape and current density of the anodic peak of A1 were almost not influenced by the change of pH when the applied potential was lower than 0.6 V, the current density of A2 peak increased with the increase of the pH when the applied potential was higher than 0.6 V (Fig. 4(a)). The similar phenomena were observed at other temperatures by some researchers [7,9]. The anodic oxidation current increased with the decrease of the acidity, indicating that the influence of pH on the chalcopyrite anodic oxidation reactions is contrary to that on chemical leaching.
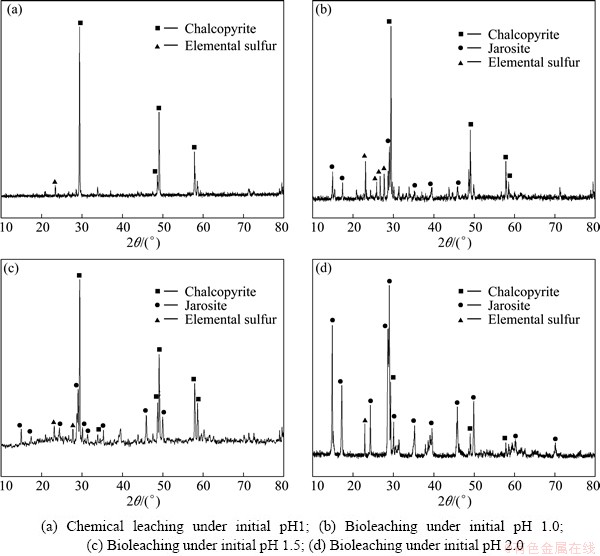
Fig. 3 XRD spectra of leached residues
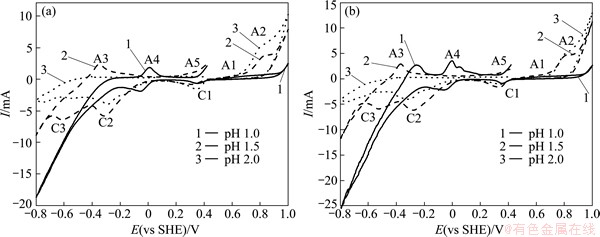
Fig. 4 Cyclic voltammograms of massive chalcopyrite electrodes at different initial pH values (1.0, 1.5 and 2.0) in sterilized 9K medium (a) and in the presence of Acidianus manzaensis (b)
Described as our previous work [14], A1 should represent chalcopyrite oxidation to elemental sulfur and covellite. A2 represents the massive oxidation dissolution of chalcopyrite and the decomposition of covellite to copper ions and sulfate. Meanwhile, the oxidation of elemental sulfur and ferrous ions to sulfate and ferric ions, respectively, also take place in A2. WARREN et al [19] reported that the anodic reactions currents increased with the decrease of the acidity. WARREN et al [19] and LI et al [9] considered that the oxidation reactions take place during chalcopyrite anodic oxidation according to reactions (3) and (4), both the two reactions produce proton. Therefore, the increase of the acidity inhibits the anodic oxidation reactions of chalcopyrite.
(3)
S0+2H2O+3O2→4H2++
(4)
CuFeS2+4H+
Cu2++Fe2++2H2S (5)
The contrary influence of pH on the chalcopyrite chemical leaching and anodic oxidation reactions indicated besides through reaction (1) chalcopyrite oxidation dissolution could be by other non-oxidative reactions in the chemical leaching. High acidity may be favorable to the non-oxidative dissolution, rather than oxidative dissolution of chalcopyrite. LAZARO and NICOL [15] suggested that a parallel, non-oxidative dissolution process (reaction 2) exists in the chalcopyrite chemical leaching in acidic solution. The comparison of chalcopyrite chemical leaching and electrochemical experiments confirmed chalcopyrite dissolution in chemical leaching is under the combined action of oxidation and non-oxidation according to Eqs. (1) and (2).
Comparison of Figs. 4(a) and (b) shows that the influence of pH on the anodic oxidations of chalcopyrite electrode is the same whether A. manzaensis presents or not. Meanwhile, the presence of A. manzaensis did not lead to changes in the shape and the potentials of the oxidation reactions of chalcopyrite. Similar phenomena were observed by LI and HUANG [20]. As shown in Figs. 4(a) and (b), the presence of A. manzaensis effectively promoted the oxidation reaction currents when the potential was higher than 0.6 V, but slightly influenced the oxidation reactions when the potential was lower than 0.6 V.
A1 represents the oxidation of chalcopyrite to covellite, therefore the presence of A. manzaensis cannot influence the oxidation current of A1. The promotion of A. manzaensis to the current of anodic peak A2 can be mainly due to its ability to oxidize elemental sulfur and ferrous ions to sulfuric acid and ferric ions, respectively. Anodic oxidation current of chalcopyrite electrode under three pH values exhibited the following order: IpH2.0>IpH1.5>IpH1.0, which is identical to chalcopyrite bioleaching rate order.
3.3 Characterization of anodic products of chalcopyrite electrode
It is clear that the anodic peak A1 previous to the massive dissolution of chalcopyrite represents the initial oxidation reactions of chalcopyrite, and it is also the pre-wave described by other authors [21,22]. A1 was suggested to lead to the passivation of chalcopyrite, but the oxidation reactions taking place are still disputed [21,23-25].
To characterize the products formed at A1, potentiostatic oxidation of the electrode at the potential 0.607 V for 4 min was performed. Surface images of the electrode after potentiostatic oxidation were observed by automatic image analyzer (Leica DMRXE) and the result is shown in Fig. 5. Products formed in A1 were analyzed by Raman spectroscopy and the spectrum is shown in Fig. 6.
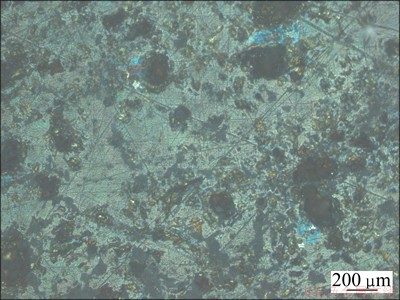
Fig. 5 Surface image of chalcopyrite electrode after potentiostatically oxidized at 0.607 V for 4 min
It can be seen from Fig. 5 that there were some blue products formed on the electrode surface. PARKER et al [26] found the formation of covellite when chalcopyrite electrode oxidation was at 0.644 V in the sulfuric acid system. ZENG [27] also found that there were some blue products formed on the surface of chalcopyrite electrode when it was potentiostatic oxidation at 0.4-0.5 V (vs Ag/AgCl) for 1 min. The blue products formed on the electrode surface should be covellite in accordance with the above results.
Figure 6 clearly shows the characteristic peak of chalcopyrite at 291.5 cm-1, the covellite peak at 473 cm-1 and the elemental sulfur absorption peaks at 153, 218, 438 and 469 cm-1. Five absorption peaks, 152.3, 219, 291.8, 465.2 and 473.1 cm-1, respectively, were observed by the Raman spectroscopy of chalcopyrite electrode potentiostatic oxidized at 0.607 V for 4 min, indicating clearly the formation of elemental sulfur (152.3, 219 and 465.2 cm-1), and covellite (473.1 cm-1) on the chalcopyrite electrode surface. These results confirmed that A1 represents the decomposition of chalcopyrite to covellite and elemental sulfur according to reaction (6) as follows:
CuFeS2→CuS+Fe2++S0+2e (6)
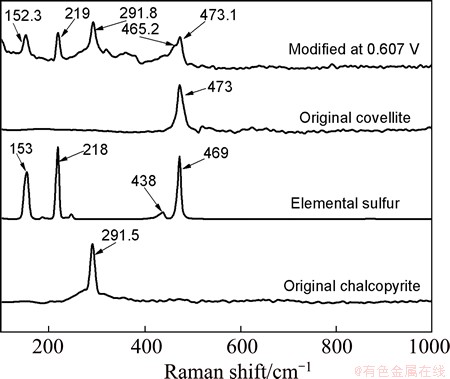
Fig. 6 Raman spectra of chalcopyrite electrode potentiostatic oxidized at 0.607 V for 4 min
4 Conclusions
Chalcopyrite dissolution rate increases with the decrease of initial pH in chemical leaching, and the decrease of initial pH from 2.0 to 1.0 significantly promotes chalcopyrite dissolution. However, chalcopyrite dissolution rate increases with the increase of the initial pH in bioleaching, and the presence of A. manzaensis significantly promotes chalcopyrite dissolution in bioleaching, except the case of the initial pH 1.0. High acidity in the case of initial pH 1.0 has serious inhibition to the growth of A. manzaensis. Independent of the presence of A. manzaensis, anodic oxidation currents increase with the increase of pH, and elemental sulfur and covellite are found on the electrode surface. Different effects of initial pH on chalcopyrite chemical leaching and electrochemical experiments confirm that chalcopyrite dissolution in chemical leaching is under the combined action of oxidation and non-oxidation of proton, with conversion of chalcopyrite to covellite and elemental sulfur.
References
[1] BRIERLEY J A. A perspective on developments in biohydrometallurgy [J]. Hydrometallurgy, 2008, 94(1-4): 2-7.
[2] MARHUAL N P, PRADHAN N, KAR R N, SUKLA L B, MISHRA B. Differential bioleaching of copper by mesophilic and moderately thermophilic acidophilic consortium enriched from same copper mine water sample [J]. Bioresource Technology, 2008, 99(17): 8331-8336.
[3] ROHWERDER T, GEHRKE T, KINZLER K, SAND W. Bioleaching review part A [J]. Applied Microbiology and Biotechnology, 2003, 63(3): 239-248.
[4]
J, YAMADA R, INOUE C. Effect of pH reduction and ferric ion addition on the leaching of chalcopyrite at thermophilic temperatures [J]. Hydrometallurgy, 2009, 96(1-2): 62-71.
[5]
E M, MUNOZ J A, BLAZQUEZ M L, GONZALEZ F, BALLEZTER A. Leaching of chalcopyrite with ferric ion. Part I: General aspects [J]. Hydrometallurgy, 2008, 93(3-4): 81-87.
[6]
G D. Investigation of the leaching of chalcopyrite ore in acidic solutions [J]. Hydrometallurgy, 2004, 73(3-4): 245-256.
[7] HIROYOSHI N, KITAGAWA H, TSUNEKAWA M. Effect of solution composition on the optimum redox potential for chalcopyrite leaching in sulfuric acid solutions [J]. Hydrometallurgy, 2008, 91(1-4): 144-149.
[8] WARREN G W, SOHN H J, WADSWORTH M E, WANG T G. The effect of electrolyte composition on the cathodic reduction of CuFeS2 [J]. Hydrometallurgy, 1985, 14(2): 133-149.
[9] LI Hong-xu, QIU Guan-zhou, HU Yue-hua, CANG Da-qiang, WANG Dian-zuo. Electrochemical behavior of chalcopyrite in presence of Thiobacillus ferrooxidans [J]. Transactions of Nonferrous Metals Society of China, 2006, 16(5): 1240-1245.
[10] ZHU Li, ZHANG De-cheng, LUO Xue-gang. Electrochemical behavior of chalcopyrite in sulfuric acid leaching [J]. Metal Mine, 2008, 38(5): 66-69. (in Chinese)
[11] HE Huan, XIA Jin-lan, YANG Yi, JIANG Hong-chen, XIAO Chun-qiao, ZHENG Lei, MA Chen-yan, ZHAO Yi-dong, QIU Guan-zhou. Sulfur speciation on the surface of chalcopyrite leached by Acidianus manzaensis [J]. Hydrometallurgy, 2009, 99(1-2): 45-50.
[12] DING Jian-nan, ZHANG Rui-yong, YU You-zun, JIN De-cai, LIANG Chang-li, YANG Yi, ZHU Wei, XIA Jin-lan. A novel acidophilic, thermophilic iron and sulfur-oxidizing archaeon isolated from a hot spring of tengchong, Yunnan, China [J]. Brazilian Journal of Microbiology, 2011, 42(2): 514-525.
[13] XIA Jin-lan, YANG Yi, HE Huan, ZHAO Xiao-juan, LIANG Chang-li, ZHENG Lei, MA Chen-yan, ZHAO Yi-dong, NIE Zhen-yuan, QIU Guan-zhou. Surface analysis of sulfur speciation on pyrite bioleached by extreme thermophile Acidianus manzaensis using Raman and XANES spectroscopy [J]. Hydrometallurgy, 2010, 100(3-4): 129-135.
[14] LIANG Chang-li, XIA Jin-lan, YANG Yi, NIE Zhen-yuan, ZHAO Xiao-juan, ZHENG Lei, MA Chen-yan, ZHAO Yi-dong. Characterization of the thermo-reduction process of chalcopyrite at 65 °C by cyclic voltammetry and XANES spectroscopy [J]. Hydrometallurgy, 2011, 107(1-2): 13-21.
[15] LAZARO I,NICOL M J. The mechanism of the dissolution and passivation of chalcopyrite: An electrochemical study [C]//VANCOUVER B C. 5th International Symposium Honoring Professor Ian M. Ritchie. America: Minerals, Metals & Materials Society, 2003: 405-417.
[16] RUAN Ren-man, LIU Xin-yu, ZOU Gang, CHEN Jing-he, WEN Jian-kang, WANG Dian-zuo. Industrial practice of a distinct bioleaching system operated at low pH, high ferric concentration, elevated temperature and low redox potential for secondary copper sulfide [J]. Hydrometallurgy, 2011, 108(1-2): 130-135.
[17] LIANG Chang-li, XIA Jin-lan, NIE Zhen-yuan, YANG Yi, MA Chen-yan. Effect of sodium chloride on sulfur speciation of chalcopyrite bioleached by the extreme thermophile Acidianus manzaensis [J]. Bioresource Technology, 2012, 110: 462-467.
[18] KLAUBER C. A critical review of the surface chemistry of acidic ferric sulphate dissolution of chalcopyrite with regards to hindered dissolution[J]. International Journal of Mineral Processing, 2008, 86(1-4): 1-17.
[19] WARREN G, WADSWORTH M, EL-RAGHY S. Passive and transpassive anodic behavior of chalcopyrite in acid solutions [J]. Journal of Electronic Materials, 1982,13(1): 571-579.
[20] LI A-lin, HUANG Song-tao. Comparison of the electrochemical mechanism of chalcopyrite dissolution in the absence or presence of Sulfolobus metallicus at 70 °C [J]. Mineral Engineering, 2011, 24(13): 1520-1522.
[21] BIEGLER T, HORNE M D. The electrochemistry of surface oxidation of chalcopyrite [J]. Journal of the Electrochemical Society, 1985, 132(6): 1363-1369.
[22] PRICE D W, WARREN G W. The influence of silver ion on the electrochemical response of chalcopyrite and other mineral sulfide electrodes in sulfuric acid [J]. Hydrometallurgy, 1986, 15(3): 303-304.
[23] ARCE E M, GONZALEZ I. A comparative study of electrochemical behavior of chalcopyrite, chalcocite and bornite in sulfuric acid solution [J]. International Journal of Mineral Processing, 2002, 67(1-4): 17-28.
[24] VUKOVIC M, STANKOVIC Z, RAJCIC-VUJASINOVIC M, CVETKOVSKI V. Voltammetric investigations of anodic dissolution of natural mineral chalcopyrite [J]. Journal of Mining and Metallurgy B: Metallurgy, 2008, 44(1): 115-124.
[25] LU Yi-ping, JIANG Xiao-hui, FENG Qi-ming, OU Le-ming, ZHANG Guo-fan. Electrochemical behavior of chalcopyrite at normal temperature in acidic solution [J]. Transactions of Nonferrous Metals Society of China, 2007, 17(3): 465-470.
[26] PARKER A J, PAUL R L, POWER G P. Electrochemical aspects of leaching copper from chalcopyrite in ferric and cupric salt solutions [J]. Australian Journal of Chemistry, 1981, 34(1): 13-34.
[27] ZENG Wei-min. The formation mechanism of passivation layer and its elimination way during bioleaching of chalcopyrite [D]. Changsha, China: Central South University, 2010: 78-82. (in Chinese).
初始pH对极端嗜热菌万座嗜酸两面菌作用下黄铜矿氧化溶解的影响
梁长利1,2,夏金兰2,3,聂珍媛2,3,余水静1,许宝泉1
1. 江西理工大学 资源与环境工程学院,赣州 341000;
2. 中南大学 资源加工与生物工程学院,长沙 410083;
3. 中南大学 教育部生物冶金重点实验室,长沙 410083
摘 要:采用生物浸出和循环伏安试验研究初始pH对黄铜矿在65 °C条件下氧化溶解的影响,分别采用XRD和拉曼光谱分析黄铜矿生物浸出过程中和电极表面形成的氧化产物。化学浸出结果表明:黄铜矿溶解速率随着初始pH的减小而增大,然而初始pH对黄铜矿生物浸出的影响却正好相反。当初始pH为1.0时,万座嗜酸两面菌的加入几乎没有促进黄铜矿的溶解,这主要是由于高酸度严重抑制了万座嗜酸两面菌的生长。电解液中存在或不存在万座嗜酸两面菌时黄铜矿的阳极氧化电流都随着初始pH的增大而增大,同时在黄铜矿电极表面检测到了单质硫和铜蓝。结果表明,在化学浸出过程中黄铜矿是在质子作用和氧化作用下溶解的,黄铜矿氧化生成了铜蓝和单质硫。
关键词:黄铜矿;生物浸出;万座嗜酸两面菌;初始pH;氧化溶解
(Edited by Xiang-qun LI)
Foundation item: Projects (50974140, 51274257) supported by the National Natural Science Foundation of China; Project (JXXJBS11003) supported by the Doctor Initiating Foundation of Jiangxi University of Science and Technology, China
Corresponding author: Jin-lan XIA; Tel: +86-731-88836944; E-mail: jlxia@csu.edu.cn
DOI: 10.1016/S1003-6326(14)63268-4