
ARTICLE
J. Cent. South Univ. (2019) 26: 1435-1442
DOI: https://doi.org/10.1007/s11771-019-4099-2

Improving electrochemical properties of carbon paper as negative electrode for vanadium redox battery by anodic oxidation
HOU Bing-xue(侯冰雪)1, 2, CUI Xu-mei(崔旭梅)2, ZHANG Qi(张祺)3, CHEN Yun-gui(陈云贵)1
1. Department of Advanced Energy Materials, College of Materials Science and Engineering,Sichuan University, Chengdu 610065, China;
2. College of Vanadium and Titanium, Panzhihua University, Panzhihua 617000, China;
3. School of Intelligent Manufacturing , Panzhihua University, Panzhihua 617000, China
Central South University Press and Springer-Verlag GmbH Germany, part of Springer Nature 2019
Abstract: Anodic oxidation with different electrolyte was employed to improve the electrochemical properties of carbon paper as negative electrode for vanadium redox battery (VRB). The treated carbon paper exhibits enhanced electrochemical activity for V2+/V3+ redox reaction. The sample (CP-NH3) treated in NH3 solution demonstrates superior performance in comparison with the sample (CP-NaOH) treated in NaOH solution. X-ray photoelectron spectroscopy results show that oxygen- and nitrogen-containing functional groups have been introduced on CP-NH3 surface by the treatment, and Raman spectra confirm the increased surface defect of CP-NH3. Energy storage performance of cell was evaluated by charge/discharge measurement by using CP-NH3. Usage of CP-NH3 can greatly improve the cell performance with energy efficiency increase of 4.8% at 60 mA/cm2. The excellent performance of CP-NH3 mainly results from introduction of functional groups as active sites and improved wetting properties. This work reveals that anodic oxidation is a clean, simple, and efficient method for boosting the performance of carbon paper as negative electrode for VRB.
Key words: vanadium redox battery; carbon paper; negative electrode; anodic oxidation; electrochemical kinetics
Cite this article as: HOU Bing-xue, CUI Xu-mei, ZHANG Qi, CHEN Yun-gui. Improving electrochemical properties of carbon paper as negative electrode for vanadium redox battery by anodic oxidation [J]. Journal of Central South University, 2019, 26(6): 1435-1442. DOI: https://doi.org/10.1007/s11771-019-4099-2.
1 Introduction
Benefiting from massive utilization of new energy (solar, wind, etc.), energy storage technology has been extensively developed to resolve low stability of electrical energy resulting from the intermittent feature of new energy [1]. Vanadium redox battery (VRB), initially proposed by SKYLLAS-KAZACOS et al in 1980s, has been acknowledged as a promising energy storage candidate thanks to many advantages of high efficiency, long cycling life and large scale [2, 3]. Recently, VRB has been paid much attention and reached commercial fruition. Distinguishing from other secondary batteries, vanadium ions with various valences stored in electrolyte are employed as active species in positive and negative half-cells, which prevents cross-contamination issue. Electrolyte circulation system favors to design capacity and power, separately [4]. Great potential of VRB in large-scale energy storage integrated with photovoltaic and turbine has been demonstrated in many applications from kW/(kW·h) to MW/(MW·h) [5].
VRB realizes charge/discharge process by valence transfer of V2+/V3+ and VO2+/VO2+ couples in negative and positive sides, respectively. The standard voltage of 1.26 V is produced from potential separation of two couples [6]. Two reactions take place on electrode surface, and the electrode does not join the reaction like the electrode of lithium ion battery. Hence, electrochemical activity of electrode is crucial for VRB performance. Commonly, VRB mainly employs carbon-based fiber as electrode, such as graphite felt, and carbon paper. Nevertheless, these raw electrodes demonstrate low electrochemical and wetting performances. Thus, many methods such as acid treatment and heating treatment have been developed to raise the electrode performance. Moreover, developing new catalysts is a research hotspot for VRB. Developed catalysts mainly consist of three kinds of materials including carbon-based materials (graphene [7], CNTs [8], etc.), metals (Bi [9], Cu [10], etc.), and metal oxides (ZrO2 [11], Ta2O5 [12], etc.).
Electrochemical modification is a clean, simple, and efficient method, and has been used in many energy storage fields. LI et al [13] treated graphite felt electrode by electrochemical oxidation treatment in sulfuric acid solution. The treated electrode exhibited improved electrochemical activity, leading to the increase of energy storage efficiency of VRB. HE et al [14] modified graphite felt for VRB by square wave potential pulse. This treatment introduced oxygen- containing groups and nano-scale pores on graphite felt surface. Recently, the electrochemical kinetics of VO2+/VO2+ reaction has been improved greatly by many routes including modifying electrode and optimizing electrolyte. Moreover, V2+/V3+ reaction takes larger proportion for cell voltage loss. Thus, improving the performance of negative electrode is very important for comprehensive performance of VRB. In this work, anodic oxidation was employed to modify carbon paper as negative electrode with different electrolyte. The modification using NH3 solution etched carbon paper and introduced oxygen- and nitrogen-containing groups. The electrochemical performances of modified carbon paper were investigated systematically.
2 Experimental
2.1 Preparation of materials
In this experiment, all reagents were of analytical grade. Carbon paper (HCP030N) was provided by Hesen electric Co. Ltd., Shanghai. Carbon paper was modified by constant-current anodic oxidation at ambient temperature. Two-electrode system was employed, which consisted of carbon paper as anode and Pt sheet as cathode (1 cm×2 cm). Anodic oxidation process was carried out at a current density of 20 mA/cm2 for 30 min in 1 mol/L NaOH and 2 mol/L NH3 solutions, respectively. The treated carbon paper was washed with deionized water until water became neutral, then followed by drying at 80 °C for 8 h. The corresponding carbon papers using NaOH and NH3 solutions were denoted for CP-NaOH and CP-NH3, respectively.
2.2 Characterizations of materials
Scanning electron microscope (SEM, S-4800, Hitachi) was employed to survey morphology of samples. X-ray photoelectron spectroscope (XPS, Kα 1063, Thermo Fisher Scientific) was conducted to study surface composition of samples. Surface defect degree of carbon paper was evaluated by Raman spectroscope (DXR, Thermo Fisher Scientific).
2.3 Electrochemical measurements
Electrochemical measurements were performed on CHI660E electrochemical workstation in 1.0 mol/L V3+ + 3.0 mol/L H2SO4 electrolyte. Three-electrode system composed of working electrode (carbon paper), counter electrode (Pt sheet), and reference electrode (saturated calomel electrode, SCE) was employed in measurements. The working area of carbon paper was controlled at 1 cm2. Cyclic voltammetry was conducted at fixed scanning rate between -0.1 and -0.8 V. Electrochemical impedance spectroscopy was performed in range of 10-1-105 Hz with polarization potential of -0.45 V.
2.4 VRB performance
VRB performance was assessed by charge/discharge measurement with a static cell. The cell was mainly composed of electrode, proton-exchange membrane, and conductive plastic. Carbon paper and graphite felt were employed as negative and positive electrodes, respectively. Electrode size was controlled at 4 cm×4 cm. Galvanostatic charge/discharge measurement was performed on CT2001A testing system (Land, Wuhan) in 0.7-1.7 V. 1.6 mol/L VO2++3 mol/L H2SO4 and 1.6 mol/L V3++3 mol/L H2SO4 were employed as original negative and positive electrolytes, respectively. The electrolyte was stored in corresponding electrode. The charge/discharge performance was evaluated at 60 mA/cm2 for 50 cycles. Before normal measurements, the cell was measured for 2 cycles at 10 mA/cm2.
3 Results and discussions
Representative SEM images of three samples are shown in Figure 1. All samples exhibit obvious fiber shape with diameter of about 8 μm. CP has relatively smooth surface, while CP-NaOH and CP-NH3 demonstrate rough surface. This indicates that anodic oxidation can etch the carbon paper surface. It is noted that CP-NaOH and CP-NH3 have the similar etching degree, implying that the electrolyte species for anodic oxidation has no obvious impact on surface morphology of carbon paper.
XPS measurements were employed to investigate the surface composition of electrode. XPS survey spectra of three samples are presented in Figure 2. As for pristine CP, there are a large peak for C 1s at 285 eV and a little peak for O 1s at 532 eV [15]. The existence of O with little content maybe is ascribed to the adsorbed oxygen. Compared with CP, the peak for O 1s at 532 eV becomes stronger for both CP-NaOH and CP-NH3. Moreover, a new large peak at about 400 eV appears for CP-NH3, which is attributed to N 1s. The relevant composition of three samples is listed in Table 1. O content increases to 8.53% for CP-NaOH from 1.27% for CP, indicating that oxygen-containing groups are introduced on carbon paper surface by anodic oxidation in NaOH solution. As for CP-NH3, O and N content increases to 6.06% and 9.52%, respectively, which confirms the introduction of oxygen- and nitrogen-containing groups by anodic oxidation in NH3 solution. It is noted that the O content for CP-NH3 is slightly lower than that for CP-NaOH. This probably results from the competition of NH4+ ions to OH- ions in NH3 solution. XPS measurements show that functional groups can be introduced on carbon paper surface by anodic oxidation treatment, and the electrolyte can greatly affect the composition of introduced groups.
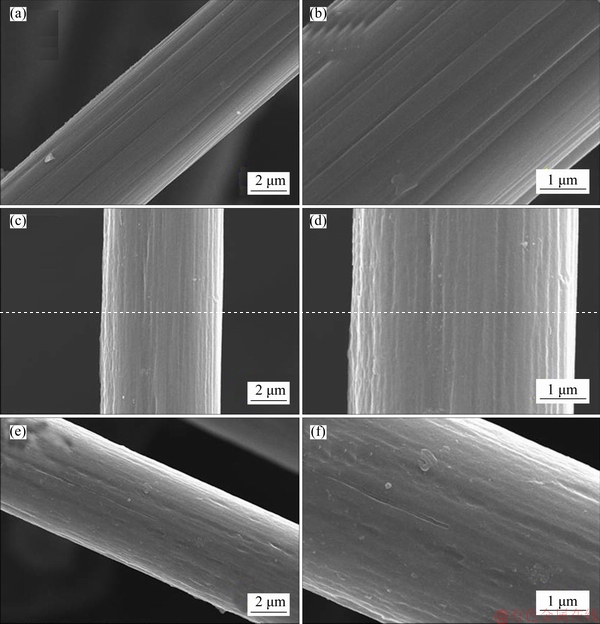
Figure 1 Representative SEM images of CP (a, b), CP-NaOH (c, d) and CP-NH3 (e, f) samples at different magnifications
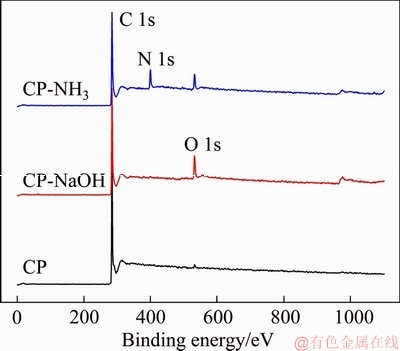
Figure 2 XPS survey spectra of carbon paper samples in range of 1-1100 eV
Table 1 Composition of major elements on carbon paper surface obtained from XPS measurements (molar fraction, %)
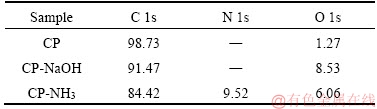
Raman spectroscopy was employed to study surface carbon structure of samples, and the results are displayed in Figure 3. Two strong peaks at 1360 and 1600 cm-1 are ascribed to disordered amorphous carbon (D band) and crystalline graphitic carbon (G band), separately [16]. Intensity ratio of D band to G band (ID/IG) can be used to evaluate surface defect [17]. Larger ID/IG value for CP-NaOH (1.03) and CP-NH3 (1.05) implies more disordered characteristic compared with that of pristine CP (0.97), which implies increased defects on electrode surface owing to the introduction of functional groups. The largest ID/IG value for CP-NH3 probably comes from the oxygen- and nitrogen-containing groups. This has been verified by XPS results. It is well-known that increased surface defects can enhance the electrocatalytic performance of carbon-based electrode for VRB [18].
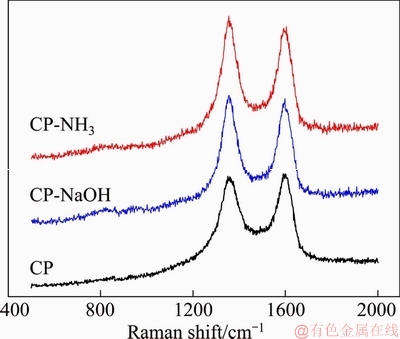
Figure 3 Raman spectra of CP, CP-NaOH and CP-NH3
The electrocatalytic performance of three carbon paper samples was compared by cyclic voltammetry measurements. The cyclic voltammograms of three samples are presented in Figure 4. As can be seen, there is no obvious peak shape for pristine CP. However, both CP-NaOH and CP-NH3 show obvious peak shape, with increased peak current and distinct peak potential gap. This indicates that anodic oxidation treatment can enhance the electrocatalytic performance of carbon paper. Clearly, CP-NH3 exhibits superior performance compared with CP-NaOH. Moreover, peak potential gap for CP-NH3 is 284 mV, 22 mV smaller than that for CP-NaOH (306 mV), implying that electrochemical reversibility of V2+/V3+ reaction on CP-NH3 is better than that on CP-NaOH. Superior electrocatalytic performance of CP-NH3 is probably related to two reasons. The first is that introduced functional groups can be employed as active sites for V2+/V3+ reaction, which has been verified by XPS measurements. The second is that these introduced groups can improve the hydrophilicity of carbon paper, further accelerating the mass transfer of vanadium ions.
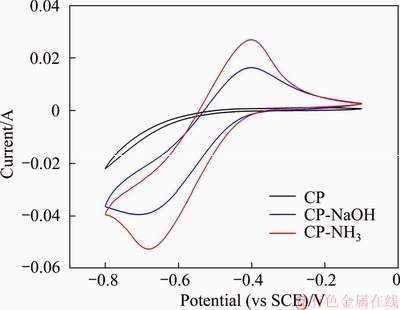
Figure 4 Cyclic voltammograms of three carbon paper samples obtained at scanning rate of 4 mV/s
Cyclic voltammograms of three electrodes at a series of scanning rate for V2+/V3+ redox reaction are shown in Figures 5(a)-(c). As observed, with the increase of scanning rate, the peak potential gap becomes larger, meaning that the electrochemical polarization becomes severer at large scanning rate. Compared with CP and CP-NaOH, the peak shape of CP-NH3 maintains well at large scanning rate, implying good electrochemical stability of CP-NH3. Moreover, mass transfer performance of vanadium ions can be assessed by variations of peak current combining with square root of scanning rate. The relevant plots for three electrodes are shown in Figure 5(d). The plots exhibit good linear relationship, indicating that V2+/V3+ reaction is controlled by the diffusion process. Slope of plots can reflect the mass transfer properties of electrode by Randles-Sevick equation [19, 20]. The large slope means better mass transfer performance. Clearly, two modified carbon paper electrodes show larger slope compared with pristine CP, implying the improved mass transfer performance of vanadium ions on both modified electrodes. CP-NH3 demonstrates the best performance among three electrodes. It is ascribed to introduce oxygen- and nitrogen-containing functional groups with good hydrophilicity, and CP-NH3 has the largest total content of these hydrophilic groups.
Electrochemical impedance spectroscopy was employed to further examine electrochemical kinetics of V2+/V3+ reaction. Nyquist plots of three samples and the corresponding equivalent electrical circuit are presented in Figure 6. In equivalent electrical circuit, Rct is charge transfer resistance, Rs is ohmic resistance of system, Qt and Qm are constant-phase elements corresponding to diffusion capacitance of vanadium ions and electric double- layer capacitance between electrode/electrolyte surface, separately. Nyquist plots mainly consist of semi-circle at high frequency corresponding to charge transfer resistance and oblique line at low frequency corresponding to transfer process of active species [21, 22]. It implies that V2+/V3+ reaction is conjointly dominated by charge transfer process and diffusion process of active species. Rct for both CP-NaOH (1.3 Ω) and CP-NH3 (0.3 Ω) decreases greatly compared with pristine CP (13.6 Ω), demonstrating quicker charge transfer for redox reaction on modified carbon paper. Moreover, CP-NH3 exhibits the smallest Rct among modified samples, which is related to the introduction of oxygen- and nitrogen-containing groups by anodic oxidation with NH3 solution. The aforementioned results show that CP-NH3 with functional groups as active sites can accelerate electrochemical kinetics of reaction greatly.
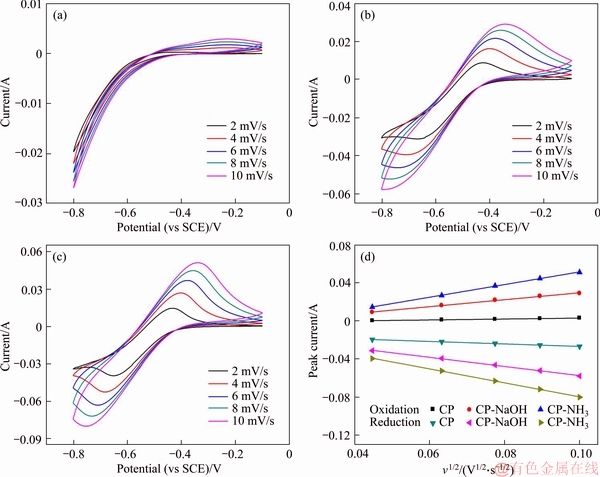
Figure 5 Cyclic voltammograms of CP (a), CP-NaOH (b), and CP-NH3 (c) at a series of scanning rate of 2-10 mV/s in 1.0 mol/L V3+ electrolyte, and relevant plots of peak current versus square root of scanning rate (d)
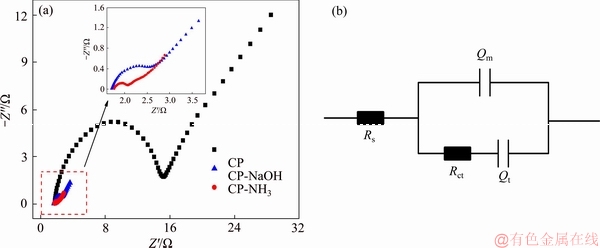
Figure 6 Nyquist plots of three carbon paper samples (a) and corresponding equivalent electrical circuit (b)
Typical charge-discharge measurements using static cell were carried out to evaluate the practical application of modified carbon paper. As shown in Figure 7(a), CP-NH3 based cell exhibits larger discharge capacity compared with pristine cell. Initial discharge capacity for CP-NH3 is 234.8 mA·h, 42.6 mA·h higher than that for pristine cell. Moreover, capacity retention for CP-NH3 maintains 86.0% after 50 cycles, higher than that for pristine cell (81.1%). The efficiency (current, voltage, and energy efficiency) of two cells is compared in Figures 7(b)-(d). The current efficiency for pristine cell is slightly higher than that for CP-NH3. It is maybe related to severe self-discharge and parasitic side reaction including hydrogen and oxygen evolution for CP-NH3 based cell in longer charge-discharge process. However, voltage efficiency reflecting cell electrochemical polarization for CP-NH3 is greatly higher than that for pristine cell, which results from enhancement in electrochemical and mass transfer processes [23]. Therefore, comprehensive energy efficiency, conjointly determined by current and voltage efficiency, increases by 4.8% by using CP-NH3 as negative electrode (79.5%). Charge-discharge curves for two cells after different cycles are shown in Figures 7(e) and (f). As seen, the cell for CP-NH3 occupies more charge-discharge time. Compared with pristine cell, the cell using CP-NH3 shows lower charge voltage and higher discharge voltage, indicating lower electrochemical polarization of the cell using CP-NH3. Charge-discharge measurements reveal that using CP-NH3 as negative electrode can raise energy storage performance of VRB.
4 Conclusions
Surface-etched carbon paper with functional groups was prepared by anodic oxidation. Oxygen- and ntirogen-containing groups can be introduced on the surface of carbon paper by using NH3 soultion as electrolyte. CP-NH3 exhibits superior electrocatalytic performance for V2+/V3+ reaction in comparison with CP and CP-NaOH. The performance improvement of CP-NH3 mainly is ascribed to introduction of functional groups as active sites and improved wetting performance, further enhancing the electrochemical reaction and mass transfer. Therefore, CP-NH3 as negative electrode can improve cell performance including increased discharge capacity and energy efficiency. CP-NH3 treated by a clean and efficient anodic oxidation holds promising application potential in large-scale energy storage system.
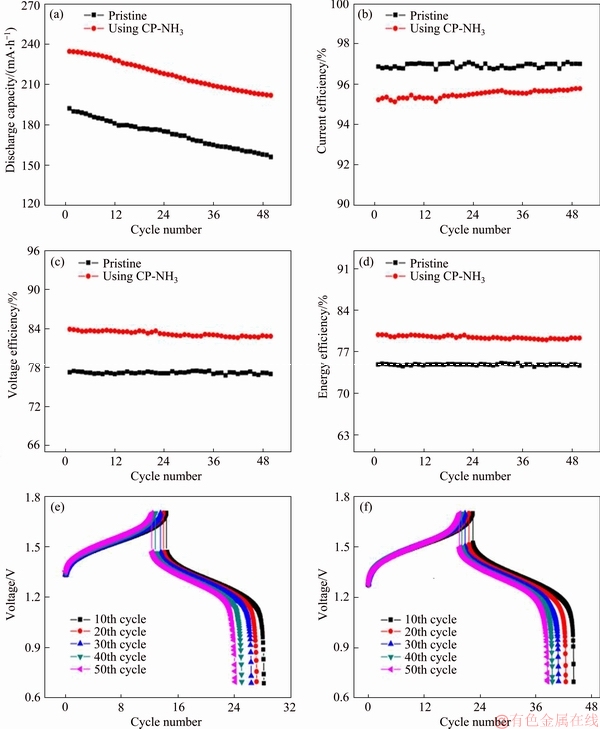
Figure 7 Discharge capacity (a), current efficiency (b), voltage efficiency (c) and energy efficiency (d) of two cells without and with CP-NH3 as negative electrode at 60 mA/cm2, charge-discharge curves without (e) and with (f) CP-NH3 after different cycles
References
[1] LI C, XIE B, CHEN J, HE Z, CHEN Z, LONG Y. Emerging mineral-coupled composite phase change materials for thermal energy storage [J]. Energy Conversion and Management, 2019, 183: 633-644.
[2] MEHBOOB S, ALI G, SHIN H J, HWANG J, ABBAS S, CHUNG K Y, HA H Y. Enhancing the performance of all-vanadium redox flow batteries by decorating carbon felt electrodes with SnO2 nanoparticles [J]. Applied Energy, 2018, 229: 910-921.
[3] HE Z, LI M, LI Y, LI C, YI Z, ZHU J, DAI L, MENG W, ZHOU H, WANG L. ZrO2 nanoparticle embedded carbon nanofibers by electrospinning technique as advanced negative electrode materials for vanadium redox flow battery [J]. Electrochimica Acta, 2019, 309: 166-176.
[4] YAN Y, SKYLLAS-KAZACOS M, BAO J. Effects of battery design, environmental temperature and electrolyte flowrate on thermal behaviour of a vanadium redox flow battery in different applications [J]. Journal of Energy Storage, 2017, 11: 104-118.
[5] ARENAS L F, PONCE D E LE N C, WALSH F C. Engineering aspects of the design, construction and performance of modular redox flow batteries for energy storage [J]. Journal of Energy Storage, 2017, 11: 119-153.
[6] LI L Y, KIM S, WANG W, VIJAYAKUMAR M, NIE Z M, CHEN B W, ZHANG J L, XIA G G, HU J Z, GRAFF G, LIU J, YANG Z G. A stable vanadium redox-flow battery with high energy density for large-scale energy storage [J]. Advanced Energy Materials, 2011, 1: 394-400.
[7] PARK M, JEON I Y, RYU J, JANG H, BACK J B, CHO J. Edge-halogenated graphene nanoplatelets with F, Cl, or Br as electrocatalysts for all-vanadium redox flow batteries [J]. Nano Energy, 2016, 26: 233-240.
[8] NOH C, MOON S, CHUNG Y, KWON Y. Chelating functional group attached to carbon nanotubes prepared for performance enhancement of vanadium redox flow battery [J]. Journal of Materials Chemistry A, 2017, 5: 21334- 21342.
[9] GONZALEZ Z, SANCHEZ A, BLANCO C, GRANDA M, MENENDEZ R, SANTAMARIA R. Enhanced performance of a Bi-modified graphite felt as the positive electrode of a vanadium redox flow battery [J]. Electrochemistry Communications, 2011, 13: 1379-1382.
[10] WEI L, ZHAO T S, ZENG L, ZHOU X L, ZENG Y K.. Copper nanoparticle- deposited graphite felt electrodes for all vanadium redox flow batteries [J]. Applied Energy, 2016, 180: 386-391.
[11] ZHOU H, SHEN Y, XI J, QIU X, CHEN L. ZrO2-nanoparticle-modified graphite felt: Bifunctional effects on vanadium flow batteries [J]. ACS Applied Materials & Interfaces, 2016, 8: 15369-15378.
[12] BAYEH A W, KABTAMU D M, CHANG Y C, CHEN G C, CHEN H Y, LIN G Y, LIU T R, WONDIMU T H, WANG K C, WANG C H. Ta2O5-nanoparticle-modified graphite felt as a high-performance electrode for a vanadium redox flow battery [J]. ACS Sustainable Chemistry & Engineering, 2018, 6: 3019-3028.
[13] LI X G, HUANG K L, LIU S Q, TAN N, CHEN L Q. Characteristics of graphite felt electrode electrochemically oxidized for vanadium redox battery application [J]. Transactions of Nonferrous Metals Society of China, 2007, 17: 195-199.
[14] HE Z, JIANG Y, ZHOU H, CHENG G, MENG W, WANG L, DAI L. Graphite felt electrode modified by square wave potential pulse for vanadium redox flow battery [J]. International Journal of Energy Research, 2016, 41: 439-447.
[15] LV YR, ZHANG L, CHENG G, WANG P F, ZHANG T Z, LI C C, JIANG Y Q, HE Z X, DAI L, WANG L. Preparation of carbon nanosheet by molten salt route and its application in catalyzing VO2+/VO2+ redox reaction [J]. Journal of the Electrochemical Society, 2019, 166: A953-A959.
[16] SUN D, TANG Y, HE K, REN Y, LIU S, WANG H. Long-lived aqueous rechargeable lithium batteries using mesoporous LiTi2(PO4)3@C anode [J]. Scientific Reports, 2015, 5: 17452-17459.
[17] SUN D, XUE X, TANG Y, JING Y, HUANG B, REN Y, YAO Y, WANG H, CAO G. High-rate LiTi2(PO4)3@N-C composite via bi-nitrogen sources doping [J]. ACS Applied Materials & Interfaces, 2015, 7: 28337-28345.
[18] PARK M, RYU J, KIM Y, CHO J. Corn protein-derived nitrogen-doped carbon materials with oxygen-rich functional groups: A highly efficient electrocatalyst for all-vanadium redox flow batteries [J]. Energy & Environmental Science, 2014, 7: 3727-3735.
[19] PARK M, JUNG YJ, KIM J, LEE H I, CHO J. Synergistic effect of carbon nanofiber/nanotube composite catalyst on carbon felt electrode for high-performance all-vanadium redox flow battery [J]. Nano Letters, 2013, 13: 4833-4839.
[20] LV Y, ZHANG J, LV Z Q, WU C X, LIU Y Y, WANG H N, LU S F, XIANG Y. Enhanced electrochemical activity of carbon felt for V2+/V3+ redox reaction via combining KOH-etched pretreatment with uniform deposition of Bi nanoparticles [J]. Electrochimica Acta, 2017, 253: 78-84.
[21] ZHANG W, XI J, LI Z, ZHOU H, LIU L, WU Z, QIU X. Electrochemical activation of graphite felt electrode for VO2+/VO2+ redox couple application [J]. Electrochimica Acta, 2013, 89: 429-435.
[22] WU L, SHEN Y, YU L, XI J, QIU X. Boosting vanadium flow battery performance by Nitrogen-doped carbon nanospheres electrocatalyst [J]. Nano Energy, 2016, 28: 19-28.
[23] HUANG Y, DENG Q, WU X, WANG S. N, O Co-doped carbon felt for high-performance all-vanadium redox flow battery [J]. International Journal of Hydrogen Energy, 2017, 42: 7177-7185.
(Edited by FANG Jing-hua)
中文导读
阳极氧化提升钒液流电池负极碳纸电极的电化学性能
摘要:本文利用阳极氧化来提升钒液流电池负极碳纸电极电化学性能。改性电极对V2+/V3+反应展现了优异的电化学活性。相比NaOH溶液处理的CP-NaOH样品,NH3溶液处理的CP-NH3样品展现了更好的性能。XPS结果显示含氧和含氮官能团被引入到CP-NH3表面,拉曼光谱确认了CP-NH3表面增加的缺陷。通过充放电实验来评估CP-NH3对钒电池储能性能的影响,使用CP-NH3可大幅度提升电池性能,在60 mA/cm2时能量效率提升4.8%。CP-NH3优异的性能主要来自于官能团的引入和提升的润湿性能,本文工作说明阳极氧化是一种清洁、简单、高效的提升钒液流电池负极碳纸电极性能的方法。
关键词:钒液流电池;碳纸;负极电极;阳极氧化;电化学动力学
Foundation item: Project(NCET-10-0946) supported by Program for New Century Excellent Talents in University of China; Project(2017JY0038) supported by Science and Technology Key Project of Sichuan Province, China; Project(2013TX8 ) supported by Titanium and Titanium Alloy Innovation Team of Panzhihua City, China
Received date: 2018-10-31; Accepted date: 2019-02-26
Corresponding authors: CUI Xu-mei, PhD, Professor; Tel: +86-812-3371996; E-mail: cuixumei@163.com; ORCID: 0000-0001-8464- 7773; CHEN Yun-gu, PhD, Professor; Tel: +86-28-85407335, E-mail: ygchen60@aliyun.com; ORCID: 0000-0001-8403-7289