
Strength distribution at interface of rotary-friction-welded aluminum to nodular cast iron
SONG Yu-lai(宋雨来), LIU Yao-hui(刘耀辉), ZHU Xian-yong(朱先勇),
YU Si-rong(于思荣), ZHANG Ying-bo(张英波)
Key Laboratory of Automobile Materials, Ministry of Education, College of Materials Science and Engineering,
Jilin University, Changchun 130025, China
Received 23 January 2007; accepted 25 July 2007
Abstract: The morphology, size and composition of intermetallic compound at the interface of Al 1050 and nodular cast iron were studied by electron microprobe analysis(EMPA) and scan electron microscopy (SEM), respectively. The bond strength of the interface was measured by the tensile tests and the morphology of the fracture surface was observed by SEM. The observation of the interface reveals that there are two distinct morphologies: no intermetallic compound exists in the central area at the interface; while numbers of intermetallic compounds (FexAly) are formed in the peripheral area due to the overfull heat input. The tensile tests indicate that the distribution of strength in radial direction at the interface is inhomogeneous, and the central area of the interface performs greater bond strength than the peripheral area, which proves directly that the FexAly intermetallic compounds have a negative effect on the integration of interface. The morphology on the fracture surface shows that the facture in the central area at the interface has characteristic of the ductile micro-void facture. So it is important to restrain the form of the intermetallic compound to increase the bond strength of the Al 1050 and nodular cast iron by optimizing welding parameters and the geometry of components.
Key words: aluminum; nodular cast iron; rotary friction welding; microstructure; strength distribution
1 Introduction
Joining of dissimilar materials is generally more challenging than that of similar metals because of differences in the physical, chemical and metallurgical properties of the parent metals to be joined[1-2]. Many highly efficient solid-state joining processes such as explosion, friction, diffusion, and cold roll welding are being developed to join dissimilar materials. Among these welding techniques, rotary friction welding(RFW) technique is a distinct, favorite method to join dissimilar metal substrates due to its characteristics of high reproducibility, sub-melting temperature, short weld time, low energy input and negligible intermetallic formation[3-4]. At present many dissimilar materials have been bonded by the RFW, such as aluminum[2,5], copper[6,7], titanium[8] and steel[9].
Pure aluminum to nodular cast iron welded joints can be readily produced by RFW, and such joints are extensively used in the various types of components for electrical machinery to obtain lightmass products and reduce manufacturing costs. Studies of the pure aluminum to nodular cast iron welded joint combinations, however, have not yet been well documented. It is well known that the bond strength at the interface is an important criterion to evaluate the mechanical properties of the RFW materials. A large number of references on the RFW of dissimilar metals have indicated that the relations between the bond strength and the microstructure at the interface have been established extensively[10-12]. However, the investigation on the strength distribution in radial direction at the interface has not been found yet. In the RFW process, the heat inputs and the temperature in the radial direction are inevitably different because of the different linear velocity under the same rotate speed. As a consequence, the strength in the radial direction maybe exhibits obvious distinction, which influences intensively the integration strength on the interface. So the investigation on the strength distribution in the radial direction is of importance for optimizing welding parameters and geometry of components and ultimately improving the integration strength. Until now no detailed measurements of the distribution of strength in the radial direction at interface have been made.
In the present work, Al 1050 and nodular cast iron were successfully joined by the RFW process. The microstructures at the interface and the facture surface were investigated by electron microprobe analysis (EMPA) and scan electron microscopy(SEM), respectively. The distribution of the bond strength in radial direction at interface was measured by a series of tensile tests, and the fracture behavior was also analyzed. It is expected that the preliminary results can be significant in promoting the properties of the rotary- friction-welded Al 1050/nodular cast iron composite.
2 Experimental
2.1 Rotary friction welding
The commercial Al 1050 (pure aluminum containing 99.823% of Al, mass fraction) and nodular cast iron, which were machined into rods with a diameter of 20 mm, were used for rotary friction welding. The working surfaces of both materials were polished successively with fine grades of emery papers up to 1 000 level before welding. All welds were produced using a continuous-driving machine with the constant parameters, which were optimized by static tensile strength in earlier studies. The interesting parameters were as follows: the friction pressure(p1) was maintained at 40 MPa with the friction time(t1) fixed at 1.0 s. The rotational speed(v) was held at 3 000 r/min. The forging pressure and forging time were 100 MPa and 3.0 s, respectively.
2.2 Microstructure of interface
Cross-sectional interfaces of the RFW were ground and polished using an emery paper and diamond powder. Microstructure and elements distribution at the cross-sectional interfaces were investigated by EMPA and SEM, respectively.
2.3 Static tensile testing
In order to measure the distribution of strength in radial direction on the interface, the specimens were machined into a series of tensile rods with different diameters as schematically shown in Fig.1. The tensile tests were conducted at room temperature using an Instron type testing machine with cross head speed of 2.5×10-2 mm/s. The morphologies on the fracture surfaces were observed by SEM.
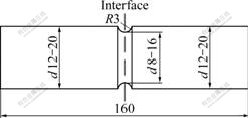
Fig.1 Schematic illustration of specimens for tensile tests
3 Results and discussion
3.1 Microstructure of interface
The interfaces are separated into five zones in the radial direction as shown in Fig.2. The preliminary observation shows that the microstructures in zone 2 and zone 4 exhibit representative features. Fig.3 and Fig.4 show the cross section microstructures of zone 2 and zone 4, respectively. It can be seen that the interface is smooth and relatively straight, and the reaction layer can not be easily discerned in zone 2, but the jagged interface is apparently observed in zone 4. The results of EMPA shown in Figs.5 and 6 indicate that no intermetallic compound forms in zone 2, while FexAly intermetallic compounds form with a maximum thickness of around 3 μm in zone 4. The different microstructures between zone 2 and zone 4 are exclusively affected by heat input resulted from the different linear velocities on friction surface under the same processing condition.
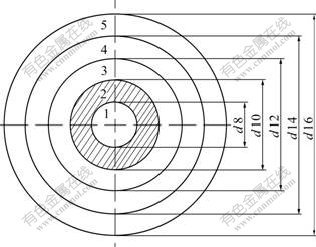
Fig.2 Partition of zones in radial direction at interface
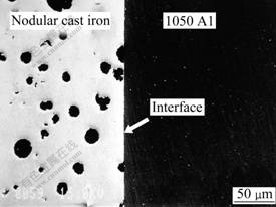
Fig.3 Cross section microstructure in zone 2 at interface
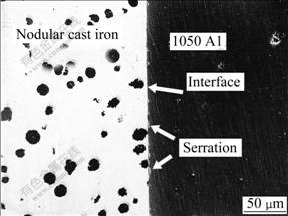
Fig.4 Cross section microstructure in zone 4 at interface
According to the results of VARIS and FROST [13], the heat generation rate (q) per cycle per unit interfacial area is
q=μpωr (1)
where μ is the coefficient of friction, p is the friction pressure, ω is the angular velocity and r is the diameter of materials. Obviously, the heat input on the friction surface increases linearly with the radii of materials. Theoretically, the differences of heat input are inevitable. Only through optimizing the geometries of materials and welding parameters can the difference be limited into an appropriate degree. In the present case, the heat input in zone 4 is much greater than that in zone 2 as a result of the higher linear velocity. The overfull heat input inevitably results in the high temperature, which contributes to the formation of the intermetallic compounds[3,14]. As a consequence, numbers of FexAly intermetallic compounds are formed in zone 4.
3.2 Strength distribution at interface and morphology of fracture surface
The bond strength in the different zones at the interface, which is schematically shown in Fig.2, was calculated using the following equation:
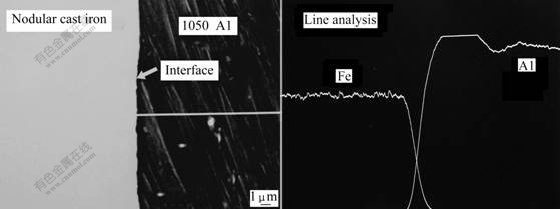
Fig.5 EMPA line analysis in zone 2 at interface
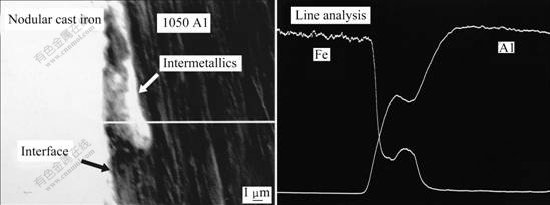
Fig.6 EMPA line analysis in zone 4 at interface
(2)
where σn is tensile strength in different zones at the interface (MPa), Fn and Fn-1 are fraction loads of the specimens with different diameters (N), An and An-1 are areas of fraction surface with different diameters (mm2) and n is the sequence numbers of different zones in radial direction at interface.
Fig.7 illustrates the distribution of the bond strength in the radial direction at the interface. The results indicate that the local bond strength at the interface increases slightly with the increase of the radii, and then decreases sharply when the radii is up to 5 mm. That is to say, the bond strength is high in the central area (zones 1 and 2) at the interface, but the strength drops dramatically in the peripheral area (zones 3, 4 and 5) at the interface. The former results reveal that the superabundant intermetallic compounds have negative effects on the bond strength at the interface[15-16]. In this work, based on the microstructure of interface, the decrease of the bond strength attributes to numbers of FexAly intermetallic compounds in the peripheral area at the interface.
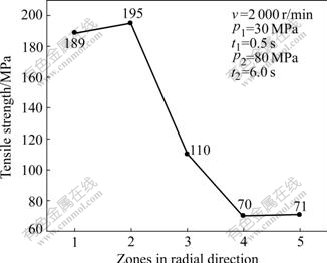
Fig.7 Distribution of strength in radial direction at interface
The morphology on the fracture surface is shown in Fig.8. The facture is obviously characterized by the ductile micro-void mode in the central area. The amount of tearing ridge gradually decreases from the central area to the peripheral area. Based on the observation of microstructure, numbers of FexAly intermetallic compounds are formed in the peripheral area in the course of welding, which destroys the direct joining between Al and nodular cast iron. Furthermore, neither Al nor ductile iron is sound integrated with FexAly intermetallic compounds, which inevitably reduces the effectual joining area. So the bond strength decreases in the peripheral area. However, as for the central area, the negative effects of the intermetallic compounds decrease as a result of the appropriate heat input, and Al can be directly joined with nodular cast iron by plastic deformation and atom diffusion. The distribution of strength is in conformity with the microstructure at the interface.
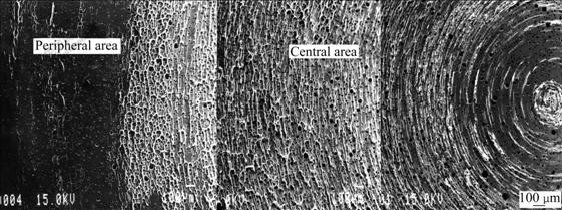
Fig.8 SEM morphology of fracture surface on side of nodular cast iron
4 Conclusions
1) No intermetallic compound exists in the central areas. However, numbers of intermetallic compounds (FexAly), which inevitably reduces the effective joining area, are formed in the peripheral areas as a result of the overfull heat input.
2) The distribution of the strength in the radial direction at the interface is inhomogeneous due to the different heat inputs, and the bond strength in the central areas is obviously higher than that in the peripheral areas.
3) The facture in the central area is obviously characterized by the ductile micro-void mode.
References
[1] SATYANARAYANA V V, REDDY G M, MOHANDAS T. Dissimilar metal friction welding of austenitic-ferritic stainless steels [J]. J Mater Process Technol, 2005, 160(2): 128-137.
[2] OCHI H, OGAWA K, YAMAMOTO Y, KAWAI G, SAWAI T. The formation of intermetallic compounds in aluminium alloy to copper friction-welded joints and their effect on joint efficiency [J]. Welding International, 2004, 18(7): 516-523.
[3] SAHIN A Z, YIBAS B S, AHMED M, NICKEL J. Analysis of the friction welding process in relation to the welding of copper and steel bars [J]. J Mater Process Technol, 1998, 82(1/3): 127-136.
[4] ARITOSHI M, OKITA K. Friction welding of dissimilar metals [J]. Welding International, 2003, 17(4): 271-275.
[5] YOKOYAMA T, OGAWA K. Impact tensile properties of 6061 aluminium alloy to SUS 304 stainless steel friction-welded butt joints [J]. Welding International, 2003, 17(7): 514-523.
[6] KIM S Y, JUNG S B, SHUR C C, YEON Y M, KIM D U. Mechanical properties of copper to titanium joined by friction welding [J]. J Mater Sci, 2003, 38(6): 1281-1287.
[7] FU L, DU S B, JIE W Q. Effect of external electric field on microstructure and diffusion behavior of friction welding joint of dissimilar materials during post weld annealing treatment [J]. Transactions of Materials and Heat Treatment, 2003, 24(1): 73-77.
[8] LEE W B, KIM M G, KOO J M, KIM K K, QUESNEL D J, KIM Y J, JUNG S B. Friction welding of TiAl and AlSi4140 [J]. J Mater Sci, 2004, 39(3): 1125-1128.
[9] SAHIN M. Joining with friction welding of high-speed steel and medium-carbon steel [J]. J Mater Process Technol, 2005, 168(2): 202-210.
[10] MALDONADO C, NORTH T H. Softened zone formation and joint strength properties in dissimilar friction welds [J]. J Mater Sci, 2002, 37(10): 2087-2095.
[11] YIBAS B S, SAHIN A Z, KAHRAMAN N, GARNI A Z A. Friction welding of St-A1 and A1-Cu materials [J]. J Mater Process Technol, 1995, 49(3/4): 431-443.
[12] OZDEMIR N. Investigation of the mechanical properties of friction-welded joints between AlSi 304L and AlSi 4340 steel as a function rotational speed [J]. Mater Lett, 2005, 59(19/20): 2504-2509.
[13] VARIS A, FROST M. On the extrusion stage of linear friction welding of Ti6Al4V [J]. Mater Sci Eng A, 1999, 271(1/2): 477-484.
[14] LEE W B, BANG K S, JUNG S B. Effects of intermetallic compound on the electrical and mechanical properties of friction welded Cu/Al bimetallic joints during annealing [J]. J Alloy Compd, 2005, 390(1/2): 212-219.
[15] YILMAZ M, COL M, ACET M. Interface properties of aluminum/steel friction-welded components [J]. Materials Characterization, 2003, 49(5): 421-429.
[16] ABBASI M, TAHERI A K, SALEHI M T. Growth rate of intermetallic compounds in Al/Cu bimetal produced by cold roll welding process [J]. J Alloy Compd, 2001, 319(1/2): 233-241.
Foundation item: Project supported by the 985 Program of Jilin University, China
Corresponding author: LIU Yao-hui; Tel: +86-431-5095862; Fax: +86-431-5686346; E-mail: liuyaohui2005@yahoo.com
(Edited by LI Xiang-qun)