
Ferroic domain characterization of Ni55Mn20.6Ga24.4 ferromagnetic shape memory alloy
SONG Hong-zhang1, ZENG Hua-rong2, LI Yong-xiang2, YIN Qing-rui2, HU Xing1
1. Key Laboratory of Material Physics of Ministry of Education, School of Physical and Engineering,
Zhengzhou University, Zhengzhou 450052, China;
2. Shanghai Institute of Ceramics, Chinese Academy of Sciences, Shanghai 200050, China
Received 20 September 2010; accepted 16 December 2010
Abstract: The microstructure and coupling between structural and magnetic domains of ferromagnetic shape memory alloy Ni55Mn20.6Ga24.4 were investigated by scanning electron acoustic microscopy (SEAM). Stripe ferroelastic domains (martensite variants) exist in every grain, and exhibit the configurations of the typical self-accommodation arrangement. Magnetic domain structure of Ni55Mn20.6Ga24.4 was observed by the Bitter method and magnetic force microscopy (MFM). Due to the unique subsurface imaging capability of SEAM, combined with the Bitter method, the ferroelastic domain structure can be compared with in situ ferromagnetic domain structure. It is found that the martensitic variant boundaries coincide well with the ferromagnetic domain walls, which is beneficial for the understanding of the correlation between two kinds of ferroic domains.
Key words: ferroic domain; ferromagnetic shape memory alloy; scanning electron acoustic microscopy
1 Introduction
The ferroic (ferromagnetic, ferroelectric, and ferroelastic/martensitic) materials exhibiting one or more order parameters are important functional materials. For Ni-Mn-Ga ferromagnetic shape memory alloys (FSMAs), they contain both ferroelastic and ferromagnetic domains, and have attracted great attention due to their large magnetic-field-induced strain (6.0%-9.5%) in the martensitic state [1]. It is well known that ferroic domains play a central role in controlling all the properties of ferroic materials, in particular the ferroic properties. Therefore, the characterization of domain structure in ferroic materials is very important. So far, ferroelastic domains (martensite variants) of Ni-Mn-Ga alloys have been investigated widely by optical microscopy [2-3] and transmission electron microscopy (TEM) [4], and their magnetic domains have been also investigated extensively by various imaging techniques, e.g., Bitter and scanning electron microscopy (SEM) [5], magnetic force microscopy (MFM) [6], interference- contrast-colloid (ICC) [7], Lorentz microscopy, and electron holography [8-9]. However, the study on the correlations between the ferroelastic and ferromagnetic domain structure of FSMA remains scarce.
Due to the unique imaging mechanism of scanning electron acoustic microscopy (SEAM) [10], it exhibits a subsurface layered imaging ability by tuning the modulating frequencies. It is thus expected that the SEAM combined with the Bitter method should be an effective technique to investigate the relations between ferroelastic and magnetic domains. In this work, ferroelastic domain structure, ferromagnetic domain structure and their relations of Ni55Mn20.6Ga24.4 alloys were investigated by the SEAM, the Bitter method and the MFM.
2 Experimental
Polycrystalline Ni55Mn20.6Ga24.4 alloys were prepared by vacuum arc remelting (VAR) using high-purity (>99.9%) raw materials of Ni, Mn, and Ga, and were then homogenized at 1 073 K for 24 h and quenched into cold water. The alloys were sliced into an appropriate thickness, and their surfaces were polished
mechanically to a thickness of 1.0 mm. After the polishing process, the alloys were diced into the specimens with the dimensions of 5.0 mm in length and 4.0 mm in width.
The ferroelastic domain structure was observed by the SEAM modified from a commercial SEM (KYKY-EM3200) [10]. An electron acoustic image (EAI) and a secondary electron image (SEI) can be obtained in situ simultaneously. The ferromagnetic domain structure was obtained by the Bitter method and MFM (Nanoscope Ⅲa Multimode Scanning Probe Microscope).
3 Results and discussion
Figure 1(a) shows the SEI of a Ni55Mn20.6Ga24.4 sample, and Fig.1(b) shows its in situ EAI at modulating frequencies of 136.0 kHz. In the SEI, no special information is shown. While, from the EAI, typical polycrystalline boundaries or twin boundaries with grain size of 400-900 ?m can be seen. In corresponding grains, stripe or lamellar martensite variants (ferroelastic domains) aligning along different directions exist in each grain, and exhibit a typical self-accommodation arrangement. The domain width varies from 10 to 100 ?m. These results are similar to those of the nonstoichiometric Ni2MnGa alloy observed by the optical microscopy [3]. However, compared with common optical observation of metallographic topography, the sample for SEAM imaging does not need to be etched with specific acid. In addition, a subsurface defect region as labeled is also revealed by the EAI, which exhibits the unique imaging advantage of the SEAM.
Figures 1(c) and 1(d) show the SEI and the EAI (at a modulating frequency of 160.6 kHz) of another spot of the same sample. They also exhibit regular lamellar variants clearly. Some holes as marked on the surface are seen in situ in the both SEI and EAI. Especially, in the region designated by the symbols A, B and C, martensite variants cross the grain boundaries or the cracks as marked, and form twin domain structure. In the region designated by the symbol D, variants intersect here, and extend themselves into each other. In this EAI, the domain width varies from 10 to 30 ?m.
Figure 2(a) shows the SEI giving the ferromagnetic domain structure of Ni55Mn20.6Ga24.4 alloy in the Bitter pattern. Though the effect is not perfect, the shape of the domain walls can be distinguished as stripe or lamellar, which domains in different regions align in different directions. Figure 2(b) shows the EAI at a modulating frequency of 182.0 kHz. Stripe martensite variants are clearly seen. By comparing Fig.2(a) with 2(b) in situ, an interesting phenomenon is found that the ferromagnetic domain walls are well coincident with the martensite variant boundaries. Though SOLOMON et al [8], MURAKAMI et al [9], and BRINTLINGER et al [11] reported the similar coincidence between martensite plate boundaries and domain walls in the nano-scale of Ni-Mn-Ga by Lorentz microscopy, in the micron-scale, most investigations are focused on the 180° magnetic domains crossing the martensite variants [5, 12] or on the fine magnetic domains superimposed upon the martensite variants [7, 13-14]. In this work, the direct evidence of the coincidence between micron-scale (or sub-millimeter-scale) martensite variants (also as ferroelastic domains) and magnetic domains was provided. This conclusion is consistent with our early investigation from a similar material Mn50Ni28Ga22 [15], and the theory of KISELEV et al [16].
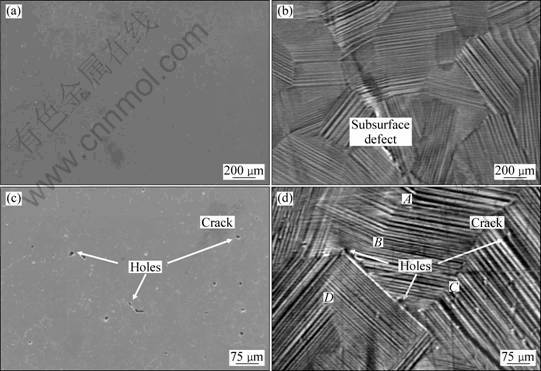
Fig. 1 SEI of Ni55Mn20.6Ga24.4 sample (a), in situ EAI at modulating frequency of 136.0 kHz (b), SEI of another spot of same sample (c), in situ EAI at modulating frequency of 160.6 kHz (d)
Figures 3(a) and 3(c) show the topography of the same sample at two regions, and only some surface defects or impurities are seen. Figures 3(b) and 3(d) are their in situ MFM images, respectively. The MFM images show a higher resolution than the EAI. It can be seen that the primary stripe ferromagnetic domains marked with lines are observed, and the domain width is 8-12 ?m. The sub-domains in side of primary domains are irregular stripe, and the domain width is 2 ?m. This particular multilayer magnetic domain structure is similar to the herringbone ferroelectric domain pattern in ferroelectrics due to the demand of the minimum domain wall energy [17-18].
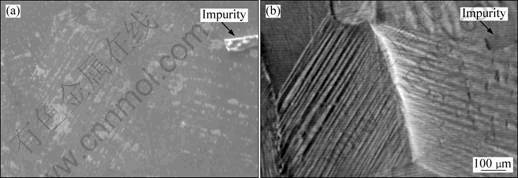
Fig. 2 SEI of Ni55Mn20.6Ga24.4 sample in Bitter pattern (a) and in situ EAI at modulating frequency of 182.0 kHz (b)
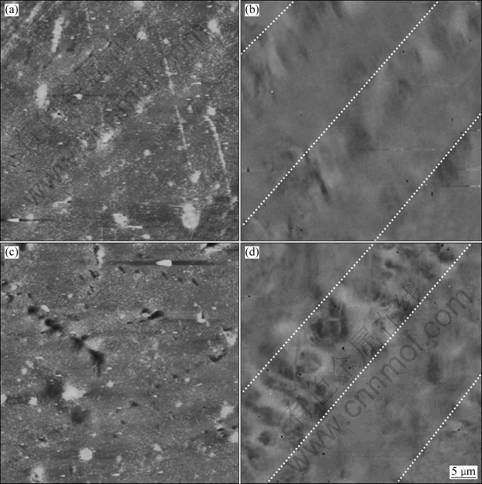
Fig. 3 Topography of a Ni55Mn20.6Ga24.4 sample (a), in situ MFM (b), and topography of another spot of same sample (c) and in situ MFM (d)
By comparing the sizes of primary ferromagnetic domains and martensite variants, it is found that they have approximate size or width, which also means that ferromagnetic domains coincide with ferroelastic domain. However, this MFM characteristic is different with Refs. [13] and [14] also based on MFM, in which uniformly spaced magnetic Weiss domains terminate at the interface of the martensitic domains. The reason of this inconsistency is not known now, and needs to explore farther.
4 Conclusions
1) A direct evidence of the coincidence between micron-scale martensite variants (also as ferroelastic domains) and magnetic domains is obtained. The good coincidence is also proved by MFM indirectly.
2) The experimental results indicate that the SEAM technique combined with the Bitter method can be used as an effective method to observe the relationship between magnetic domains and martensites. These results will be beneficial for the development of ferromagnetic shape memory alloys and for the understanding of the correlation between magnetic domains and crystallographic variant domains as well.
References
[1] SOZINOV A, LIKHACHEV A A, LANSKA N, ULLAKKO K. Giant magnetic-field-induced strain in NiMnGa seven-layered martensitic phase [J]. Appl Phys Lett, 2002, 80: 1746-1-1746-3.
[2] GE Y, HECZKOO, SODERBERG O, HANNULA S P. Direct optical observation of magnetic domains in Ni-Mn-Ga martensite [J]. Appl Phys Lett, 2006, 89: 082502-1-082502-3.
[3] MA Yun-qing, JIANG Cheng-bao, LI Yan, XU Hui-bing, WANG Cui-ping, LIU Xing-jun. Microstructure and high-temperature shape-memory effect in Ni54Mn25Ga21 alloy [J]. Transactions of Nonferrous Metals Society of China, 2006, 16: 502-506.
[4] LIU C, GAO Z Y, AN X, SAUNDERS M, YANG H, WANG H B, GAO L X, CAI W. Microstructure and magnetic properties of Ni-rich Ni54Mn25.7Ga20.3 ferromagnetic shape memory alloy thin film [J]. J Magn Magn Mater, 2008, 320: 1078-1082.
[5] HECZKO O, JUREK K, ULLAKKO K. Magnetic properties and domain structure of magnetic shape memory Ni-Mn-Ga alloy [J]. J Magn Magn Mater, 2001, 226-230: 996-998.
[6] PAN Q, JAMES R D. Micromagnetic study of Ni2MnGa under applied field (invited) [J]. J Appl Phys, 2000, 87: 4702-1-4702-5.
[7] CHOPRA H D, JI C, KOKORIN V V. Magnetic-?eld-induced twin boundary motion in magnetic shape-memory alloys [J]. Phys Rev B, 2000, 61: 14913-1-14913-5.
[8] SOLOMON V C, MCCARTNEY M R, SMITH D J, TANG Y J, BERKOWITZ A E, OHANDLEY R C. Magnetic domain configurations in spark-eroded ferromagnetic shape memory Ni-Mn-Ga particles [J]. Appl Phys Lett, 2005, 86: 192503-1-192503-3.
[9] MURAKAMI Y, SHINDO D, SUZUKI M, OHTSUKA M, ITAGAKI K. Magnetic domain structure in Ni53.6Mn23.4Ga23.0 shape memory alloy films studied by electron holography and Lorentz microscopy [J]. Acta Mater, 2003, 51: 485-494.
[10] YIN Qing-rui, ZHU Bing-he. Microstructure, property and processing of functional ceramics [M]. Shanghai: Metallurgical Industry Press,2005: 143-170. (in Chinese)
[11] BRINTLINGER T, LIM SH, BALOCH K H, ALEXANDER P, QI Y, BARRY J, MELNGAILIS J, SALAMANCA-RIBAL L, TAKEUCHI I, CUMINGS J. In situ observation of reversible nanomagnetic switching induced by electric fields [J]. Nano Lett, 2010, 10: 1219-1223.
[12] GE Y, HECZKO O, SODERBERG O, HANNULA S P. Magnetic domain evolution with applied field in a Ni-Mn-Ga magnetic shape memory alloy [J]. Scr Mater, 2006, 54: 2155-2160.
[13] SULLIVAN M R, CHOPRA H D. Temperature- and field-dependent evolution of micromagnetic structure in ferromagnetic shape-memory alloys [J]. Phys Rev B, 2004, 70: 094427-1-094427-8.
[14] JAIN D, BANIK S, CHANDRA L S S, BARMAN S R, NATH R, GANESAN V D. Domain structures across the martensitic transformation in Ni2+xMn1-xGa [J]. Materials Science Forum, 2010, 63: 69-74.
[15] SONG H Z, LI Y X, ZENG H R, MA L, WU G H, HUI S X, LI G R, YIN Q R. Electron acoustic imaging of Mn50Ni28Ga22 ferromagnetic shape memory alloy [J]. Appl Phys A, 2008, 92: 309-311.
[16] KISELEV N S, DRAGUNOV I E, ONISAN A T, ROBLER U K, BOGDANOV A N. Theory of stripe domains in magnetic shape memory alloys [J]. Eur Phys J Special Topics, 2008, 158: 119-124.
[17] ZHAO K Y, ZENG H R, SONG H Z, HUI S X, LI G R, LUO H S, YIN Q R. Intrinsic stress-induced adaptive ferroelectric domain rearrangement in the monoclinic Pb(Mg1/3Nb2/3)O3-PbTiO3 single crystal [J]. Mater Lett, 2009, 63: 1715-1718.
[18] ANBUSATHAIAH V, KAN D, KARTAWIDJAJA F C, MAHJOUB R, ARREDONDO M A, WICKS S, TAKEUCHI I, WANG J, NAGARAJAN V. Labile ferroelastic nanodomains in bilayered ferroelectric thin films [J]. Adv Mater, 2009, 21: 3497-3502.
Ni55Mn20.6Ga24.4铁磁形状记忆合金的铁性畴结构表征
宋红章1,曾华荣2,李永祥2,殷庆瑞2,胡 行1
1. 郑州大学 物理工程学院 材料物理教育部重点实验室,郑州 450052;
2. 中国科学院 上海硅酸盐研究所,上海 200050
摘 要:采用扫描电声显微技术(SEAM)研究Ni55Mn20.6Ga24.4铁磁形状记忆合金的马氏体变体晶体畴与铁磁畴的微观结构及其耦合。每个晶粒内部都存在条状的马氏体孪生变体,并呈现典型的自协作组态。利用SEAM独特的亚表面成像能力,结合Bitter粉纹法原位对比了马氏体变体与磁畴的相互关系,发现马氏体变体与磁畴具有良好的重合性。利用SEAM和MFM两种技术证明了这个结论。该结果对进一步理解磁畴和马氏体变体晶体畴的相互关系有重要意义。
关键词:铁性畴;铁磁形状记忆合金;扫描电声显微术
(Edited by LI Xiang-qun)
Foundation item: Project (2009CB623305) supported by the National Basic Research Program of China; Project (50821004) supported by the Foundation for Innovative Research Groups of the National Natural Science Foundation of China
Corresponding author: SONG Hong-zhang; Tel: +86-371-67766013; E-mail: songhongzhang@hotmail.com
DOI: 10.1016/S1003-6326(11)60965-5