J. Cent. South Univ. Technol. (2007)01-0073-05
DOI: 10.1007/s11771-007-0015-2

New amperometric glucose biosensor by entrapping glucose oxidase into chitosan/nanoporous ZrO2/multiwalled carbon nanotubes nanocomposite film
WEI Wan-zhi(魏万之)1, ZHAI Xiu-rong(翟秀荣)2, ZENG Jin-xiang(曾金祥)1,
GAO Yan-ping(高艳萍)1, GONG Shu-guo(龚淑果)1
(1. State key Laboratory of Chemo-Biosensing and Chemometrics, School of Chemistry and Chemical Engineering, Hunan University, Changsha 410082, China;
Abstract: A new nanocomposite material for construction of glucose biosensor was prepared. The biosensor was formed by entrapping glucose oxidase(Gox) into chitosan/nanoporous ZrO2/multiwalled carbon nanotubes nanocomposite film. In this biosensing thin film, the multiwalled carbon nanotubes can effectively catalyze hydrogen peroxide and nanoporous ZrO2 can enhance the stability of the immobilized enzyme. The resulting biosensor provides a very effective matrix for the immobilization of glucose oxidase and exhibits a wide linear response range from 8 μmol/L to 3 mmol/L with a correlation coefficient of 0.994 for the detection of glucose. And the response time and detection limit of the biosensor are determined to be 6 s and 3.5 μmol/L, respectively. Another attractive characteristic is that the biosensor is inexpensive, stable and reliable.
Key words: biosensor; nanocomposite; glucose oxidase; nanoporous ZrO2; multiwalled carbon nanotubes; chitosan
1 Introduction
In recent years, much interest has been attributed to construct glucose biosensors, because the determination of glucose is very important for the human metabolic diagnose and agricultural applications[1]. For construction of biosensor, the method for immobilization of enzymes is very important. Some methods including cross-linking to a matrix, entrapment within the membrane and microencapsulation into polymer microspheres, hydrogels, are continuously developed[2-3]. However, some techniques are somewhat complex and the enzyme can be easily denatured and leached out from the matrix.
ZrO2 is an inorganic ceramic material with very high mechanical strength and enhanced thermal stability in solution. Nanoporous ZrO2 was used as a matrix for immobilization of enzyme[4-5]. However, the application of the inorganic ceramic material was limited because of its brittleness. To overcome this disadvantage, nanoporous ZrO2 is often combined with organic polymers to form inorganic-organic materials because the inorganic-organic materials possess the advantages of organic component’s toughness and inorganic phase’s chemical and thermal stability[5].
Carbon-nanotubes (CNTs) lead to many new technical developments and applications due to large surface area, unique electronic properties, and relatively high mechanical strength[6]. It is found that CNTs have high electrocatalytic effect and fast electron-transfer rate[7-11]. Besides, CNTs can minimize the surface fouling onto electrochemical devices without mediator. The ability of CNTs to promote the electron transfer of hydrogen peroxide (H2O2) and NADH etc suggestes that CNTs have great promise as oxidase-based and dehydrogenase-based amperometric biosensors[11-12].
Chitosan(CHIT), a natural biopolymer, exhibits excellent film forming ability, high permeability toward water. It has gained growing interest to be used in immobilizing of biomolecules[13-14]. Nanocomposite of CHIT/CNTs was prepared[11-12] and used to covalently immobilize glucose dehydrogenase by cross-linking of glutaraldehyde (GA). But in the method, the unreacted excess GA must be removed from the mixture by multiple extractions with ethyl ether because GA can make enzyme denatured[11,15]. So the method of immobilizing enzyme using GA is very complex and noneffective.
In this study, a new nanocomposite was developed by dispersion of nanoporous ZrO2 and multiwalled carbon nanotubes (MWNTs) in the matrix of biopolymer CHIT. By entrapping glucose oxidase (Gox) into the CHIT/MWNTs/ZrO2 nanocomposite, a glucose biosen- sor was prepared.
2 Experimental
2.1 Apparatus and reagents
Scanning electron microscopy (SEM) image was obtained from JSM 6700 LV (Japan) operating at 5.0 kV. Cyclic voltammetry and amperometric measurements were performed with CHI 660A electrochemical station. A conventional three-electrode system using the modified glassy carbon (GC) electrode as working electrode, a saturated calomel electrode(SCE) as reference electrode and a platinum foil electrode as counter electrode was used. All applying potentials were measured and reported vs the SCE, and all experiments were carried out at room temperature.
Glucose oxidase (E.C 1.1.3.4 from Aspergillus niger, type VII-S; 196×103 unit/g) and chitosan (CHIT, relative molecular mass of 1×106; 75%-85% deacety- lation) were produced in Sigma (St. Louis, Mo, USA). Nanoporous ZrO2 was supplied by Nano Material Application Engineering Technology Center (Zhejiang, China). MWNTs with 95% purity were purchased from Shenzhen Nanotech Port Co., Ltd. (Shenzhen, China). Nafion was obtained from Aldrich. β-D glucose was purchased from ICN Biomedicals Inc. (USA). Glucose stock solutions were allowed to rotate at room temperature overnight before use. All other reagents were of analytical grade. Phosphate buffer solution (0.067 mol/L, pH=7.0) was used as the supporting electrolyte in all measurements, and redistilled water was used throughout.
2.2 Preparation of CHIT/ZrO2 /MWNTs solution
MWNTs were functionalized according to Ref.[16]. Appropriate amount of nanoporous ZrO2 was dispersed in 0.3% (mass fraction) of CHIT (2% acetic acid), and the mass ratio of ZrO2 to CHIT was 1?100. The mixture was sonicated for 15 min after stirring for 1 h until a high dispersed colloidal solution was formed. Then the functionalized MWNTs (0.6-6.0 g/L) were dispersed into CHIT/ZrO2 solution by ultrasonic agitation to give a black suspension.
2.3 Preparation of CHIT/MWNTs solution
For preparation of CHIT/MWNTs solution, the functionalized MWNTs (6 g/L) were added into in 0.3% (mass fraction) of CHIT (2% acetic acid) by ultrasonic agitation to get uniformly dispersed solution.
2.4 Preparation of CHIT/ZrO2/MWNTs/Gox Nafion glucose biosensor
Prior to the surface modification, the GC electrode was first polished with alumina slurry (followed by 0.3 mm and 0.05 mm), then rinsed thoroughly with redistilled water, and ultrasonically agitated successively in ethanol and redistilled water, each for 10 min. Enzyme solution with the Gox concentration of 30 g/L was prepared in phosphate buffer solution (0.067 mol/L, pH=7.0). The glucose biosensor was constructed by mixing 10 mL of Gox solution with 10 mL of CHIT/ZrO2/MWNTs solution, and then dropping 6 mL of the composite solution on the GC electrode surface. The glucose biosensor was dried at 4 ℃. Then 10 mL of 0.25% Nafion solution was dropped onto the enzyme electrode surface and dried in air. When not in use, the electrode was stored dry at 4 ℃ in refrigerator.
2.5 Preparation of CHIT/MWNTs/Gox glucose biosensor
The casting solution was obtained by mixing 10 mL of CHIT/MWNTs composite solution with 10 mL of enzyme solution (30 g/L). Then 6 mL of the resulting casting solution was pipetted onto the GC electrode surface. The glucose biosensor was dried at 4 ℃.
3 Results and discussion
3.1 SEM image of CHIT/ZrO2/MWNTs nanocompo-site film
Homogenization within the nanocomposite film is a key factor in the construction of biosensor. Fig.1 shows the SEM image of CHIT/ZrO2/MWNTs nanocomposite film. As can be seen, both of nanoporous ZrO2 and MWNTs distribute homogeneously in the CHIT film. MWNTs can act as high conductivity wires connecting nanocomposite film domains throughout the film. Besides, nanoporous ZrO2 and MWNTs can bind to glucose oxidase effectively[4-5,17]. So, this nanocomposite film offers an effective matrix to immobilize enzyme and is beneficial to the special response between enzyme and substrate.
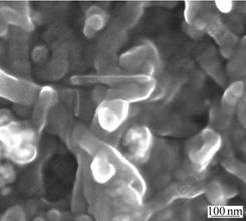
Fig.1 SEM image of CHIT/ZrO2/MWNTs nanocomposite film
3.2 Effect of CHIT/ZrO2/MWNTs/GC electrode of electrochemical catalytic behavior of H2O2
H2O2 is produced by the enzymatic reaction. To evaluate the catalytic activity toward H2O2 at the CHIT/ ZrO2/MWNTs/GC electrode, the modified electrode was characterized by cyclic voltammogram in the presence of H2O2 at 0-1.2 V (vs SCE). Fig.2 shows the cyclic voltammograms at the CHIT/ZrO2/MWNTs/GC electrode in phosphate buffer solution with and without H2O2. After addition of 2 mmol/L H2O2 into buffer solution, the oxidation current increases. The result indicates that the CHIT/ZrO2/MWNTs nanocomposite can be applied to fabricate biosensors based on the determination of H2O2.
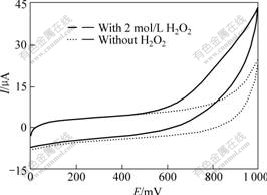
Fig.2 Cyclic voltammograms at CHIT/ZrO2/MWNTs/GC electrode in phosphate buffer solution (pH=7.0) at scan rate of 50 mV/s
3.3 Effect of MWNTs in nanocomposite materials on catalysis of H2O2
As shown in Fig.3, the GC electrode modified with MWNTs and nanoporous ZrO2 has a remarkable response to H2O2, while the GC electrode modified with only nanoporous ZrO2 has very small current toward the catalysis of H2O2. This indicates that MWNTs play an important role in improving the electron transfer and catalyzing of H2O2. The content of MWNTs in the nanocomposite material is one of the crucial factors on the response of CHIT/ZrO2/MWNTs/GC electrode. Amperometric responses were compared at the CHIT/ ZrO2/MWNTs/GC electrodes by varying the amount of MWNTs from 0.6 to 6.0 g/L on subsequent addition of 2 mmol/L H2O2 in phosphate buffer solution (pH=7.0) at the applied potential of 0.6 V (vs SCE). As can be observed, the response increases with increasing the amount of the MWNTs. However, further increase of the MWNTs content will cause the instability of nanocomoposite and the leakage of Gox. Thus the amount of MWNTs was selected as 6.0 g/L in the experiments.
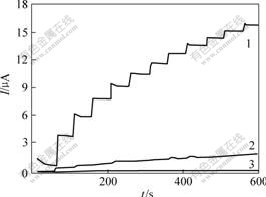
Fig.3 Dynamic current time response of CHIT/ZrO2/ MWNTs/GC electrode with different MWNTs contents to successive addition of 2 mmol/L H2O2 in 0.067 mol/L phosphate buffer (pH 7.0) at applied potential of 0.6 V (vs SCE)
ρ(MWNT)/(g?L-1): 1—6.0; 2—0.6; 3—0
3.4 Effect of two different nanocomposite materials on enzyme immobilization
To investigate the effect of nanoporous ZrO2 on the enzyme immobilization, the successive response of the biosensors prepared by nanocomposite with and without nanoporous ZrO2 were compared. As shown in Fig.4, CHIT/ZrO2/MWNTs nanocomposite material is more stable than CHIT/MWNTs nanocomposite material on the immobilization of Gox. This behavior indicates that nanocomposite prepared with nanoporous ZrO2 can enhance the stability of the immobilized enzyme. The good stability of CHIT/ZrO2/MWNTs/Gox biosensor may be mostly due to the following reasons. Firstly, ZrO2 has general affinity for the binding of enzyme because the amine and carboxyl groups on the surface of enzyme can act as ligands to ZrO2[4-5]. Secondly, MWNTs provide negative functionalities to bind to positive charged Gox[17]. Thirdly, CHIT/ZrO2/MWNTs nanocomposite is an organic-inorganic composite material which can display the advantages of toughness of CHIT and chemical and thermal stability of nanoporous ZrO2.
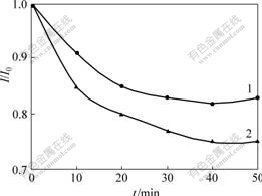
Fig.4 Successive responses of different biosensors to 0.5 mmol/L glucose (I is current of successive response and I0 is initial current)
1—CHIT/ZrO2/MWNTs/Gox biosensor;2—CHIT/MWNTs/Gox biosensor
3.5 Influential factors on response characteristics of CHIT/ZrO2/MWNTs/Gox biosensor
Because the biosensor performance may be related to the enzyme loading, the effect of Gox concentration on the response of the biosensor was examined. Fig.5 shows the calibration curves of the enzyme electrode with different concentrations of Gox loadings at the applied potential of 0.6 V (vs SCE). When the enzyme loading is fixed,the current response increases with increasing glucose concentration. When glucose concentration is fixed, the current response increases in the enzyme loading range from 7.5 and 30.0 g/L. However, no significant improvement can be observed when the Gox concentration is increased beyond 30.0 g/L. So the Gox concentration of 30.0 g/L is selected in this experiment.
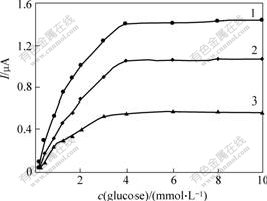
Fig.5 Effect of Gox concentration on responses of CHIT/ZrO2/MWNTs/Gox biosensor in 0.067 mol/L phosphate buffer (pH=7.0) at 0.6 V(vs SCE)by amperometric measurement of glucose
ρ(Gox)/(g?L-1): 1—30.0; 2—15.0; 3—7.5
The effect of pH value of the detected solution on the response behavior of the biosensor was studied between pH=5.5 and 9.0, and the corresponding results are shown in Fig.6. The highest amperometric response is obtained around pH=7.0. This is in accordance with the optimum pH value of Gox in Ref.[5]. Based on the above results, the pH value of 7.0 is selected in all experiments.
Response currents of the biosensor to 1 mmol/L glucose in 0.067 mol/L phosphate buffer solution (pH=7.0) at different applied potentials are shown in Fig.7. At 0.4 V, small response currents can be observed. With the increase of the applied potential, the response current to glucose can be observed obviously. However, to avoid interference at high applied potential, the potential of 0.6 V (vs SCE) is selected as the applied potential for amperometric measurements.
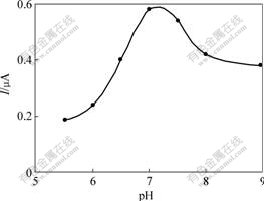
Fig.6 Effect of pH value of phosphate buffer solution on biosensor response by amperometric measurement of 1 mmol/L glucose at 0.6 V(vs SCE)
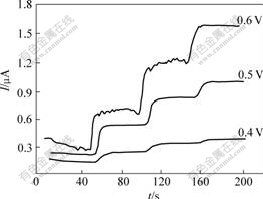
Fig.7 Current response of CHIT/ZrO2/MWNTs/Gox biosensor to addition of 1 mmol/L glucose at various applied potentials in 0.067 mol/L phosphate buffer solution (pH=7.0)
3.6 Amperometric determination of glucose with biosensor
Under the optimized experimental conditions, the biosensor was used to determinate glucose. Fig.8 shows a typical current—time response of the biosensor on successive addition of 0.2 mmol/L glucose in 0.067 mol/L phosphate buffer solution (pH=7.0) at 0.6 V (vs SCE). A subsequent addition of glucose to the stirring phosphate buffer solution leads to a remarkable increase in the oxidation current, and the time required to reach the 95% steady state response is within 6 s. The proposed biosensor presents a linear range from 8 mmol/L to 3 mmol/L (correlation coefficient R=0.994) with a detection limit of 3.5 mmol/L. And the linear part of the calibration curve is shown in Fig.9. This high sensitivity in detection of glucose suggests that the CHIT/ZrO2/ MWNTs nanocomposite well retains the electrochemical catalytical activity of MWNTs and provides the enzyme with a biocompatible microenvironment.
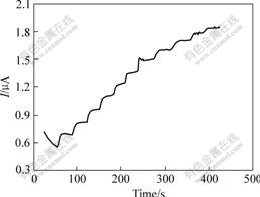
Fig.8 Amperometric responses of CHIT/ZrO2/MWNTs/Gox biosensor to subsequent additions of 0.2 mmol/L glucose
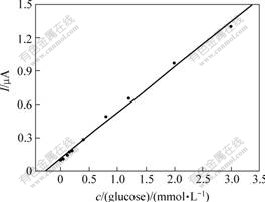
Fig.9 Linear part of glucose biosensor in 0.067 mol/L phosphate buffer (pH=7.0) at 0.6 V(vs SCE)
A coating of Nafion as an effectively perselective barrier was used to eliminate the interference of ascorbic acid (AA) and uric acid (UA) in the determination of glucose[18]. In a Nafion-coated CHIT/ZrO2/MWNTs/Gox biosensor, the addition of 0.1 mmol/L AA or 0.1 mmol/L UA does not affect the amperometric response of 1 mmol/L glucose, which indicates that the coating of the Nafion membrane can effectively eliminate the interference of AA and UA.
The biosensor is stored at 4 ℃ when not in use. It remains about 80% of its original sensitivity after 28 d. Good long-term stability may result from the stability and biocompatibility of the nanocomposite film.
4 Conclusions
1) Glucose biosensors with good stability and sensitive response were prepared by entrapping Gox into CHIT/ZrO2/MWNTs nanocomposite film.
2) MWNTs can effectively catalyze and promote the electron-transfer of H2O2 and nanoporous ZrO2 can enhance the stability of the immobilized enzyme. Nanocomposite film can effectively prevent Gox from leaching out and contain high bioactivity of Gox. So, the CHIT/ZrO2/MWNTs nanocomposite has a potential application for designing a variety of oxidase-based amperometric biosensors.
References
[1] RABA J, MOTTOLA H A. Glucose oxidase as an analytical reagent[J]. Crit Rev Anal Chem, 1995, 25: 1-42.
[2] WEETALL H H. Preparation of immobilized proteins covalently coupled through silane coupling agents to inorganic supports[J]. Appl Biochem Biotechnol, 1993, 41(3): 157- 168.
[3] DORETTI L, FERRARA D, LORA S. Enzyme-entrapping membranes for biosensors obtained by radiation-induced polymerization[J]. Biosens Bioelectron, 1993, 8: 443-450.
[4] LIU B H, CAO Y, CHEN D D, et al. Amperometric biosensor based on a nanoporous ZrO2 matrix[J]. Anal Chim Acta, 2003, 478: 59-66.
[5] YANG Y H, YANG H F, YANG M H, et al. Amperometric glucose biosensor based on a surface treated nanoporous ZrO2/chitosan composite film as immobilization matrix[J]. Anal Chim Acta, 2004, 525(2):213-220.
[6] MOORE R R, BANKS C E, COMPTON R G. Basal plane pyrolytic graphite modified electrodes: comparison of carbon nanotubes and graphite powder as electrocatalysts[J]. Anal Chem, 2004, 76(10): 2677-2682.
[7] GONG K P, ZHANG M N, YAN Y M, et al. Sol-gel-derived ceramic-carbon nanotube nanocomposite electrodes: tunable electrode dimension and potential electrochemical applications[J]. Anal Chem, 2004, 76(21): 6500-6505.
[8] LIN Y, RAO A M, SADANADAN B, et al. Functionalizing multi-walled carbon nanotubes with aminopolymers[J] J Phy Chem B, 2002, 106(6): 1294-1298.
[9] JIANG K, EITAN A, SCHADLER L S, et al. Selective attachment of gold nanoparticles to nitrogen-doped carbon nanotubes[J]. Nano Lett, 2003, 3(3): 275-277.
[10] EITAN A, JIANG K Y, DUKES D, et al. Surface modification of multiwalled carbon nanotubes: toward the tailoring of the interface in polymer composites[J]. Chem Mater, 2003, 15(16): 3198-3201.
[11] ZHANG M G, SMITH A, GORSKI W. Carbon nanotube-chitosan system for electrochemical sensing based on dehydrogenase enzymes[J]. Anal Chem, 2004, 76(17): 5045-5050.
[12] JIANG L Y, WANG R X, LI X M, et al. Electrochemical oxidation behavior of nitrite on a chitosan-carboxylated multiwall carbon nanotube modified electrod electrochem[J]. Electrochem Commun, 2005, 597(7): 597-601.
[13] MIAO Y Q, TAN S W. Amperometric hydrogen peroxide biosensor based on immobilization of peroxidase in chitosan matrix crosslinked with glutaraldehyde[J]. Analyst, 2000, 125: 1591-1594.
[14] OKUMA H, WATANABE E. Flow system for fish freshness determination based on double multi-enzyme reactor electrodes[J]. Biosens Bioelectron, 2002, 17(5): 367-372.
[15] POZZO A D, VANINI L, FAGNONI M, et al. Preparation and characterization of poly(ethylenegly(ol)-crosslinked reactylated chitosans[J]. Carbohydrate Polym, 2000, 42: 201-206.
[16] KIM B, SIGMUND W M. Functionalized multiwall carbon nanotube/gold nanoparticle composites[J]. Langmuir, 2004, 20(19): 8239-8242.
[17] TSAI Y C, LI S C, CHEN J M. Cast thin film biosensor design based on a nafion backbone, a multiwalled carbon nanotube conduit, and a glucose oxidase function[J]. Langmuir, 2005, 21(8): 3653-3658.
[18] TANG H, CHEN J H, YAO S Z, et al. Amperometric glucose biosensor based on adsorption of glucose oxidase at platinum nanoparticle-modified carbon nanotube electrode[J]. Analytical Biochemistry, 2004, 331(1): 89-97.
(Edited by CHEN Wei-ping)
Foundation item: Project (20060532006) supported by Specialized Research Fund for the Doctoral Program of Higher Education
Received date: 2006-05-06; Accepted date: 2006-07-05
Corresponding author: WEI Wan-zhi, Professor; Tel: +86-731-8821861; E-mail: weiwz2003@126.com