J. Cent. South Univ. Technol. (2010) 17: 454-459
DOI: 10.1007/s11771-010-0506-4 
Influence of deposition voltage on phase, microstructure and
antioxidation property of cristobalite aluminum phosphate coatings
YANG Wen-dong(杨文冬)1, HUANG Jian-feng(黄剑锋)1, CAO Li-yun(曹丽云)1, ZENG Xie-rong(曾燮榕)2
1. Key Laboratory of Auxiliary Chemistry and Technology for Chemical Industry, Ministry of Education,
Shaanxi University of Science and Technology, Xi’an 710021, China;
2. Shenzhen Key Laboratory of Special Functional Materials, Shenzhen University, Shenzhen 518086, China
? Central South University Press and Springer-Verlag Berlin Heidelberg 2010
Abstract: Cristobalite aluminum phosphate (C-AlPO4) coatings were prepared by a hydrothermal electrophoretic deposition process on SiC-coated C/C composites. Phase compositions and microstructures of the as-prepared coatings were characterized by XRD and SEM analyses. The influence of deposition voltage on the phase, microstructure and antioxidation property of the cristobalite aluminum phosphate coatings was investigated. Results show that the as-prepared coatings are composed of cristobalite aluminum phosphate crystallites. The thickness and density of cristobalite aluminum phosphate coatings are improved with the increase of deposition voltage. The deposition amount and bonding strength of the cristobalite aluminum phosphate coatings also increase with the increase of deposition voltage. The deposition mass per unit area of the coatings and the square root of the deposition time at different hydrothermal voltages satisfy linear relationship. The antioxidation property of the coated C/C composites is improved with the increase of deposition voltage. Compared with SiC coatings prepared by pack cementation, the multilayer coatings prepared by pack cementation with a later hydrothermal electrophoretic deposition process exhibit better antioxidation property. The as-prepared multi-coatings can effectively protect C/C composites from oxidation in air at 1 773 K for 37 h with a mass loss rate of 0.53%.
Key words: carbon/carbon composites; aluminum phosphate; hydrothermal electrophoretic deposition; deposition voltage; micro- structure; antioxidation
1 Introduction
Carbon/carbon (C/C) composites exhibit excellent properties and are considered as the most promising thermo-structural materials with wide applications, such as engine, aerospace and thermonuclear fusion applications where most of the ceramic and metallic materials cannot be used. Only in inert or vacuum environments are they stable up to a very high temperature of 3 273 K, but can be oxidized at a temperature as low as 723 K in the air [1-3]. It is therefore important to increase the resistance of the materials towards air oxidation at high temperature.
In the past several decades, many researches have been conducted on oxidation resistance for C/C composites, and the application of refractory materials coated on the surface of C/C composites is considered to be the best choice. Consequently, most of the researchers focused on the research of silicon-containing ceramic coatings [4]. However, the crack formed by the thermal expansion coefficient mismatch of the coatings and the C/C substrate is an inevitable problem, which results in limited oxidation protection. Recently, the advanced coatings, with a functionally gradient layer to minimize the influence of the thermal expansion coefficient mismatch, have been designed to be multilayer coatings [5-6]. Most of these coatings were prepared by chemical vapor deposition (CVD) [7], chemical vapor reaction (CVR) [8] and pack cementation [9-11]. However, the interfacial cracks and the high cost still limit the application of such coatings.
The properties of cristobalite aluminum phosphate (C-AlPO4) make it a promising candidate material for high temperature applications. These can be summarized as high melting point, low elastic modulus, low oxygen permeability and good erosion resistance. Besides, its thermal expansion coefficient (5.5×10-6/℃) is close to that of SiC ((4.3-5.4)×10-6/℃) and it has good chemical and physical compatibility to SiC, which will result in less defects and oxidation activity sites in matrix [12-13]. On the other hand, hydrothermal electrophore technology was used to produce various coatings because of its numerous advantages such as low cost, high efficiency, and simple operation, in particularly, homogeneous coatings on substrates with complex structures could be easily achieved [14-16]. Moreover, no research about using hydrothermal electrophoretic deposition process to deposit C-AlPO4 coatings, especially on SiC-coated C/C composite surface, was reported.
In this work, C-AlPO4 coatings on SiC-coated C/C composites were prepared by the hydrothermal electrophoretic deposition technique. The influence of deposition voltage on the phase, microstructure and oxidation resistance of the as-prepared coatings was investigated.
2 Experimental
Small specimens (10 mm×10 mm×10 mm) were cut from 2D C/C composites with a density of 1.72 g/cm3. Then, the specimens were hand-polished using SiC paper and ultrasonically cleaned in acetone. After the preparation of the SiC bonding layer by pack cementation process reported in Ref.[17], hydrothermal electrophoretic deposition process was conducted. Firstly, the C-AlPO4 powders were dispersed in isopropanol by a magnetic stirrer for 24 h. Secondly, iodine was added into the suspension solution as a charging agent with an ultrasonic bath for 15 min (ultrasonic power was 200 W), and a later mixing was conducted by a magnetic stirrer for 24 h. Suspension concentration was 20 g/L, and iodine concentration was 0.6 g/L. Thirdly, the as- prepared suspension solution was put into hydrothermal autoclaves. The anode of the autoclaves was graphite substrate (20 mm×10 mm×3 mm); cathode was SiC-coated C/C substrate. After that, the autoclaves were heated to 373 K and held at that temperature until the end of the deposition. During the deposition process, the voltage was kept at 180, 200 and 220 V, respectively. After 45 min deposition, the specimens were taken out from the autoclave and cooled naturally to room temperature. Then, the samples were dried in air at 333 K for 4 h. Finally, the C-AlPO4 coatings were achieved on the SiC-coated C/C substrate.
A JSM–6460 scanning electronic microscope (SEM) was used for the observation of morphologies of the C-AlPO4 coatings. The coating phase structures were analyzed by a Rigaku D/max-3C X-ray diffractometer (XRD). Analytical balance with the precision of 0.1 mg was adopted to measure the mass change of the sample after being deposited for15, 25, 35 and 45 min during the deposition process.
The as-coated specimens were heated at 1 773 K in an electrical furnace to investigate the isothermal and thermal cycling oxidation behavior. Cumulative mass change of the samples after every thermal cycle from high temperature to room temperature was measured by a precision balance and was recorded as a function of time. The mass loss rate (η) was calculated as follows:
×100% (1)
where m0 is the original mass of the coated C/C composites; m1 is the mass of the coated C/C composites after oxidation at high temperature for certain time.
3 Results and discussion
Fig.1 shows the XRD patterns of surface of the as-prepared coatings at different hydrothermal deposition voltages. The peak of C-AlPO4 at 20?-35? can be observed in all the as-prepared coatings, which well accords with the original composition. The peak intensities of the C-AlPO4 phase increase obviously with increasing deposition voltage. The peak intensities of the coating prepared at 180 V show a weak and small characterization. Moreover, fewer peaks of SiC can be observed, inferring that the prepared coating is not perfect and thin, which can be detected by XRD. When the deposition voltage increases to 200 V, peaks of C-AlPO4 phase become sharp and SiC phase disappears. When the deposition voltage increases to 220 V, the strongest peak intensity of the coating is obtained, which indicates that the crystallization of C-AlPO4 crystallites in the as-prepared coatings can be improved with the increase of deposition voltage, this is because the discharge sintering phenomenon between the cathode and anode appears as the deposition voltage increases. In addition, the higher the voltage, the more obvious the sintering, which results in the improvement in crystallization of C-AlPO4 coatings.
Fig.2 shows the SEM images of surface of the as-
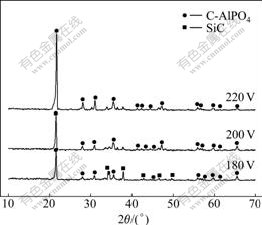
Fig.1 XRD patterns of surface of coatings prepared at different deposition voltages
prepared C-AlPO4 coatings on SiC-pre-coated C/C composites at different hydrothermal deposition voltages. Clearly, the coating surfaces are composed of some crystallites, which are C-AlPO4 crystals by XRD analysis shown in Fig.1. Some microholes on the coating surfaces can be observed. In addition, no cracks on the coating surfaces are found. With the increase in deposition voltage from 180 to 220 V, the surface density of the coatings shows different morphologies. Porous coatings are obtained at 180 V (Fig.2(a)), which coincides with XRD analysis. Inhomogeneous C-AlPO4 coatings are
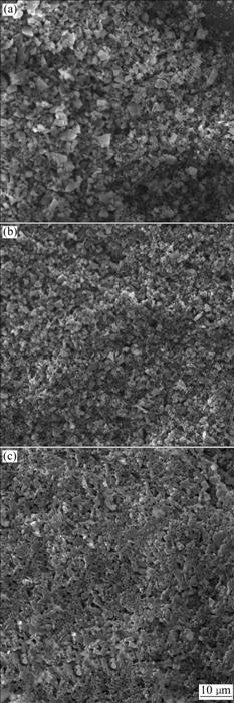
Fig.2 SEM images of surface of C-AlPO4 coatings prepared at different deposition voltages: (a) 180 V; (b) 200 V; (c) 220 V
obtained at 200 V (Fig.2(b)), and very dense and homogeneous C-AlPO4 coatings are achieved at 220 V (Fig.2(c)). The higher deposition voltage will result in stronger discharge sintering between the cathode and anode. Moreover, the higher voltage will also accelerate the diffusion rate of C-AlPO4 crystallites to the C/C-SiC cathode, which is beneficial to obtaining dense and homogeneous C-AlPO4 coatings. The influence of the hydrothermal deposition voltages on the coating morphologies indicates that anticipative structure coatings may be acquired through controlling hydrothermal deposition voltages during hydrothermal electrophoretic process.
Fig.3 reveals the SEM images of cross-section of the prepared C-AlPO4 coatings on SiC-coated C/C composites at different deposition voltages. An obvious two-layer multi-coating is observed. No holes and cracks
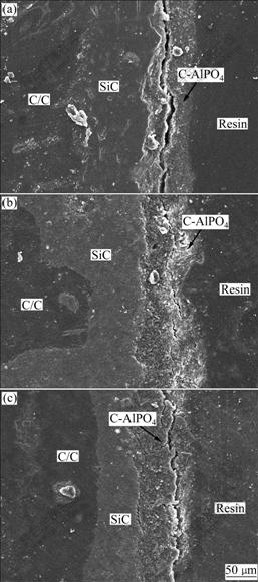
Fig.3 SEM images of cross-section of C-AlPO4 coatings prepared at different deposition voltages: (a) 180 V; (b) 200 V; (c) 220 V
are found due to good match in thermal expansion coefficients between SiC bonding layer and C-AlPO4 layer. The thickness of SiC coatings prepared by pack cementation is about 80 ?m and that of the C-AlPO4 coatings prepared by hydrothermal electrophoretic deposition is 50-60 ?m. At lower deposition voltages, the adhesion strength between C-AlPO4 coatings and substrate is weak; an obvious crack can be observed, and the thickness of the coating is not homogeneous (Fig.3(a)). When the deposition voltage increases to 200 V (Fig.3(b)), the adhesion strength is improved to some extent, but the thickness of the coatings is still not homogeneous. When the deposition temperature reaches 220 V (Fig.3(c)), a large crack at the interface of C-AlPO4 coatings and resin (seal the coated specimens with the resin when preparing cross-section of C-AlPO4 coatings) can be found, which indicates that the adhesion strength of the coating is obviously improved, no visible microcracks and other defects at the interface of C-AlPO4 coatings and substrates are observed, indicating that good adhesion of the coatings to substrates is achieved. From Figs.1-2, it can be concluded that the higher deposition voltage will result in stronger discharge sintering phenomenon between the cathode and anode, which is helpful for obtaining dense, homogeneous and good bonding coatings.
The results of bond strength test between the C-AlPO4 coatings and C/C-SiC substrate at different deposition voltages are shown in Table 1. The as- prepared coating’s bonding strength is respectively 13, 21 and 30 MPa when the deposition voltage is 180, 200 and 220 V. This reveals that the bonding strength of the C-AlPO4 coatings increases with the increase of deposition voltage, which infers that the good bonding C-AlPO4 coatings can be achieved at deposition voltage of 220 V.
Table 1 Results of bonding strength test of coatings deposited at different deposition voltages
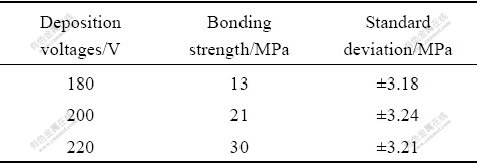
The relationship between deposition amount of C-AlPO4 coatings and deposition voltage is displayed in Fig.4. It is clear that the deposition of C-AlPO4 coatings amount increases with increasing deposition voltage, which is an approximate parabola relationship. The increase of deposition voltage will increase potential energy of the electric field between the two electrodes, which will lead to the acceleration of the charged particles
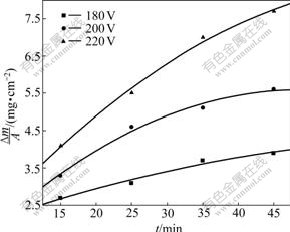
Fig.4 Deposition mass vs deposition time at different deposition voltages
diffusion rate and result in the increase in deposition rate.
According to Ref.[18], the general expression for the thickness of deposited coating is shown as follows:
(2)
where ds(t) is the thickness of the deposited layer, dl is the distance between anode and substrate, e0 is the unit charge, m is the average mass of suspended particles, ρs is the effective density of the deposited layer, σl is the conductivity of the suspension, σs is the conductivity of the deposited layer, U is the applied voltage, and t is the time.
In addition, the distance between the anode and SiC-C/C substrate is much larger than the thickness of the deposited layer, and conductivity of the suspension is larger than that of the deposited layer. Therefore, the following condition is satisfied:
>>
(3)
For this condition, Eq.(2) can be simplified into:
(4)
where A is the total surface of the substrate, z is the average valence of the suspended particles, and ?m(t) is the deposition mass.
From Eq.(4), it can be concluded that the deposition mass per unit area of the coatings and the square root of the deposition time at different hydrothermal voltages satisfy linear relationship, which is also demonstrated by our research shown in Fig.5.
Fig.6 shows the isothermal oxidation curves of the coated C/C composites at 1 773 K. It reveals that the mass loss rate of SiC-coated C/C composites reaches 6.36% after oxidation at 1 773 K for 10 h, which indicates that the single SiC coating cannot effectively protect C/C composites from oxidation for several hours. After the deposition of outer C-AlPO4 coatings, the antioxidation property of the coated C/C composites is obviously improved. And the oxidation resistance of the coated sample increases with the increase of deposition voltage. When deposition voltage is 220 V, the mass loss rate of the prepared multilayer coated C/C sample is only 0.53% after oxidation at 1 773 K for 37 h. Additionally, the sample endures 18 thermalcycles between 1 773 K and room temperature during the oxidation test process; and no cracks are found, inferring that the excellent thermal shock resistance of the as-prepared multilayer coated C/C composites is achieved.
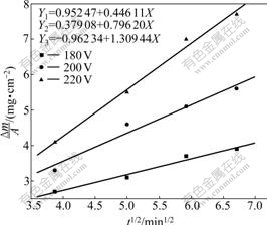
Fig.5 Deposition mass of coatings as function of square root of deposition time at different deposition voltages
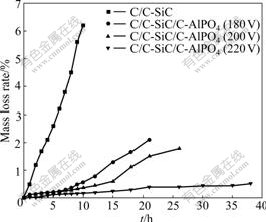
Fig.6 Isothermal oxidation curves of coated C/C composites in air at 1 773 K
4 Conclusions
(1) C-AlPO4 coatings on SiC-coated C/C composites surfaces can be prepared at deposition voltages of 180-220 V by a hydrothermal electrophoretic deposition technique.
(2) The deposition voltage has a major influence on the microstructure of the prepared coatings. The porous coatings are obtained at 180 V, inhomogeneous C-AlPO4 coatings are obtained at 200 V, and dense, homogeneous and good bonding C-AlPO4 coatings can be acquired at deposition voltage of 220 V. The thickness of the as- prepared coatings is 50-60 ?m.
(3) The deposition amount and bonding strength of the C-AlPO4 coatings increase with the increase of deposition voltage. Linear relationship exists between the deposition mass per unit area of the coatings and the square root of the deposition time at different voltages.
(4) The antioxidation property of the coated C/C composites is improved with the increase of deposition voltage. Compared with SiC coating prepared by pack cementation, the multilayer coatings prepared by pack cementation with a later hydrothermal electrophoretic deposition process exhibit better antioxidation property. The as-prepared multi-coatings can effectively protect C/C composites from oxidation in air at 1 773 K for 37 h with a mass loss rate of 0.53%.
References
[1] SHEEHAN J E, BUESKING K W, SULLIVAN B J. Carbon-carbon composites [J]. Annual Review of Materials Science, 1994, 24: 19-44.
[2] TANG Zhong-hua, ZOU Zhi-qiang, XIONG Jie, XU Hui-juan. Fabrication of carbon-carbon composites for aircraft brakes by directional flow thermal gradient chemical vapor infiltration [J]. Journal of Central South University of Technology, 2002, 33(4): 380-386. (in Chinese)
[3] HUANG Jian-feng, LI He-jun, XIONG Xin-bo, ZENG Xie-rong, LI Ke-zhi, FU Ye-wei, HUANG Min. Progress on the oxidation protective coating of carbon-carbon composites [J]. New Carbon Materials, 2005, 20(4): 373-379. (in Chinese)
[4] JANG B P. Oxidation behavior of carbon-carbon composites with/ without CVD ceramic coatings [D]. Atlanta: The Graduate Factuate of Aulurn University, 1995: 92-114.
[5] FU Qian-gang, LI He-jun, SHI Xiao-hong, LI Ke-zhi, WANG Chuang, HUANG Min. Double-layer oxidation protective SiC/glass coatings for carbon/carbon composites [J]. Surface and Coatings Technology, 2006, 200(11): 3473-3477.
[6] LI Guo-dong, XIONG Xiang, HUANG Bai-yun, HUANG Ke-long. Structural characteristics and formation mechanisms of crack-free multilayer TaC/SiC coatings on carbon-carbon composites [J]. Transactions of Nonferrous Metals Society of China, 2008, 18(2): 255-261.
[7] LI Guo-dong, XIONG Xiang, HUANG Bai-yun. Effect of temperature on composition, surface morphology and microstructure of CVD-TaC coating [J]. The Chinese Journal of Nonferrous Metals, 2005, 15(4): 565-571. (in Chinese)
[8] LIU Xing-fang, HUANG Qi-zhong, SU Zhe-an, JIANG Jian-xian. Preparation of SiC coating by chemical vapor reaction [J]. Journal of the Chinese Ceramic Society, 2004, 32(7): 906-910. (in Chinese)
[9] FU Qian-gang, LI He-jun, SHI Xiao-hong, LIAO Xiao-ling, LI Ke-zhi, HUANG Min. Microstructure and anti-oxidation property of CrSi2-SiC coating for carbon/carbon composites [J]. Applied Surface Science, 2006, 252(10): 3475-3480.
[10] HUANG Jian-feng, DENG Fei, XIONG Xin-bo, LI He-jun, LI Ke-zhi, CAO Li-yun, WU Jian-peng. High performance Si-SiC composite coating for C/C composites prepared by a two-step pack cementation process [J]. Advanced Engineering Materials, 2007, 9(4): 322-324.
[11] WANG Biao, LI Ke-zhi, LI He-jun, FU Qian-gang, WANG Xiang, LAN Feng-tao. SiC coating prepared by a two-step technique of pack cementation and CVD on carbon/carbon composites [J]. Journal of Inorganic Materials, 2007, 22(4): 737-741. (in Chinese)
[12] WANG Zhen-jie, YE Da-nian. An investigation of the phase transformation of AlPO4 at high pressure and high temperature [J]. Scientia Geologica Sinica, 1990(3): 287-292. (in Chinese)
[13] WANG Xin-peng, TIAN Shi. Studies of phase transformation, thermal and dielectric properties of aluminum phosphate compounds [J]. Rare Metal Materials and Engineering, 2005, 34(s2): 716-719. (in Chinese)
[14] DENG Fei. Novel hydrothermal electrodeposition technology for preparing oxidation resistant coatings for C/C composites [D]. Xi’an: Shaanxi University of Science and Technology, 2007. (in Chinese)
[15] HUANG Jian-feng, ZHANG Yu-tao, ZENG Xie-rong, CAO Li-yun, DENG Fei, WU Jian-peng. Hydrothermal electrophoretic deposition of yttrium silicate coating on SiC-C/C composites [J]. Materials Technology, 2007, 22(2): 85-87.
[16] HUANG Jian-feng, LIU Miao, WANG Bo, CAO Li-yun, XIA Chang-kui, WU Jian-peng. SiCn/SiC oxidation protective coating for carbon/carbon composites [J]. Carbon, 2009, 47(4): 1198-1201.
[17] HUANG Jian-feng, ZENG Xie-rong, LI He-jun, XIONG Xin-bo, FU Ye-wei. Influence of the preparing temperature on phase, microstructure and antioxidation property of SiC coating for C/C composites [J]. Carbon, 2004, 42(8/9): 1517-1521.
[18] ARGIRUSIS C, DAMJANOVIC T, STOJANOVIC M, BORCHARDT G. Synthesis and electrophoretic deposition of an yttrium silicate coating system for oxidation protection of C/C-Si-SiC composites [J]. Materials Science Forum, 2005, 494: 451-456.
Foundation item: Project(50772063) supported by the National Natural Science Foundation of China; Project(NCET-06-0893) supported by the Foundation of New Century Excellent Talent in University of China; Project(20070708001) supported by the Doctorate Foundation of Ministry of Education of China; Project(SJ08-ZT05) supported by the Natural Science Foundation of Shaanxi Province, China; Project supported by the Graduate Innovation Fund of Shaanxi University of Science and Technology, China
Received date: 2009-06-15; Accepted date: 2009-10-28
Corresponding author: HUANG Jian-feng, PhD, Professor; Tel: +86-29-86168803; E-mail: huangjf@sust.edu.cn
(Edited by CHEN Wei-ping)