
3D FEA simulation of 4A11 piston skirt isothermal forging process
DONG Wan-peng(董万鹏), CHEN Jun(陈 军)
Department of Plasticity Engineering, Shanghai Jiao Tong University, Shanghai 200030, China
Received 22 October 2007; accepted 26 May 2008
Abstract: To eliminate the defects during piston skirt isothermal forming process, simulations under different process parameters such as the deformation temperature and friction factor were analyzed with the rigid-plastic FEA. Deformation pattern, metal flow and influence of process parameters were concluded. The prediction load value with a relative error of 4.98% is more accurate to the testing one than that from the empirical formula whose relative error is up to 50.8%. Finally, based on the simulation results, an improved process at 300 ℃ and 0.005-0.05 s-1 was verified without any defects by the physical try-out.
Key words: finite element analysis(FEA); piston skirt; isothermal forming; internal combustion engines
1 Introduction
A piston skirt, as shown in Fig.1, is one of the important components of internal combustion engines (ICE). As ICE will be run in higher speed, larger pressure and heavier load, it results in more burdening working conditions for piston skirts[1]. Consequently, it is necessary to improve the design and manufacturing process of piston skirt. Recently, light-mass design has been widely spread in metal forming[2-4]. According to this concept, studies on aluminum alloy instead of steel to be the main material of piston skirt have attracted more and more research interests, which may benefit energy conservation and environmental protection. Another technical renovation is about manufacturing process. Forging is adopted to substitute for casting to gain a better metal structure quality. Isothermal forging is a metal forming process developed since 1960s, in which dies should be heated and kept at the same temperature as the billet during the whole process[5-6]. This technology may improve the homogeneity of metal flow, enhance the plasticity and decrease the deformation pressure, which is important to shape the complex surface with accurate dimensions. Therefore, it is proposed to trial-produce an aluminum piston skirt by isothermal forging. Due to lack of experience about how to integrate these technologies, it is critical to further research thoroughly and urgently.
SONG et al[7-8] experimentally studied the influence of the deformation condition on the isothermal forming for type 240 aluminum piston skirt. YOU and MA[9] discussed the isothermal extrusion process of 4A11 alloy piston skirt. Using CAE (computer aided engineering) technology, ZHOU et al[10-11] analyzed the deformation load and relative effects by different billet profiles during forging process of LD11 aluminum alloy.
In the present work, 3D FEA simulations were carried out under different process parameters such as deformation temperatures and interfacial friction factors based on commercial FEA code, DEFORM 3D V6.0.
2 Simulation
2.1 Piston skirt isothermal forming process
A piston skirt has a complex inner face which consists of many non-machining slips and a rather large volume. The forging ratio is up to 75%. The metal flows tempestuously in die cavities, which might result in defects or die failures if the process parameters were not set up properly. The isothermal forming process maintains a constant temperature and slow strain rate, which can effectively improve the homogeneity of metal flow and decrease the deformation pressure on the material. Experimental studies showed that the isothermal forming process may prevent many forging defects, for instance, eccentricity, sticking to die and un- filled pores, which are common in the hot forging process[6].
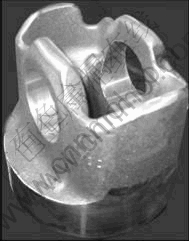
Fig.1 Photograph of piston skirt after machining
Isothermal forming process adopts a closed isothermal forging[12], as shown in Fig.2, and the motion pattern is like back extrusion. Through adjusting the punch velocity, the forming temperature can be kept relatively as a constant. The billet is heated up through resistant heating while the die uses an internal heating system that can control the temperature automatically.
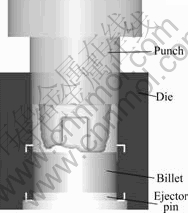
Fig.2 Schematic diagram of tooling assembly of forging process
2.2 Methodologies
A half of the billet was modeled for symmetry. The symmetry face was predefined as a boundary condition. In process simulations, the billet was defined as a rigid-plastic object while the punch and the die were considered to be rigid. According to traditional experience, the parameters used are listed in Table 1.
Table 1 Simulation parameter
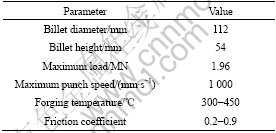
Aluminum alloy 4A11 was adopted in this process and it is assumed to be isotropic. The stress—strain curves are shown in Fig.3. Based on the experiments of existing products, there were two forging temperatures to compare, whose data were from the technical document of factory through their previous material testing.
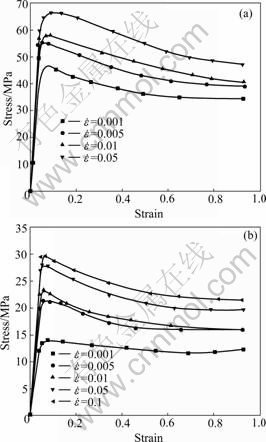
Fig.3 Stress vs strain curves with different strain rates: (a) 300 ℃; (b) 450 ℃
Limited by the plant equipments, we are required to analyze two ranges of punch velocity; one was smaller than 5 mm/s, and the other was 5-50 mm/s. And the average value of the effective strain rate was approximately calculated by[13]

where v is the initial velocity of the punch, and h is the maximum height of the workpiece.
Considering the complex of lubricant, we used two types of friction models, coulomb friction and shear friction to computer simulations on software DEFORM. Coulomb friction is used when contact occurs between two elastically deformed objects, or between an elastic object and a rigid object, generally to model sheet forming processes, while constant shear friction is used mostly for bulk-forming simulations. The frictional force in the constant shear model is defined by[14]
fs=mk (2)
where fs is the frictional stress, k is the shear yield stress and m is the friction factor. The typical values of m range from 0.2 to 0.9 as suggested in Ref.[13], generally, 0.2-0.3 for lubricated hot forming processes, and 0.7- 0.9 for un-lubricated surfaces.
In this study, the constant shear law was used to model the lubricant condition between billets and die during isothermal forging process.
3 Results and discussion
3.1 Metal flow pattern
As can be seen from Fig.4, there are two stagnant zones of the billet in the forging process. One is located around the upper end face which contacts with the punch, and the other is adjacent to the bottom which contacts with the lower die. Between these two zones, there is a zone with severe deformation, where the metal flows outward and upward to shape the piston skirt. It flows almost as the pattern of a back-forward extrusion[15]. The zone with the greatest deformation lies in the inner surface at the beginning but turns up both in the inner surface and the upper end face by the end. There is a local deformation of upset pattern occurring close to the thick wall during die closure. Furthermore, the extruded metal translates upward with no deformation until it reaches the stepped face of the punch. Because of the non-equal-thickness of the shaped piston skirt, Fig.4 also shows that the thick side has the quickest metal flow, and such phenomenon become more and more notable as the deformation increases.
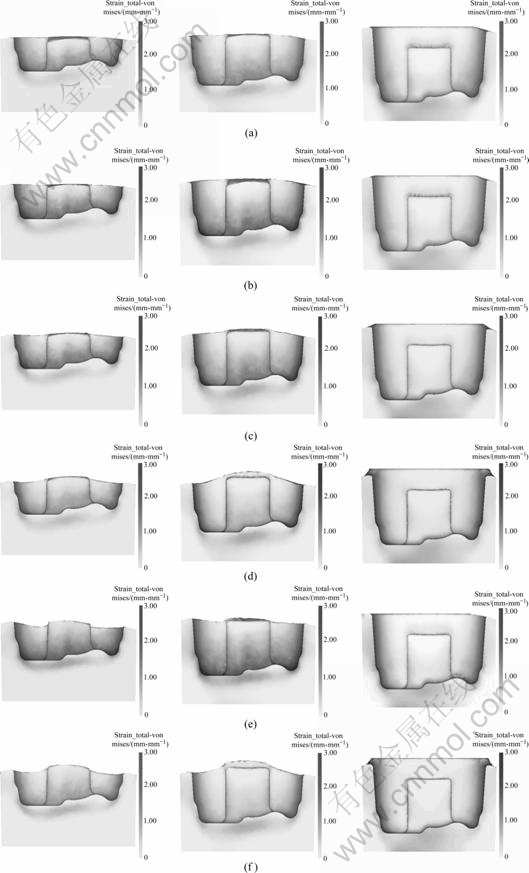
Fig.4 Effective strain distribution with different process parameters: (a) t=300 ℃; m=0.2,
<0.05; (b) t=300 ℃, m=0.2,
=0.1-1.0; (c) t=300℃, m=0.4,
<0.05; (d) t=300 ℃, m=0.9,
<0.05; (e) t=450 ℃, m=0.2,
=0.1-1.0; (f) t=450 ℃, m=0.9,
<0.05
At the end of forming process, the metal flow brings a jump of the forming load, as shown in Fig.5. This jump is due to the final die closure, where the cavity was filled up (without considering the residual air) and there was no more space for the metal to flow. Forging process became a closed compression process, as a result, the load increases sharply.
3.2 Effects of process parameters
Forging temperature is the primary factor affecting metal flow stress. Fig.4 ((b) vs (e) and (d) vs (f)) shows the effect of the temperature on metal flow at a certain time step. Fig.5 represents the influence of forging temperature on the load prediction. In the whole forging process, the billet experienced extraordinarily uneven deformation. The state variable of effective strain presented details about the accumulated deformation of billet at a certain time step. Lower temperature improved the homogeneity of metal flow at a higher flow stress level, and higher temperature brought more active metal motions, which enhanced the plasticity subsequently. Thus, at a certain deformation, the load decreased as the forging temperature increased with heating time and energy consumption.
Due to interfacial friction and non-uniform cross sections of skirt, the metal flow ispostponed in the thin wall area. Larger friction factor indicates faulty lubrication. Fig.4 shows that the faulty lubrication does lots of harm to the homogeneity of metal flow. And it also made the forging load increase, and energy consumption as well. As shown in Fig.5, together with a complex inner surface, the metal flow velocity to the upper end face varies so much that a bulge is formed which might result in fold or under filling. Fig.6 indicates the under filling in the thin wall area.
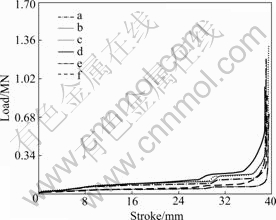
Fig.5 Load prediction with different process parameters: (a) t=300 ℃; m=0.2,
<0.05; (b) t=300 ℃, m=0.2,
=0.1- 1.0; (c) t=300℃, m=0.4,
<0.05; (d) t=300 ℃, m=0.9,
<0.05; (e) t=450 ℃, m=0.2,
=0.1-1.0; (f) t=450 ℃, m=0.9,
<0.05
Under a certain temperature and deformation, the forging load increases with the increases of punch velocity. With the increases of strain rate, flow stress gets larger; accordingly, the load becomes greater.
The whole forging process is analyzed by numerical simulations prior to the shop trials. Design improvements are used to balance the metal deformation.
3.3 Load prediction
During conventional engineering calculation, empirical equations are employed to estimate the forming load. The modified formula[16] is used to obtain the pressure with taking a flow pattern as back-forward extrusion with shear law:

where σs is the yield stress of billet material, m is the friction factor, d0 is the diameter of an initial billet, and d1 is the diameter of the deformed one.
Thus, the forming load was calculated to be 22.62 MN, which is much larger than the testing value (15 MN). The relative error is up to 50.8%. Fig.5 presents the instantaneous load prediction with the respect to the stroke increment (t=300 ℃, m=0.2). The maximum of load reaches 14.252 7 MN, which is smaller than that of actual measurement. And the relative error of simulation prediction vs actual measurement is just 4.98%. Because of the complex interfacial contact condition, the friction factor varies much even in a single forming process with one type of lubricant. It is a general law that increasing friction not only blocks the metal flow but also causes the metal to be unevenly deformed. In order to shape the workpiece, the forming load is set up to be greater with an extended forming stroke.
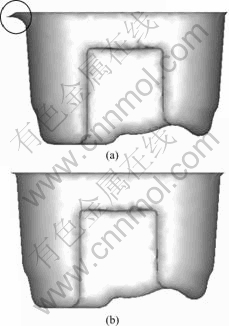
Fig.6 Unfilling in thin wall area: (a) Initial design; (b) Modified design
4 Conclusions
1) The simulation results demonstrate that it is a feasible way to improve the conventional hot forming process at t=300℃, ε=0.005-0.05 s-1 with isothermal forming technology.
2) The distribution of deformation is revealed by metal flow pattern on computers. And the influence of forming conditions was also studied without any cost before a workshop trial.
Acknowledgments
The authors would like to thank Mr. ZHU Li-min and his associates at Shandong Binzhou Bohai Piston Group Corporation for the experimental data.
References
[1] ZHU Li-min, LUO Da-chun. Lower loose core casting process of aluminum piston [J]. Special Casting and Nonferrous Metal, 1993(2): 35-37. (in Chinese)
[2] CHOI H, KOC M, NI J. A study on the analytical modeling for warm hydro-mechanical deep drawing of lightweight materials [J]. International Journal of Machine Tools and Manufacture, 2007, 47(11): 1752-1766.
[3] CUI Xin-tao, WANG Shu-xin, HU S J. A method for optimal design of automotive body assembly using multi-material construction [J]. Materials & Design, 2008, 29(2): 381-387.
[4] SHI Yu-liang, ZHU Ping, SHEN Li-bing, LIN Zhong-qin. Lightweight design study of automotive front rail with TWB structure [J]. Chinese Journal of Mechanical Engineering, 2008, 19(3): 374-377. (in Chinese)
[5] SHAN D B, WANG Z, YAN L, LU Y, XUE K M. Study on isothermal precision forging technology for a cylindrical aluminum-alloy housing [J]. Journal of Materials Processing Technology, 1997, 72: 403-406.
[6] RUSZ S, SINCZSK J, LAPKOWSKI W. Isothermal plastic forming of high-carbon steel [J]. Materials Science and Engineering A, 1997, 234/236: 430-433.
[7] SONG Zhong-ming. Research on LD11 piston skirt isothermal forming extrusion experiments [J]. Journal of Locomotive Engineering, 2000(4): 41-46. (in Chinese)
[8] SONG Zhong-ming, YOU He-qing. The influence of the deformation condition on the isothermal forming for the type 240 aluminum piston skirt [J]. Foundry Technology, 2001(6): 33-34. (in Chinese)
[9] YOU He-qing, MA Qin. Isothermal extrusion process of 4A11 alloy piston skirt [J]. Hot Working Technology, 2007, 36(5): 65-68. (in Chinese)
[10] ZHOU Fei, PENG Ying-hong, RUAN Xue-yu. Influence of deformation load on forging process of aluminum piston skirt [J]. The Chinese Journal of Nonferrous Metals, 2000, 10(S1): 23-27. (in Chinese)
[11] ZHOU Fei, PENG Ying-hong, LEI Jun, RUAN Xue-yu. Billets study during piston skirt forging process of LD11 aluminum alloy [J]. Journal of Shanghai Jiaotong University, 2001, 35(1): 86-89. (in Chinese)
[12] HUANG Z H. Workability and microstructure evolution of Ti-47Al-2Cr-1Nb alloy during isothermal deformation [J]. Intermetallics, 2005, 13(3/4): 245-250.
[13] DEFORM-3D Version 6.0 User’s Manual [M]. Columbia, Ohio: Scientific Forming Technologies Corporation, 2006.
[14] ZHAO Zhen-zhe, SHAO Ming-zhi. Friction and lubrication in metal forming [M]. Beijing: Chemical Industry Press, 2004. (in Chinese)
[15] OSAKADA K, MATSUMOTO R, OTSU M, HANAMI S. Precision extrusion methods with double axis servo-press using counter pressure [J]. CIRP Annals-Manufacturing Technology,2005, 54(1): 245-248.
[16] CUI Ling-jiang, HAN Fei. Plastic forming technology [M]. Beijing: Mechanical Industry Press, 2007. (in Chinese)
Corresponding author: DONG Wan-peng; Tel: +86-21-62824255; E-mail: dong@shenmogroup.com
(Edited by LI Xiang-qun)