Trans. Nonferrous Met. Soc. China 20(2010) s973-s980

Reverse design of microstructure and application effectiveness for semi-solid processing of aluminum alloy
YANG Yi-tao(杨弋涛)1, YIN Xiang-lin(尹湘林)1, SUN Bao-liang(孙保良)2, ZHANG Heng-hua(张恒华)1,
JIN Xiao-chun(金晓春)2, SHAO Guang-jie(邵光杰)1
1. School of Materials Science and Engineering, Shanghai University, Shanghai 200072, China
2. Shanghai Huizhong Automotive Manufacturing Co. Ltd., Shanghai 200122, China
Received 13 July 2010; accepted 15 July 2010
Abstract:The hypoeutectic Al-Si alloy billet with non-dendrite was reheated to meet the needs of the semi-solid thixoforming microstructure by four kinds of reheating power, achieving the same final temperature of 851 K. Subsequently, under the same condition of thixoforming, the microstructure, surface hardness and tensile properties were observed. Afterwards, quantitative analysis was made for the microstructures of the reheated semi-solid of billet and the thixoforming parts. The results show that when the induction reheating power is 90 kW, the average grain size of the semi-solid billet is the minimum, the microstructures of the thixoforming samples also are the finest, and the mechanical properties of the relevant thixoforming samples are the best. Furthermore, after studying on the relationship between the microstructures of the semi-solid billet of aluminum alloy and the mechanical properties of the thixoforming samples, the reverse design of microstructure is primarily achieved. Finally, the effectiveness of the reverse design for semi-solid microstructure is confirmed by an actual automobile part with complex shape.
Keywords: Al-Si alloy; semi-solid; microstructure; reheating; reverse design
1 Introduction
It is an important method to improve the material properties by the effectual control of structure morphology and grain size during the material forming process[1]. The semi-solid process effectively combines the good formability of liquid metal and the high forming quality of solid metal so as to manufacture some mechanical parts with fine microstructure and properties[2-4]. The semi-solid thixoforming technology of aluminum alloy mainly includes the preparation of non-dentrite material, the remelting of non-dentrite material and the semi-solid die-casting technology, among which the material remelting plays the key role of linking the preceeding with the following in the semi-solid forming, for the purpose of quickly and precisely obtaining the solid fraction and the microstructure of grain with certain appearance[5-6]. Different semi-solid microstructures appear under different reheating processes, which affect the afterwards semi-solid die-casting remarkably[7-8]. Some research progresses, which have been gotten about the semi-solid process of different Al alloys[10-11], show that reheating makes round grains in semi-solid micro structure and appropriate reheating time favor the formation of near global grains, but reasonable determination of grain micro structure do not realize yet in semi-solid process. Therefore, the effect of semi-solid microstructure on the microstructures and properties of die-castings after induction reheating is analyzed quantitatively, which finally provides effective thought for the reverse design of remelting semi-solid microstructure from the needs of microstructures and properties.
2 Experimental
The chemical composition of experimental material that was non-dentrite hypoeutectic aluminum alloy was shown in Table 1. The experimental billets were prepared by electromagnetic continuous casting technology, during which the primary material was made to be round-bar shaped non-dentrite billet with 72 mm diameter, and then to be cut short with length of 140 mm. During the continuative reheating process, the materials were heated to semi-solid state in the rotary induction heating apparatus with median frequency power. The heating power should be controlled firstly high and then low to ensure the uniform temperature. In the final thixoforming, the heated billets were pressed into cavity by the horizontal cold-chamber die-cast machine with working pressure of 500-5000 kN.
Table 1 Composition of experimental aluminum alloy (mass fraction, %)
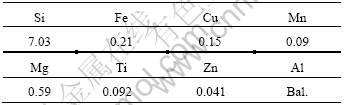
The prepared billets by continuous casting was put in he induction heating apparatus and heated under four kinds of powers, respectively, with the final temperature of the core of 851K. When the heating powers were 60, 75, 90 and 105 kW, the heating time in each working location should be 51.5, 36.1, 26.1 and 20.2 s, respectively. In order to compare the microstructures and mechanical properties of the thixoforming samples under different heating powers in a lateral direction, the processing parameters were kept unchanged during the thixoforming.
The quenched billet in water was intercepted from the top, middle and bottom position to form metallographic specimens, as shown in Fig.1(a). The metallographic observation positions of the thixoforming sample is shown in Fig.1(b), in which the tensile specimens are cut by linear cutting machine and the scraps are used for metallographic observation and hardness test.
The metallographic observation in position A of Fig.1(b) is carried out in its cross-section. The microstructures of different vision fields were taken photos at random by metallographic microscope. Quantitative analysis for the α(Al) grain size, the roundness and solid fraction was made by the image analysis software. The computational method for the average grain size was extracting a diameter through the centroidal of the primary α(Al) phase grain every 2° in the two-dimensional image, and then calculating the average value of all data as the mean diameter of α(Al) grain. The roundness could be calculated by the formula Fc=P2/4pA (In the formula, P and A meant perimeter and area of the primary α(Al) phase grain respectively)[12], which mean the circular when the value was one. And the bigger the value is, the further away the grain from the circular is, while the solid fraction is estimated by measuring the proportion the primary α(Al) phase grain taking up. The surface hardness (Brinell) of position A in Fig.1(b) was measured for comparison in different conditions.
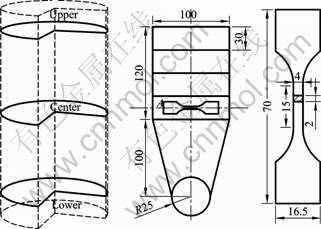
Fig.1 Schematic diagram sampling location: (a) Billet; (b) Stepped sample; (c) Tensile sample (Unit: mm)
3 Results and discussion
The microstructures in the core of the semi-solid billets heated under different powers are shown is Fig.2. Among that, the grey part is the primary α(Al) phase and the dark part is the eutectic structure. The quantitative analysis for the average grain size, the roundness and solid fraction are shown in Fig.3.
This shows that the reheating time gets shorter with the higher induction heating power, and the average grain size of the semi-solid billet becomes smaller finally. The average grain size is 54.2 μm under heating power of 90 kW, which is decreased by 35.3% compared with the one under power of 60 kW while they both meet the microstructure requirements of the semi-solid thixoforming with the roundness and solid fraction of fewer differences. But the average grain size under heating power of 105 kW gets slightly bigger and reaches 55.9 μm, roundness of 1.46 and solid fraction of 65.2% make against thixoforming. The roundnesses of 1.35 and 1.36 are separately obtained under powers of 60 kW and 90 kW. Generally speaking, in a certain range of semi-solid structure solid fraction, it is better for the later thixoforming with smaller grain and roundness.
There are thixoforming tests for the semi-solid billets reheated at 60, 75, 90 and 105 kW under the same die-casting condition. The microstructures in the crossing-section of the position A as shown in Fig.1 (b) before and after heat treatment were observed, and their results are partially shown in Fig.4. The heat treatments orderly carried in solid solution at 813 K for 2 h and in solid solution at 443 K for 2 h. Because the structures before and after heat treatment are quite similar, the quantitative analysis for their average grain size and roundness in as-cast state are carried out and their results are shown in Fig.5.
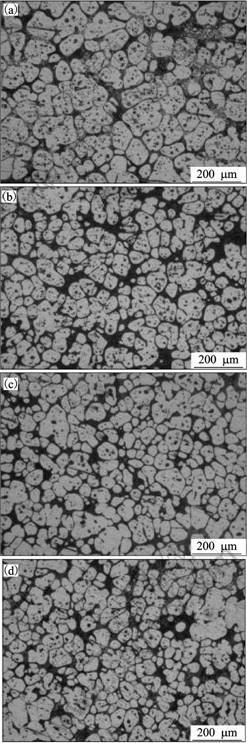
Fig.2 Microstructures of semi-solid material after induction reheating under different powers: (a) 60 kW; (b) 75 kW; (c) 90 kW; (d) 105 kW
The average grain size and roundness in the microstructures of semi-solid die-castings also decrease with the increase of induction heating power. The average grain size and roundness of thixoforming samples heated under 105 kW are larger than that in 90 kW. This is because the average grain size and roundness before die-casting for 105 kW induction heating power is larger than that of 90 kW.
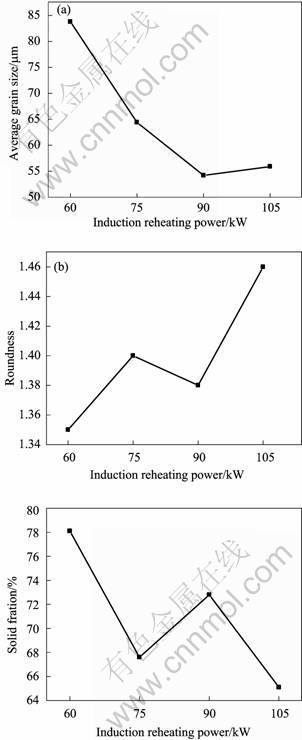
Fig.3 Quantitative analysis of billet microstructures after reheating: (a) Average grain size; (b) Roundness; (c) Solid fraction
It is concluded from the comparison between the semi-solid microstructures before and after the thixoforming,these changes of structures show the same tendency at different heating powers and some structure transmissibility from billet to die-casting, which means that the smaller the grain of the semi-solid reheated billet is, the smaller the grain of the sample after thixoforming is, thus it is desirable to obtain fine mechanical properties.
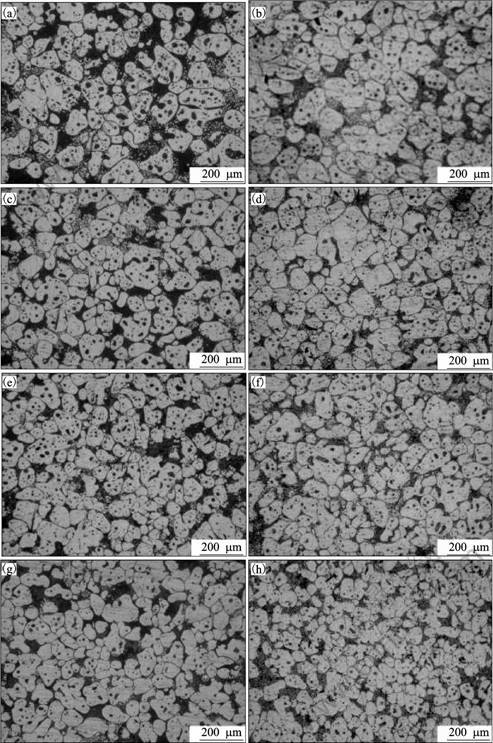
Fig.4 Microstructures of thixoforming sample in part A under different reheating powers: (a) As-cast, 60 kW; (b) Heat treatment, 65 kW; (c) As-cast, 75 kW; (d) Heat treatment, 75 kW; (e) As-cast, 90 kW; (f) Heat treatment, 90 kW; (g) As-cast, 105 kW; (h) Heat treatment, 105 kW
In order to analyze the relationships between grain sizes and reheating powers before and after die-casting, the exponential fitting results of quantitative analysis to the microstructure as shown in Fig.3 (a) and Fig.5 (b) are shown in Fig.6.
Under the different induction powers and the same die-casting condition, the mechanical properties of die-castings before and after heat treatment are measured and shown in Fig.7. This shows that the tensile strength of as-cast die-casting in 105 kW induction reheating power reaches the maximal value of 282 MPa, but its elongation and hardness are relatively low. Its reasons are that the grain size and roundness existing in semi-solid billets before die-casting and die-castings after die-casting are lager. The tensile strength, elongation and hardness of as-cast die-casting in 90kW induction reheating power are 266.2 MPa, 12.2% and 81HBS, respectively, which are increased by 3.5%, 50% and 3.3%, respectively, compared with the ones under power of 60 kW.
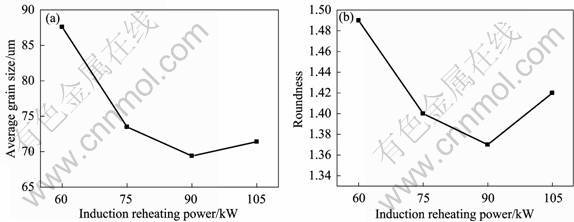
Fig.5 Quantitative analysis of microstructure in as-cast thixoforming samples: (a) Average grain size; (b)Roundness
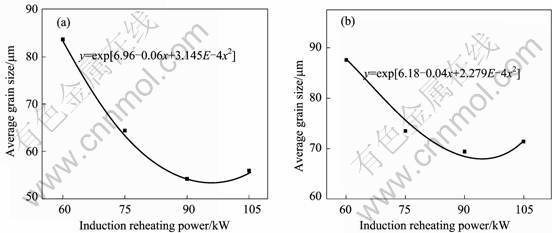
Fig.6 Fitting curve of microstructure change: (a) Before die-casting; (b) After die-casting
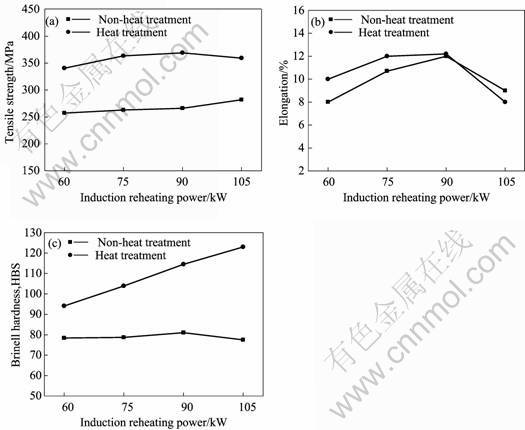
Fig.7 Comparison of mechanical properties of ladder samples: (a) Tensile strength; (b) Elongation; (c) Hardness
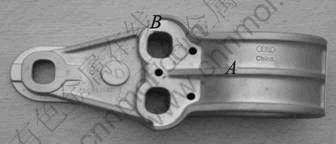
Fig.8 Locations for hardness and metallographic observation in rear supporting part
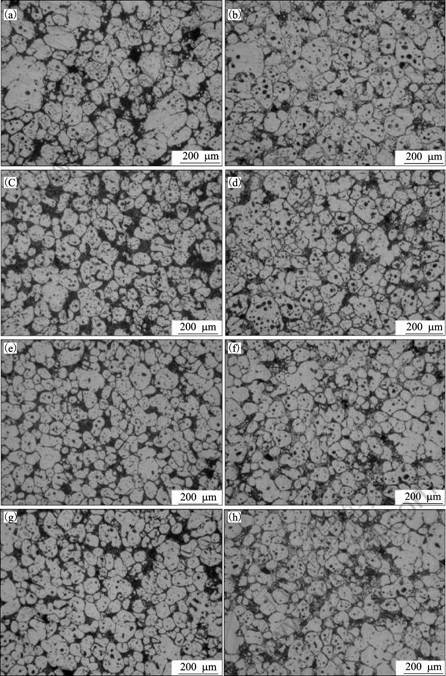
Fig.9 Microstructures of thixoforming sample under different reheating powers: (a) Position A, 60 kW; (b) Position B, 60 kW; (c) Position A, 75 kW; (d) Position B, 75 kW; (e) Position A, 90 kW; (f) Position B, 90 kW; (g) Position A, 105 kW; (h) Position B, 105 kW
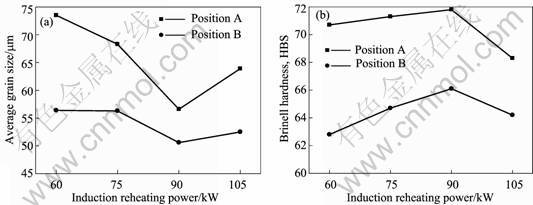
Fig.10 Changes of average grain size and Brinell hardness with induction reheating power: (a)Average grain size; (b)Hardness
Therefore, the process time shortening and mechanical properties improvement of die-castings appear with the increase of induction heating power. Simultaneously, reasonable heat treatment can obviously improve the mechanical properties of die-castings. Total properties of die-castings in 90kW induction reheating power of billet are optimal.
The above researches told us that the same non-dentrite billets of aluminum alloy can obtain some semi-solid die-castings with different structures and mechanical properties under varied reheating technologies. Therefore, some basis for the adequate manufacture method of semi-solid process was introduced to meet the needs of process optimization and cost saving, and reverse design thought of structure from the requirement of applied properties was established. In order to verify the rationality of this reverse design thought in microstructure, the study chose rear supporting part of automobile for verification test as shown in Fig.8, the locations of metallographic observation and hardness measure are positions A and B of this die-casting in Fig.8.
The observed results as shown in Fig.9 are taken quantitative statistics, and their results are shown in Fig.10. Under 90 kW induction heating power, the grain of die-castings is finest in all conditions, its size in positions A and B are 56.6 μm and 50.6 μm, respectively. Accordingly, the hardnesses in position A and B reach maximal values 71.8HBS and 66.1HBS. Compared with the results in stepped samples, the change tendency of grain size in die-castings also inherits that in billets before die-casting. Appropriate choice of reheating process conduced to gain optimum semi-solid microstructure before die-casting and furthermore forming microstructure after die-casting. Therefore, some parts with optimum mechanical properties can be manufactured and the reverse design of die-casting microstructure became reality.
4 Conclusions
1) During billet reheating process, the higher the electromagnetic induction heating power is, the shorter the time is, the smaller the grain and the roundness are, which provides high-quality semi-solid billet for the afterwards thixoforming at power of 90 kW, minimal grain diameter of 54.2 μm and roundness of 1.36.
2) The microstructures of thixoforming samples heated under different powers inherit the microstructures of semi-solid billets before thixoforming. The smaller the microstructure before thixoforming is, the smaller the microstructure after thixoforming is.
3) Appropriate choice of reheating process is conduced to gain optimum semi-solid microstructure before die-casting and furthermore forming microstructure after die-casting. Therefore, the reverse design of die-casting microstructure becomes reality.
4) Among the three essential processes of semi-solid forming, it is allowable to make a reverse design of the reheating semi-solid microstructure according to the final microstructure, which realizes the goal of improving production efficiency and saves the cost.
References
[1] FLEMINGS M C. Behavior of metal alloys in the semi-solid state [J]. Metallurgical Transactions A,1991, 22(5): 957-981
[2] YANG Bi-cheng, TIAN Zhan-feng, ZHU Xue-xin, ZHANG Zhi-feng, XIE Li-jun, XU Jun. Investigation on the thixoforming process of the advanced semi-solid aluminum alloy [J]. Foundry, 2005,54(5): 475-478
[3] YANG Yi-tao, SHAO Guang-jie, ZHANG Heng-hua, XU Luo-ping. Decennial survey on foundation and application of semi-solid processing [J]. Special Casting & Nonferrous Alloys, 2008 ,28 (5): 367-372
[4] KANG C G, SEO P K. The effect of gate on the filling limitation in the semi-solid forging process and the mechanical properties of the products[J]. Materials Processing Technology, 2003, 135: 144-157.
[5] YANG Yi-tao, WANG Jian-fu, ZHANG Heng-hua, SHAO Guang-jie. The microstructure evolvement and simulation analysis during semi-solid processing of aluminum alloy [J]. Solid State Phenomena, 2008, 141-143: 139-144.
[6] YANG Yi-tao, WANG Jian-fu, ZHANG Heng-hua, SHAO Guang-jie, Mensuration and simulation of mold filling process in semi-solid die-cast of aluminum alloy [J]. Transactions of Nonferrous Metals Society of China, 2006, 16(s): 1402-1406
[7] YIN Xiang-lin, YANG Yi-tao, SHAO Yu-peng, SHAO Guang-jie. Investigation of the relationships between billet reheating and semi-solid die-casting [J]. Advanced Materials Research, 2010, 97/101: 306-310
[8] ZOQUI E J, PAES M, ROBERT M H. Rheological Behavior and Microstructure Evolution of Semi-Solid A356 Alloy Produced by Different Routes [C]//. Proceedings of the 9th International Conference on Semi-Solid Processing of Alloys and Composites S2P 2006, Busan, Korea, 2006: 565-568
[9] KANG C G, JUNG H K, JUNG K W. Thixoforming of an aluminum component with a die designed by process simulation [J]. Journal of Materials Processing Technology, 2001, 111: 37-41
[10] MORADI M, NILI-AHMADABADI M, HEIDARIAN M, PARSA M H. Study of ECAP processing routes on semi-solid microstructure evolution of A356 alloy [J]. Solid State Phenomena, 2008, 141-143: 397-402.
[11] KHALIFA W, TSUNEKAWA Y, OKUMIYA M. Effect of reheating to the semisolid state on the microstructure of A356 aluminum alloy produces by ultrasonic melt-treatment [J]. Solid State Phenomena, 2008, 141-143: 499-504.
[12] LI Tao. Microstructure Evolvement of semi-solid metal and its real-time observation [D]. Northwest Institute of Technology. 2003
(Edited by LI Yan-hong)
Corresponding author: YANG Yi-tao; Tel: +86-21-56334465; E-mail: yyt@staff.shu.edu.cn