
TEM microstructure of mechanically alloyed Ti-12Mg powders
T. S. KIM1, B. T. LEE2, J. P. AHN3, J. K. PARK3, J. C. BAE1
1. Nanomaterials Team, Korea Institute of Industrial Technology, Techno-Park Songdo, Incheon 406-130, Korea;
2. School of Advanced Materials Engineering, Kongju National University, Kongju, Chungnam 314-701, Korea;
3. Nano-materials Research Center, Korea Institute of Science and Technology, Seoul 136-791, Korea
Received 20 April 2006; accepted 30 June 2006
Abstract: The microstructures of mechanical alloyed(MA) Ti-12%Mg alloy powders were examined using a high resolution TEM (HRTEM). The effect of MA atmospheres such as argon gas and liquid isopropyl alcohol on the resultant microstructure was investigated. Both the MA powders form a homogeneous Ti-Mg solid solution, but the oxidation behavior is distinguished. The phase change was studied as a function of milling conditions and annealing temperatures.
Key words: Ti-Mg alloy; mechanical alloying; nanostructure; HRTEM
1 Introduction
Ti alloys have been widely used in various industries such as automobile and aerospace, due to their low density, high specific strength, and excellent creep and corrosion resistance. Although much attention has been paid on alloying with light element of Mg[1,2], many difficulties have been reported to fabricate the Ti-Mg system. It is attributed to no forming any kind of metallic compound, extremely low boiling point of Mg compared to Ti, and mutually low solubility of about 1.6% Mg(mole fraction), the same below in Ti at 765 ℃ and 0.12% Ti in Mg at 654 ℃[1-3].
In order to synthesize the Ti-Mg alloy, mechanical alloying (MA) was reported to be an alternative due to not only its relatively easy operation and low cost, but also an effect of grain refining and solubility extension[4]. The refined microstructure (and hence short diffusion distances) and the lattice defects (vacancies, dislocations, grain boundaries, etc) occurred during MA allow inter-diffusion at sufficiently low temperatures, sometimes even at room temperature, leading to the formation of homogeneous alloys[5]. It has been shown that MA can synthesize many types of materials such as oxide-dispersion strengthened (ODS) superalloys, intermetallics, amorphous alloys, nanostructured materials, and a variety of other non-equilibrium phases[6-8].
Although some attempts have been made to alloy Mg with Ti using MA, full satisfaction was not obtained due to atmospheric contamination during the mechanical alloying[2]. It is due to high potential of Ti and Mg to react with oxygen, resulting in the formation of magnesium oxide. In addition, the detailed examination on the nanostructure in Ti-Mg system has not been well understood so far.
In this work, the Ti-12%Mg alloy was prepared by MA process using Ti and Mg powders under Ar atmospheres(dry condition, referred as PD) and in isopropyl alcohol (wet condition, referred as PW). The microstructural features as well as oxidation behavior of the powders during milling and the processes were studied using XRD, SEM and HRTEM.
2 Experimental
100 g commercial Ti and Mg powders with particle size in the range of 400-500 mm and 10-50 mm, respectively, were blended to make Ti-12%Mg mixture. The blended powders were mechanically alloyed in a cylindrical hardened steel vial of 100 mm in diameter and 150 mm in length with ZrO2 balls of 6mm in diameter. The ball-to-powder ratio in mass was 20∶1. In order to identify an effect of the atmosphere on the mate rials, the alloying was carried out under argon atmosphere(PD) as well as in isopropyl alcohol(PW), a processing control agent, for 60 h. The optimum rotational speed of the mill was based on the calculation of the critical mill rotational speed, Nc, obtained from the equation Nc = 76.6D-1/2, where D is the mill diameter in feet. Since the optimum speed is usually less than the critical speed, calculated to be about 145 r/min, the optimum speed was set at 130 r/min.
The MA powder was heat treated at 300 and 700 ℃. SEM (JSM 6335F) and TEM (JEM 2010) equipped with energy dispersive X-ray spectrometer (EDS) were used to examine the microstructure of both composite powders. TEM specimen was prepared using a combination of micotome, dimple grinder and ion-miller. The crystal structure of the phases in the both as-milled and heat treated powders were characterized using XRD (D/Max-250, Rigaku) with Cu Ka radiation (l=0.154 2 nm) at 30 kV and 50 mA setting.
3 Results
Fig.1 shows the XRD patterns of the powders mechanically alloyed under argon atmosphere (PD) as a function of heat treatment from 300 to 700 ℃. The MA PD powder consists of only Ti peak with no trace of Mg or other Ti-Mg related compound, indicating that Ti and Mg powders form the solid solution by mechanical milling. Annealing the solid solution resulted in a precipitation of Mg from the Ti matrix and also partial oxidation of Ti at 300 ℃. Further oxidation was found to occur, as the temperature increased to 530 ℃. On the other hand, powders in the isopropyl alcohol(PW) have maintained the structures until 300 ℃. Heating to 400 ℃, peaks corresponding to titanium dioxide and magnesium oxide began to appear, followed by further increase and new appearance of additional oxide peaks from 530 ℃.
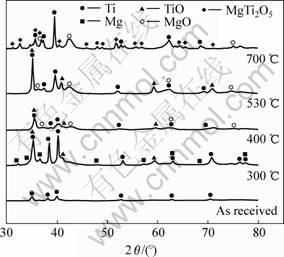
Fig.1 XRD patterns of PD powders mechanically alloyed at different temperatures
Fig.2 shows the TEM bright field images taken from PD (Fig.2(a)) and PW (Fig.2(b)) as received. Both the powders are refined to 100 to 400 nm with irregular shape. This trend is in associated with a strong plastic deformation happened during mechanical milling. However, partial distribution of much finer and spherical powders less than 100nm is simultaneously formed in both the powders (not shown here).
In order to examine the microstructure in detail, parts of the MA powders indicated by arrow in Fig.2(a) and by P and Q in Fig.2(b) were examined using HRTEM as presented in Figs.3 and 4, respectively. It can be seen from Fig.3 that the PD consists of grains (P, Q, R) of 20-30 nm in size within the boundaries (indicated by arrow heads). Inside the grains, lattice fringe of 4-5 nm is embed in amorphous matrix as marked by arrows, as well as partial formation of severe lattice distortion. Much interesting feature is seen in Fig.4 that the areas marked as P and Q in Fig.2(b) give a common information that it consists of three parts from central (described as C) to edge (A) via intermediate (M) phases. Amorphous phase is formed in part A, but the nano lattice fringes are mostly distributed in area M. Because powders have experienced a severe plastic deformation from the surface into the central area by milling, strain begins to accumulate from the surface or edge of powder
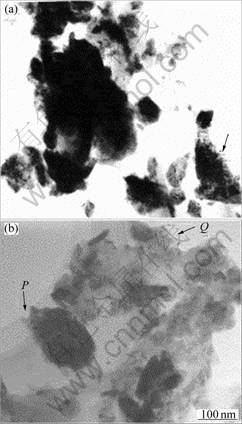
Fig.2 TEM bright field images taken from PD(a) and PW(b)
accompanied by refinement. Further milling may transfer the strain into inner part of particle and then central part, leading to deformation of the particle. Strain induced by milling for 60 h under wet condition may be enough to deform area A and M, which respectively forms amorphous and nanocrystalline embedded in amorphous phase. Because of a little deformation in part C compared with the other regions, the crystalline regions can be seen as dark contrast.
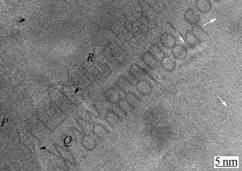
Fig.3 HRTEM image taken from part indicated by arrow in Fig.2(a)
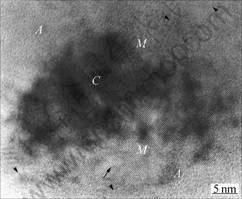
Fig.4 HRTEM image taken from P and Q of PW powder shown in Fig.2(b)
In addition, it presents the existence of edge dislocation with extra-half planes along a large arrow mark, as well as fine lattice fringes of 3-4 nm in diameter (marked by small arrow). To sum up, the MA may be an efficient process to refine the grains, and to make nanocrystalline phases and even also amorphous structures due to the plastic deformation. The EDS analysis using a probe size of 20 nm and diffraction pattern suggests that the nanocrystallites mainly consist of Ti with no precipitation of Mg.
Fig.5 shows the HRTEM image of PD (a) and PW (b) annealed at 530 ℃. The latter consists of a thin amorphous layer of about 3 nm in thickness on the powder (60 nm) surface, analyzed into titanium oxide by EDS spectrum. A few distorts lattices are observed, compared with the as-milled microstructure. SURYANARAYANA[5] reported that Ti-Mg alloy powders usually form metastable solid solution due to heavy cold work during mechanical milling, thus, heat treatment at 900 ℃ induces a stability in the solid solution. He also found a contamination or oxidation occurred above 450 ℃. Thus, heat treatment at medium temperature of 530 ℃ in this experimental condition may induce an oxidation and no complete removal of dislocation.
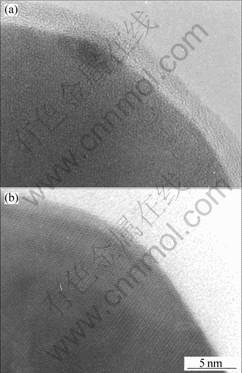
Fig.5 HRTEM image of PD(a) and PW(b) annealed at 530 ℃
However, PD (Fig.5(a)) presents to form a full oxide powder, especially at the extremely fine powders less than 60 nm. It suggests that the alloy is sensitive to atmospheric condition during MA, in which the oxidation could easily occur under dry condition. Comparing both the oxidation patterns gives a clue that the milling medium of isopropyl alcohol may be more effective to prohibit the oxidation.
4 Conclusions
Ti-Mg alloy powders mechanically alloyed under PD and PW form commonly solid solution with reducing the size range of 100 nm to 400 nm in irregular shape. The PD consists of nanocrystals of 4-5 nm embedded in the amorphous matrix of about 20-30 nm in size. In contrast, PW consists of three parts of crystalline, amorphous and combined phases due to the degree of plastic deformation which beginning to accumulate from the surface. The edge dislocation with extra-half planes as well as fine lattice fringes of 3-4 nm in diameter exists. Heating at 530 ℃ make both the PD and PW form a thin amorphous layer of about 3 nm in thickness on the powder surface, and a full oxide powder, suggesting the oxidation is more sensitive to the dry condition.
References
[1] WILKES D M J, GOODWIN P S, WARD-Close C M, BAGNALL K, STEEDS J. Mater Lett, 1996, 27: 47.
[2] SUN F S, FROES F S. Mat Sci Eng A, 2003, 345: 255.
[3] SURYANARAYANA C, FROES F H. J Mater Res, 1990, 5(9): 1880.
[4] ZHOU E, SURYANARAYANA C, FROES F H. Mater Lett, 1995, 23: 27.
[5] SURYANARAYANA C. Prog Mater Sci, 2000, 46: 1.
[6] MURTY B S, RANGANATHAN S. Internat Mater Rev, 1998, 43: 101.
[7] HONG S J, KIM T S, SURYANARAYANA C, CHUN B S. Metal Mater Trans A, 2001, 32(3): 821.
[8] HONG S J, KIM T S, SURYANARAYANA C, CHO D H, CHUN B S. J Mater Synth Proc, 2001, 9(1): 39.
(Edited by LONG Huai-zhong)