
Aging characteristics of new metastable beta titanium alloy
GE Peng(葛鹏), ZHOU Wei(周伟), ZHAO Yong-qing(赵永庆), ZHOU Lian(周廉)
Northwest Institute for Non-ferrous Metal Research, Xi’an 710016, China
Received 15 July 2007; accepted 10 September 2007
Abstract: The aging characteristics including microstructures and properties were investigated for a new metastable beta titanium alloy named Ti-B20 subjected to different solution treatments. Microstructural examination shows that precipitate-free zones, which are present in the many metastable beta titanium alloys, are not produced in the aged new alloy. The decrease of yield stress with the increase of aging temperature is due to the coarsening of alpha platelet. Furthermore, the age hardening effect of the new alloy strengthens with the increase of solution treatment temperature. These aging characteristics are attributed to the relatively lower concentration of beta stabilizer in the new alloy.
Key words: titanium alloy; aging; microstructure; tensile properties; precipitation
1 Introduction
Metastable beta titanium alloy is an attractive material in the applications of critical high-stress and corrosive service due to its excellent hot formability, deep hardenability and superior corrosion resistance[1-4]. Molybdenum equivalence (Mo.Eq.) is often considered to evaluate the overall beta stability for titanium alloy with various alloying element additions. In order to retain 100% beta phase upon quenching from above the beta transus, the molybdenum equivalence should be greater than 10[3]. For some reasons, the Mo.Eq.s of most commercial metastable beta titanium alloys are relatively high. As a result, a series of negative influences on aging response occur, such as, slower aging rate, lower hardening effect and lower homogeneity of precipitates[5-6]. Therefore, it is necessary to develop new metastable beta titanium alloy by decreasing Mo.Eq. Based on such consideration, a few beta titanium alloys have been developed recently, which exhibit excellent properties[7]. However, it should be noted that the orthorhombic α″-phase will be formed in some near beta titanium alloys after beta quenching[8]. Ti-B20 is such a kind of titanium alloy designed by ‘Mo.Eq. controlling’ with a nominal composition of Ti-3.5Al-5Mo-4V-2Cr-1Fe-4(Zr+Sn)(mass fraction, %), whose Mo.Eq. is 10.3. The purpose of this study is to exhibit the aging characteristic of Ti-B20 alloy.
2 Experimental
A 25 kg ingot of Ti-B20 alloy was produced via vacuum arc remelting for this research and then was forged at 1 050 ℃ followed by hot-rolling into bars of 18 mm in diameter at 850 ℃. Solution treatments were carried out at different temperatures for 30 min and then water quenching to room temperature. The specimens were then precipitate hardened by aging at 500, 550, 570 and 600 ℃. For comparison, the aging microstructures of Ti-10Mo-8V-1Fe-3.5Al and Ti-15V-3Cr-3Sn-3Al alloys were also performed at the given temperatures. Optical microscopy (OM), scanning electron microscopy (SEM), scanning electron microscopy (SEM), examinations and X-ray diffraction (XRD) analysis were performed to characterize the alloy. The metallographic observations indicate that the beta transus temperature of this alloy is about 810℃. Tensile specimens were machined into cylindrical shape with a gauge length of 25 mm and a diameter of 5 mm. Tensile tests were carried on an Instron 1185 Universal Testing Machine at room temperature (25 ℃).
3 Results and discussion
The solution temperature is generally controlled within the beta field for metastable beta titanium alloys. In contrary, the solution temperature is below beta transus for near beta titanium alloys. Considering the relative low molybdenum equivalent in the new alloy, both above and below beta transus solution treatment were selected in this study. The solution temperatures are 750, 780, 830 and 860 ℃, respectively. All specimens were kept 0.5 h at the solution temperatures. The X-ray diffraction (XRD) analysis shows that beta solution treatment results in the formation of single beta structure at 860 ℃ and 830 ℃. No alpha phase is detected. However, the biphase of beta plus alpha is formed after solution treatment below 810 ℃, as shown in Fig.1. The TEM examination reveals that the solution treatment at 780 ℃ results in the formation of strip phase (Fig.2(a)). This phase is regarded as primary alpha by its selected area diffraction pattern (SADP) shown in Fig.2(b).
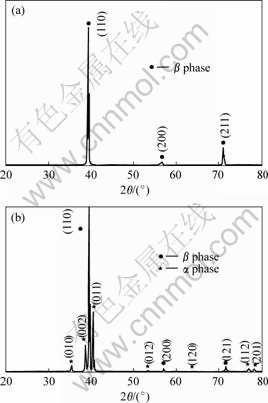
Fig.1 X-ray diffraction patterns for Ti-B20 samples after solution treatment at 860 ℃(a) and 750 ℃ (b)
Further aging was performed for the new alloy after solution treatment. Metastable beta titanium alloys with high molybdenum equivalent, such as Ti-3Al-8V-6Cr- 4Zr-4Mo, tend to age inhomogeneously at temperatures higher than 500 ℃. As a result, local weak regions of precipitate-free zones (PFZ) are produced, and cracks initiate preferentially in these areas[5-6]. At higher aging temperatures, the direct heterogeneous nucleation of the alpha phase is favored at grain boundaries and dislocations. Fig.3(a) shows that non-uniform microstructure is formed in the Ti-10Mo-8V-1Fe-3.5Al specimen aged for 8 h at 570 ℃, and large PFZs are observed. However, the scale of PFZ in Ti-15V-3Cr-3Sn-3Al specimen is significantly decreased (Fig.3(b)). Figs.3(c) and (d) show the microstructures of Ti-B20 specimens treated by 860 ℃ solution followed by aging at 600 ℃. It can be observed that the distribution of precipitates is quite homogeneous in this alloy. Furthermore, no PFZ’s are observed in the aged Ti-B20 specimens. At higher magnification (Fig.3(d)), the alpha platelet is very small.
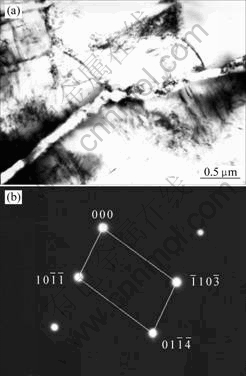
Fig.2 TEM analysis showing primary alpha in Ti-B20 specimens after solution treatment at 780 ℃: (a)Bright-field image; (b) SADP
The formation of PFZs in beta titanium alloys is related with the stability of metastable beta phase and the aging temperature. The increase of either the stability of metastable beta phase or the aging temperatures will promote the formation of PFZs. Due to the relative lower concentration of beta stabilizer in Ti-B20 alloy, the stability of the quenching beta phase is believed to be low. The PFZ is, therefore, difficult to produce during aging. In additional, the planar nature of the grain boundary phase and the large grain size lead to the long slip path in the Ti-B20 specimens treated by beta solution plus aging. The high stress concentration and local strain occur at the grain boundary especially at triple points. In these regions, crack initiates and propagates along grain boundaries and fracture occurs even at low macroscopic strains. As a result, low tensile ductility appears.
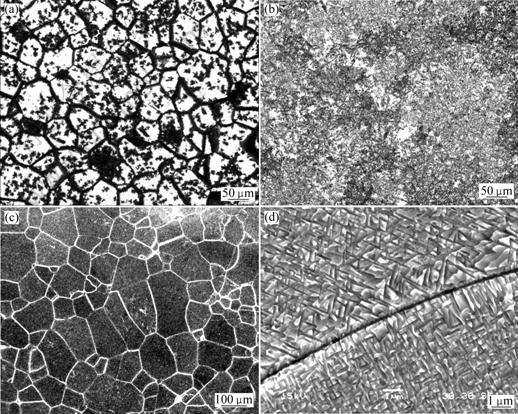
Fig.3 Comparison of microstructure for different aged Ti alloys: (a) Ti-10Mo-8V-1Fe-3.5Al, beta solution followed by aging at 570℃ for 8 h; (b)Ti-15V-3Sn-3Cr-3Al, beta solution followed by aging at 570 ℃ for 8 h; (c) and (d) Ti-B20, water quenched from 860℃ followed by aging at 600 ℃ for 8 h
Fig.4 shows the microstructures of Ti-B20 specimens aged at 500℃ and 570℃ after water quenching from different solution temperatures. The results show that the second alpha precipitates exhibit a fine platelet structure. In spite of the solution temperatures, the alpha precipitation aged at 500 ℃ is finer than that aged at 570 ℃.
For conventional beta titanium alloys, higher solution treatment temperatures always result in more recovery and recrystallization thus decreasing the stored energy and density of dislocation. Therefore, low solution temperatures generally make for good aging response. However, Ti-B20 alloy exhibits significant distinction. Fig.5 shows the aging properties of Ti-B20 specimens after water quenching from different solution temperatures. It can be seen that the tensile strength increases with the increase of solution temperatures, and the changing tendency of tensile ductility is opposite to solution temperatures. Apparently, such trends cannot be explained by the effect of recovery or recrystallization (the higher the solution temperature, the fuller the recovery of recrystallization) on aging response.
When Ti-B20 specimens are solution treated below beta transus, a certain amount of primary alpha phase will precipitate and the beta stabilizer will be enriched in retained beta phase. As discussed above, the lower the solution temperatures, the more the primary alpha phases. Such changing tendency can also be clearly displayed by comparing the distribution of primary alpha after solution treatment at 750 ℃ as shown in Fig.4(e) with that shown in Fig.4(d). Hence, the concentration of beta stabilizer in retained beta phase is decreased with the increase of solution temperatures. The aging strength depends on the size and volume fraction of precipitates. The age hardening effect of Ti-B20 alloy will increase with the increase of solution temperature due to the decrease of beta phase stability and increase of the volume fraction of metastable beta phase, when the alloy is solution treated below beta transus. However, such reason cannot explain the difference of aging properties for Ti-B20 specimens after solution treatment at 830 ℃ and 860 ℃. In these cases, the quenched structures are single beta phase and the composition of beta phase is equal to the nominal composition of this alloy. Considering the effect of recrystallization on aging response[6], it seems that the excess vacancies after solution treatment play an important role on aging treatment. It is well known that vacancies are the inherent faults in alloys, and the excess vacancies are beneficial for the nucleation of precipitates. Grain boundaries and dislocations usually act as the preferential sites for heterogeneous nucleation. Compared with grain boundaries and dislocations, the influence of vacancies on precipitation is relatively weak. However, this effect becomes important when the alloy’s driving force of precipitation is high. Here the relatively low stability of metastable phase in Ti-B20 specimens after beta solution treatment provides the possibility for vacancies to operate. The equilibrium concentration of vacancy in this alloy increases with the increase of treatment temperatures, the concentration of excess vacancies in the specimens solution treated at 830 ℃ is lower than that solution treated at 860 ℃. Consequently
the alpha platelet in 830℃ solution treated specimens as shown in Fig.4(f) is coarser than that in 860 ℃ solution treated specimens under the same aging conditions. Therefore, the tensile strength is increased with the increase of solution temperature.
Fig.6 shows the variation of yield strength versus the second alpha platelet thickness. The results show that the higher the aging temperature, the coarser the alpha platelet thickness and the lower the yield strength. Therefore, the decrease of yield stress with aging temperature could be attributed to the decrease of alpha platelet thickness. Furthermore, the distribution of primary alpha phase shown in Fig.4 is not regular. The slip initiates in the beta phase and that parallel alpha plates of the same variant allow dislocations to shear the alpha phase, while alpha plates with different orientations promote homogeneous slip. Such aging microstructure in Ti-B20 specimens after solution treatment below beta transus is beneficial to the tensile ductility.
Fig.4 SEM micrographs of alpha precipitation in Ti-B20 specimens treated by: (a) 860 ℃, 0.5 h, WQ+500 ℃, 8 h, AC; (b) 860 ℃, 0.5 h, WQ+500 ℃, 8 h, AC; (c) 780 ℃, 0.5 h, WQ+500 ℃, 8 h, AC; (d) 780 ℃, 0.5 h, WQ+500 ℃, 8 h, AC; (e) 750 ℃, 0.5 h, WQ+570 ℃, 8 h, AC; (f) 830 ℃, 0.5 h, WQ+570 ℃, 8 h, AC
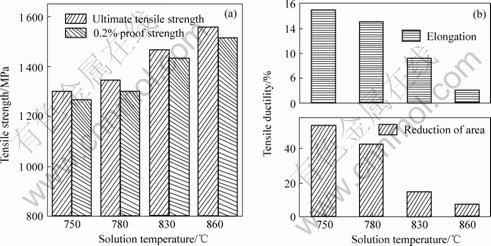
Fig.5 Tensile properties of Ti-B20 specimens aged at 570 ℃ after cooling from different temperatures: (a) Strength; (b) Ductility
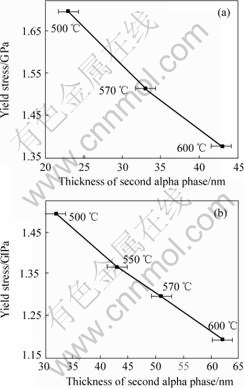
Fig.6 0.2% proof stress versus second alpha platelet thickness of Ti-B20 specimens aged at different temperatures for 8 h after solution treatment at 860 ℃(a) and 780 ℃(b)
4 Conclusions
1) Compared with traditional metastable beta titanium alloys the PFZ in Ti-B20 alloy is difficult to produce during aging due to the relatively lower concentration of beta stabilizer.
2) Beta solution results in ultra high age hardening effect with low tensile ductility, whereas a good combination of tensile strength and ductility can be obtained after solution treated below beta transus. Under the conditions, the variation of strength exhibits a liner relationship with the size of alpha precipitates especially the thickness of alpha platelet during aging.
3) The tensile strength of Ti-B20 alloy increases with the increase of solution temperatures under the same aging conditions, which is different from most metastable beta titanium alloys.
4) The specific aging characteristics of Ti-B20 alloy come from the relatively lean beta stabilizer content, which leads to the decrease in stability of quenched beta phase with the increase in solution temperature when the specimens are solution treated below beta transus and offers the opportunity for excess vacancies to affect the precipitation.
References
[1] Ivasishin O M, Markovsky P E, Semiatin S L, Ward C H. Aging response of coarse- and fine-grained beta titanium alloys[J]. Mater Sci Eng A, 2005, 405: 296-305.
[2] Doraiswamy D, Ankem S. The effect of grain size and stability on ambient temperature tensile and creep deformation in metastable beta titanium alloys [J]. Acta Mater, 2003, 51(6): 1607-1619.
[3] Bania P. Beta titanium alloys and their role in the titanium industry [C]// Eylon D, Boyer R R, Koss D A. Beta Titanium Alloy in the 1990’s. Warrendale: TMS, 1993: 3-14.
[4] Karasevskaya O P, Ivasishin O M, Semiatin S L, Matviychuk Yu V. Deformation behavior of beta-titanium alloys[J]. Mater Sci Eng A, 2003, 354: 121-132.
[5] Wagner L, Gregory J K. improvement of mechanical behavior in Ti-3Al-8V-6Cr-4Mo-4Zr by duplex aging[C]// Eylon D, Boyer R R, Koss D A. Beta Titanium Alloy in the 1990’s. Warrendale: TMS, 1993: 199-209.
[6] Ferrero J G, Wood J R, Russo P A. Microstructural/ mechanical property relationships in bar products of Beta-C(Ti-3Al-8V-6Cr-4Mo-4Zr)[C]// Eylon D, Boyer R R, Koss D A. Beta Titanium Alloy in the 1990’s. Warrendale: TMS, 1993: 211-226.
[7] TETYUKHIN V, VINOKROV D. Metallurgy of titanium production (titanium sponge, melting, conversion, alloys)[C]// LUTJERING G, ALBRECHT J. Ti-2003 Science and Technology. WILEY-VCH: DGM, 2004: 111-120.
[8] DOBROMYSLOV A V, ELKIN V A. The orthorhombic α″-phase in binary titanium-base alloys with d-metals of V–VIII groups[J]. Mater Sci Eng A, 2006, 438/440: 324-326.
Foundation item: Project(2006DFA53600) supported by the International Science and Technology Cooperation between China and France; Project(2007CB613805) supported by the National Basic Research Program of China
Corresponding author: GE Peng; Tel: +86-29-86231078-806; E-mail: gepeng-1@163.com
(Edited by YANG You-ping)