
Preparation and characterization of spinel Li4Ti5O12 anode material from industrial titanyl sulfate solution
WANG Zhi-guo(王志国), PENG Wen-jie(彭文杰), WANG Zhi-xing(王志兴),
LI Xin-hai(李新海), GUO Hua-jun(郭华军), WU Ling(伍 凌)
School of Metallurgical Science and Engineering, Central South University, Changsha 410083, China
Received 6 July 2009; accepted 30 December 2009
_____________________________________________________________________________________________________
Abstract: Metatitanic acid was synthesized from industrial titanyl sulfate solution via controlling pH during hydrolyzing process. Inductively coupled plasma (ICP) analysis confirmed that a little Fe, Mg and Ca were deposited into precursor TiO2·H2O. Spinel Li4Ti5O12 was prepared by sintering amorphous mixture at 800 ℃ for 16 h. The amorphous mixture was activated by ball-milling at room temperature, using the as-prepared TiO2·H2O and Li2CO3 as raw materials. The sample was characterized by X-ray diffractometry, scanning electron microscopy and electrochemical charge and discharge test. The results show that spinel Li4Ti5O12 is obtained, but it contains a few rutile TiO2 impurities. The sample has fine particles with size of around 50 nm and homogenous size distribution. At room temperature, the initial reversible specific capacity of the sample is 136.9, 128.0, 119.2 and 96.3 mA·h/g at 0.1C, 1C, 2C and 5C, respectively, and the sample shows excellent cycling performance.
Key words: TiOSO4; hydrolyzation; Li4Ti5O12; anode material; electrochemical performance
_____________________________________________________________________________________________________
1 Introduction
There are abundant titanic minerals in China, and Panzhihua ilmenite located in Sichuan Province is one of the largest titanium resources in the world and accounts for about 90% of the total resources in China[1]. Most of Panzhihua ilmenites associate with magnetite which has high-level CaO and MgO, therefore, almost 90% of TiO2 are manufactured with the sulfate process[2], and most of TiO2 are anatase. During sulfate process, ilmenite is firstly leached with concentrated sulphuric acid, and then the Ti-containing solution, which mostly contains TiOSO4, is purified and hydrolyzed. In general, the acid number of industrial titanyl sulfate solution is between 1.7 and 2.1. Concentrated industrial titanyl sulfate solution contains about 500 g/L sulphuric acid, 260 g/L TiO2 and a few impurities such as Ni, Mg, Al, Mn, Ca, Na[3-4]. Therefore, in order to protect environment and enhance economy benefit, using resources synthetically attracts great attention in the world.
Spinel Li4Ti5O12 is viewed as one promising alternative to graphite to be an anode material in lithium ion batteries[5], because of having a spinel related structure, extremely flat discharge and charge plateaus at about 1.55 V, excellent cycling ability and zero-strain insertion[6-8]. Generally, Li4Ti5O12 powders are mainly synthesized by solid-state reactions that involve mechanical mixing of oxides and carbonates, heating at higher temperature (800-1 100 ℃)[9-12] and sol-gel methods[13-16]. Three lithium ions per formula unit of Li4Ti5O12 can be reversibly inserted and extracted. As a consequence, a theoretical specific capacity of 175 mA·h/g is expected. This material has optimistic perspectives, and holds some promise of being used in power tools[17].
In this work, spinel Li4Ti5O12 was prepared by heating the mixture of TiO2·H2O and Li2CO3. TiO2·H2O was obtained by hydrolyzing industrial titanyl sulfate solution without crystal transformation and used as titanium precursor directly. Electrochemical properties were investigated as an anode material for lithium batteries.
2 Experimental
2.1 Preparation of samples
The precursor TiO2·H2O was synthesized as the following procedure. The industrial titanyl sulfate solution was diluted in de-ionized water to obtain 2.5 mol/L Ti-containing solution; then NH3·H2O was dropped into the solution to control the pH=5.0, white precipitate was obtained immediately; after being stirred for 30 min, the precipitate was filtered and washed several times with de-ionized water until SO42- was removed, and then dried in an oven at 80 ℃. Thus, TiO2·H2O powders were obtained.
Spinel Li4Ti5O12 was prepared by heating the amorphous mixture of TiO2·H2O and Li2CO3 at 800 ℃ for 16 h in air. The molar ratio of lithium and titanium was 4?5. The mixture was activated by ball-milling at room temperature firstly.
2.2 Detection methods
Elements contained in samples were investigated by inductively coupled plasma (ICP, Thermo Electron Corporation). The powder X-ray diffraction (XRD, Rint-2000, Rigaku) measurement using Cu Kα radiation was employed to identify the crystalline phase of the synthesized materials and the data was recorded at room temperature. The particle size and morphology of the prepared powders were observed by scanning electron microscopy (JEOL, JSM-6380LV) with an accelerating voltage of 20 kV.
2.3 Electrochemical measurements
The electrochemical characterizations were performed using CR2430 coin-type cell. For positive electrode fabrication, the prepared powders were mixed with 10% (mass fraction) acetylene black as conducting agent, and 10% (mass fraction) polyvinylidene fluorides as binder in N-methyl pyrrolidinone. And then, the blended slurries were pasted onto a copper current collector, and the electrode was dried at 120 ℃ for 6 h. The test cell consisted of the positive electrode and lithium foil negative electrode was separated by a porous polypropylene film, and 1 mol/L LiPF6 in EC?EMC?DMC (1?1?1 in volume) as the electrolyte. The assembly of the cells was carried out in a dry Ar-filled glove box. The cells were charged and discharged over a voltage range of 1.0 to 2.5 V versus Li/Li+ electrode at the rate of 0.1C, 1C (160 mA/g), 2C and 5C at room temperature. Cyclic voltammogram (CV) test was performed on a CHI660A electrochemical workstation in voltage range of 0.5 to 2.5 V versus Li/Li+ electrode at the scanning rate of 0.1, 0.5, 1 mV/s.
3 Results and discussion
3.1 Characterization of precursor TiO2·H2O
Fig.1 shows a SEM image of the precursor TiO2·H2O synthesized by hydrolyzing industrial titanyl sulfate solution. The sample exhibits a uniform fine-grained microstructure, which is conducive to the synthesis of Li4Ti5O12 fine powders.
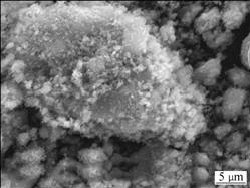
Fig.1 SEM image of precursor TiO2·H2O
The ICP results of the industrial titanyl sulfate solution (2.5 mol/L Ti) and the solution after hydrolyzing are given in Table 1. 25.0%Fe, 76.0%Mg and 24.3%Ca are deposited into the precursor TiO2·H2O; however, Al and Mn are absent. It is reported that a little Mg and Al doping could improve the electrochemical performance of Li4Ti5O12[9].
Table 1 Impurity contents in raw material and solution after hydrolyzing (mass fraction, 10-6)
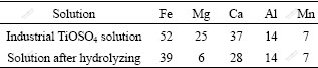
3.2 Characterization of spinel Li4Ti5O12
3.2.1 Structure and morphology analysis
Fig.2 shows the XRD pattern of spinel Li4Ti5O12 prepared by sintering at 800 ℃ for 16 h in air. The diffraction peaks of the sample can be indexed as spinel lithium titanium oxide (cubic phase, space group Fd-3m) in accordance with spinel Li4Ti5O12 (JCPDS Card No. 49-0207). The crystal lattice parameter, a, calculated by using the software WinPLOTR, is 8.359 ?, which shows good agreement to that reported in Ref.[5]. As shown in Fig.2, besides the peaks of Li4Ti5O12, two extra weak diffraction peaks are also observed at 2θ=27.4? and 54.3?, which are attributed to a secondary phase of rutile TiO2. The presence of impurity phase is due to the loss of lithium at high temperature, thus the precursor TiO2·H2O is transformed to anatase TiO2, and then transformed to rutile TiO2.
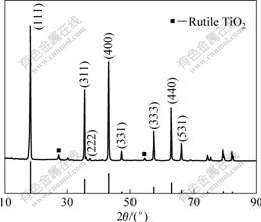
Fig.2 XRD pattern of prepared Li4Ti5O12
The SEM image of Li4Ti5O12 is shown in Fig.3. It can be seen that the dried powder sample show aggregated nanoparticles with homogeneous distribution. The size of the primary particles is around 50 nm.
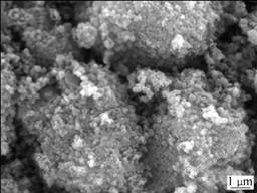
Fig.3 SEM image of prepared Li4Ti5O12
3.2.2 Electrochemical analysis
Fig.4 shows the initial charge/discharge profiles of Li4Ti5O12 at different rates (0.1C, 1C, 2C and 5C) in the voltage range of 1.0-2.5 V. The flat charge and discharge curves, intrinsic electrochemical properties of the Li4Ti5O12 spinel-based anode based on two-phase mechanism, are observed at 1.53 and 1.51 V versus Li/Li+, respectively, and indicate little polarization even up to 5C. As seen in Fig.4, the charge and discharge property is reversible, but the capacity is low.
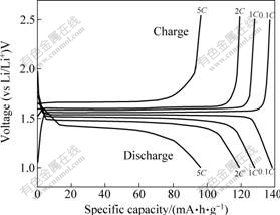
Fig.4 Initial charge/discharge curves of Li4Ti5O12 at different rates in voltage range of 1.0-2.5 V
The initial reversible specific capacity of the sample is 136.9 mA·h/g at 0.1C (1C=160 mA/g). By increasing the rate, the utilization of the active material decreases,128.0, 119.2, and 96.3 mA·h/g at 1C, 2C and 5C rate, respectively. In addition, it can be seen that the coulomb efficiency of the sample is very close to 100%[6]. Thus, Li4Ti5O12 is often called as a zero-strain material with a promising cycling ability[15].
The cycling performance of Li4Ti5O12 at different rates is shown in Fig.5. The cell cycled 10 times at 0.1C, then cycled 20 times at 1C, 2C and 5C in turn, and at last cycled 10 times at 0.1C again. It shows that the capacity of Li4Ti5O12 retains 70.3% at 5C, compared with that at 0.1C. After cycling, the reversible specific capacities at 0.1C, 1C, 2C and 5C are 134.9, 121.9, 113.0 and 86.8 mA·h/g, respectively, and retain 98.5%, 95.2%, 94.8% and 90.1% of their initial reversible specific capacity, respectively. Finally, the capacity can be recovered to 137.9 mA·h/g at 0.1C. That is to say, the structure of Li4Ti5O12 is not destroyed during the charge/discharge cycling. The loss of capacity fluctuation on the capacity-cycle profile at this high current rate also suggests that Li+ insertion/extraction only takes place in the Li4Ti5O12 grains at or near the outer surface of the highly aggregated particles. The capacity is much low at high current rates, and needs to be improved.
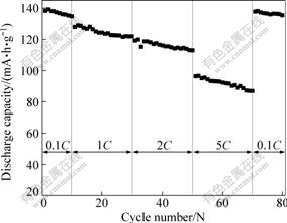
Fig.5 Cycling performance of Li4Ti5O12 at different rates
Fig.6 shows the cyclic voltammograms of Li4Ti5O12 at different scanning rates (0.1, 0.5 and 1 mV/s). At any scanning rate, a pair of reversible redox peaks can be clearly seen, corresponding to a cathodic and anodic process. The cathodic peak located around 1.50 V at 0.1 mV/s corresponds to the voltage plateau of the first cycle discharge process, in which Li intercalates into the spinel Li4Ti5O12, moves steadily to the lower voltage with the increase of scanning rate. Meanwhile, the anodic peak located at 1.66 V corresponds to the voltage plateau of the first cycle charge process, in which Li de-intercalates from the spinel Li4+xTi5O12, moves steadily to the higher voltage with the increase of scanning rate[14, 18]. Moreover, it can be seen that there is a small peak among the cathodic peaks at higher scanning rates in consequence of impurity phase.
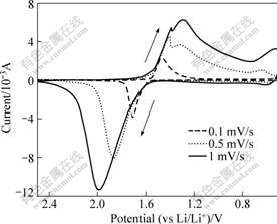
Fig.6 Cyclic voltammograms of Li4Ti5O12 at different scanning rates
4 Conclusions
1) The precursor TiO2·H2O is obtained by hydrolyzing industrial titanyl sulfate solution at pH=5.0. The sample exhibits a uniform fine-grained microstructure. The ICP results show that 25.0% Fe, 76.0% Mg and 24.3% Ca are deposited into the precursor; however, Al and Mn are not precipitated.
2) In order to cut down the cost and simplify the synthesis technology, spinel Li4Ti5O12 is prepared by heating the amorphous mixture of the as-prepared precursor TiO2?H2O and Li2CO3 at 800 ℃ for 16 h in air.
3) XRD analysis shows that Li4Ti5O12 has cubic spinel structure with the impurity rutile TiO2; SEM image shows that the particle is about 50 nm in size together with homogeneous distribution. The initial reversible specific capacity of the sample is 136.9, 128.0, 119.2, 96.3 mA·h/g at 0.1C, 1C, 2C and 5C, respectively. Li4Ti5O12 shows excellent cycling performance.
References
[1] LI C, LIANG B, CHEN S. Combined milling-dissolution of Panzhihua ilmenite in sulfuric acid [J]. Hydrometallurgy, 2006, 82(1/2): 93-99.
[2] LI C, LIANG B, GUO L. Dissolution of mechanically activated Panzhihua ilmenites in dilute solutions of sulphuric acid [J]. Hydrometallurgy, 2007, 89(1/2): 1-10.
[3] YUAN Z, WANG X, XU C, LI W, KWAUK M. A new process for comprehensive utilization of complex titania ore [J]. Minerals Engineering, 2006, 19(9): 975-978.
[4] LI C, LIANG B, WANG H. Preparation of synthetic rutile by hydrochloric acid leaching of mechanically activated Panzhihua ilmenite [J]. Hydrometallurgy, 2008, 91(1/4): 121-129.
[5] IZQUIERDO G, WEST A R. Phase equilibria in the system Li2O-TiO2 [J]. Mat Res Bull, 1980, 15(11): 1655-1660.
[6] OUYANG C, ZHONG Z, LEI M. Ab initio studies of structural and electronic properties of Li4Ti5O12 spinel [J]. Electrochemistry Communications, 2007, 9(5): 1107-1112.
[7] WEN Z, YANG X, HUANG S. Composite anode materials for Li-ion batteries [J]. J Power Sources, 2007, 174(2): 1041-1045.
[8] KATAOKAA K, TAKAHASHIA Y., KIJIMAA N., AKIMOTOA J, OHSHIMA K. Single crystal growth and structure refinement of Li4Ti5O12 [J]. J Phys Chem Solids, 2008, 69(5/6): 1454-1456.
[9] ZHAO H, LI Y, ZHU Z, LIN J, TIAN Z, WANG R. Structural and electrochemical characteristics of Li4-xAlxTi5O12 as anode material for lithium-ion batteries [J]. Electrochimica Acta, 2008, 53(24): 7079-7083.
[10] WU L, KAN S, LU S, ZHANG X, JIN W. Effect of particle size and agglomeration of TiO2 on synthesis and electrochemical properties of Li4Ti5O12 [J]. Trans Nonferrous Met Soc China, 2007, 17(s1): 117-121.
[11] HSIAO K, LIAO S, CHEN J. Microstructure effect on the electrochemical property of Li4Ti5O12 as an anode material for lithium-ion batteries [J]. Electrochimica Acta, 2008, 53(24): 7242-7247.
[12] RUAN Yan-li, TANG Zhi-yuan, PENG Qing-wen. Synthesis and electrochemical performance of spinel Li4Ti5O12 electrode material [J]. J Inorg Mater, 2006, 21(4): 873-879. (in Chinese)
[13] HAO Y, LAI Q, LU J, WANG H, CHEN Y, JI X. Synthesis and characterization of spinel Li4Ti5O12 anode material by oxalic acid-assisted sol-gel method [J]. J Power Sources, 2006, 158(2): 1358-1364.
[14] RHO Y H, KANAMURA K. Li+ ion diffusion in Li4Ti5O12 thin film electrode prepared by PVP sol-gel method [J]. J Solid State Chem, 2004, 177(6): 2094-2100.
[15] JIANG C, ICHIHARA M, HONMA I, ZHOU H. Effect of particle dispersion on high rate performance of nano-sized Li4Ti5O12 anode [J]. Electrochimica Acta, 2007, 52(23): 6470-6475.
[16] ZHAO Yao-min, LIU Guo-qun, JIANG Zhi-yu. Preparation and electrochemical properties of Li4Ti5O12 anode for lithium ion battery [J]. Rare Metal Mater Engineering, 2009, 38(6): 1076-1079. (in Chinese)
[17] CHRISTENSEN J, SRINIVASAN V, NEWMAN J. Optimization of lithium titanate electrodes for high-power cells [J]. J Electrochem Soc, 2006, 153(3): 560-565.
[18] TABUCHI T, YASUDA H, YAMACHI M. Mechanism of Li-doping into Li4Ti5O12 negative active material for Li-ion cells by new chemical method [J]. J Power Sources, 2006, 162(2): 813-817.
_______________________
Foundation item: Project(2007CB613607)supported by the National Basic Research Program of China
Corresponding author: PENG Wen-jie; Tel: +86-731-88836633, E-mail: wzg5951578@163.com
(Edited by LIU Hua-sen)