
Preparation of Al2-xYxW3O12 powders by citrate sol-gel process
ZHANG Hai-jun(张海军), ZHANG Qing(张 青), JIA Quan-li(贾全利), YE Guo-tian(叶国田)
High Temperature Ceramics Institute, Zhengzhou University, Zhengzhou 450052, China
Received 12 December 2007; accepted 23 April 2008
Abstract: Al2-xYxW3O12 (x=0.2, 0.5, 0.8, 1.0, 1.2, 1.5, 1.7 and 2.0) powders were synthesized by citrate sol-gel process. The concentration of species in a citric solution for preparing Al2-xYxW3O12 powders was calculated. The powders were characterized by differential thermal analysis(DTA), thermogravimetry(TG), X-ray diffractometry(XRD) and scanning electron microscopy(SEM), respectively. No solid solution of Al2-xYxW3O12 is formed with x values varying from 0 to 2.0. The maximum solid solubility of Y2O3 in Al2W3O12 and Al2O3 in Y2W3O12 is less than 0.5. Y2W3O12 easily absorbs water in air and forms a composition of Y2W3O12?3.2H2O, and Al2W3O12 forms Al2W3O12?0.17H2O in the same condition.
Key words: Al2-xYxW3O12; citrate sol-gel; preparation; hydration
1 Introduction
Most substances expand during heating. Recently, materials that exhibit negative thermal expansion(NTE) have attracted considerable interest because of the potential to prepare composite materials with a specific coefficient of thermal expansion, positive, negative, or zero in some specific temperature range. There are many materials that exhibit negative thermal expansion such as zeolites, b-quartz, some AMO3 oxides (such as BaTiO3, PbTiO3) with the perovskite structure[1], compounds with general formula A2(MO4)3 (A: trivalent cations and M: W6+, Mo6+, etc)[2-3], and compounds such as ZrMo2O8, HfMo2O8, Zn2GeO4[4] and Zr1-xHfxW2O8[5], ZrW2O8[6-7].
It is well known that the Pechini or citrate sol-gel process is usually considered to have the advantage of even mixing of starting materials, and easy control of the accurate composition of the final products[8-11]. To the knowledge of authors, there are still very few reports on the preparation of Y2W3O12 and Al2W3O12 powders by a homogeneous sol-gel process, as most of reported Y2W3O12 and Al2W3O12 powders are synthesized by classical solid-mixing-reaction method[12-16]. In this work, the citrate sol-gel process is used to prepare Al2-xYxW3O12 powders. A model is used to evaluate the influence of the pH value and citric acid content on the concentration of the cations citrate complexes in the starting solution. And the hydration of prepared Al2W3O12 and Y2W3O12 powders in air are also investigated.
2 Experimental
The raw materials utilized were analytical reagent grade yttrium nitrate (Y(NO3)3?6H2O), aluminum nitrate (Al(NO3)3?9H2O), ammonium tungstate ((NH4)5H5- [H2(WO4)]6?H2O) and citric acid (H3Cit). Stoichiometric amounts of yttrium nitrate and aluminum nitrate were dissolved in distilled water, and then citric acid was added. After complete mixing, ammonium tungstate and ammonia solution (about 1 mol/L) were added to get a homogenous transparent solution within a few seconds. The solution was slowly evaporated to form a highly viscous colloid, followed by heating in a temperature range of 120-140 ℃ for 24 h to obtain a dried gel. Finally, the dried gel precursor was calcined at 900 ℃ for 10 h to obtain Al2-xYxW3O12 powders.
Two series of specimens were prepared for XRD tests: 1) For series A, XRD characterization was immediately carried out as soon as the sintering process was finished in order to avoid the absorption of the water; 2) For series B, XRD tests were carried out after the prepared Al2-xYxW3O12 powders were held in air for about 6 months to study the moisture absorption properties of Al2-xYxW3O12 powders. The samples were characterized by PHILIPS (Model X’Pert PRO) diffractometer using Cu Kα radiation under 40 kV and 40 mA. The XRD data were collected in the 2θ range from 10? to 70? with a step of 0.016?.
Differential thermal analysis and thermogravimetric analysis of the precursor were carried out on a NETZSCH STA-449C Thermal Analysis System with a heating rate of 10 ℃/min in flowing air with sample mass of 30 mg. Platinum crucibles were used in TG-DTA analysis and the reference material was alpha-alumina. The powder morphology was observed with scanning electron microscopy(SEM) (Model JSM-5610LV, JEOL, Japan).
3 Calculation of concentration of yttrium and aluminum citrate complexes in Al3+-Y3+- H3Cit solution
For Al2-xYxW3O12 preparation, there are several kinds of ions, such as, Y3+, Y(H2Cit)2+, Y(OH)2+, [Al3+], Al(Cit), Al(HCit)+, Al(OHCit)-, Al(OH)2+, Al(OH)2+, H3Cit, H2Cit-, HCit2- and Cit3- existing in Al3+-Y3+- H3Cit solution. In order to achieve the complete homogeneity of yttrium and aluminum ions at atomic level and accurate control of the precursor composition, it is very important to increase the relative concentration of yttrium and aluminum citrate complexes, such as Y(H2Cit)2+, Al(Cit) and Al(OHCit)- and decreases the relative concentration of Al3+ and Y3+. In the present work, a theoretical model similar to that in Ref.[17] was used to calculate the concentration of yttrium and aluminum citrate complexes in Al3+-Y3+-H3Cit solution under different conditions. The concentration of citric acid and pH value of the solution were taken into consideration.
For Al1.0Y1.0W3O12 preparation, the theoretically calculated yttrium and aluminum complex concentrations at different pH values and concentrations of citric acid are shown in Figs.1 and 2. The following phenomena can be seen from these figures.
1) The concentration of Y3+ and Al(Cit) ions decreases with pH values increasing till pH=5 at first, then the concentration of Y3+ ions increases evidently till pH=7. The concentration of Al(Cit) ions is about zero from pH=5 to 7. The concentration of Al(OHCit)- ions increases with pH increasing. The concentration of Y(H2Cit)2+ increases with pH increasing until pH=5, and then decreases at pH=7. The percentage of [Y(H2Cit)2+] to total yttrium concentration at pH=7 is only about 50% with the 1?1 molar ratio of citric acid to the total cations (Fig.1).
2) The concentration of citric acid shows a little influence on the concentration of Y3+ and Y(H2Cit)2+ ions. The concentration of Y3+ ion decreases with increasing the citric acid content at the same pH value.
3) The concentrations of Y(OH)2+, Al3+, Al(OH)2+, Al(OH)2+, Al(HCit)+ ions are about lower than zero compared with those of Y(H2Cit)2+, Al(Cit), Al(OHCit)- in all conditions.
4) At pH=4 and [H3Cit]T/{[Y]T+[Al]T}=3, almost all yttrium and aluminum ions completely form yttrium citrate complex Y(H2Cit)2+ and aluminum citrate complex Al(OHCit)-, Al(Cit).
According to the theoretically calculation (Figs.1 and 2), at [H3Cit]T/{[Y]T+[Al]T}=3 and pH=4, the percentage of the yttrium and aluminum citrate complex would increase to about 100%, indicating that [H3Cit]T/ {[Y]T+[Al]T}=3?1 and pH=4 are suitable for Al2-xYx- W3O12 precursor synthesis. The calculation results are in agreement with the experimental phenomena, i.e. a transparent sol and gel with no precipitation is easily formed at the pH=4 and [H3Cit]T/{[Y]T+[Al]T}=3?1 in the experiment.
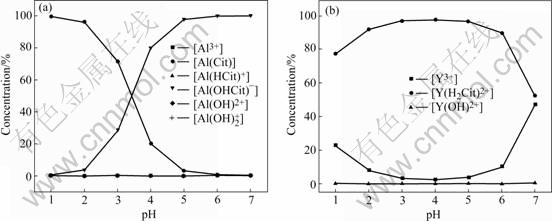
Fig.1 Evaluated concentration of aluminum and yttrium species in solution with 1?1 molar ratio of citric acid to total cations: (a) Al3+- H3Cit; (b) Y3+-H3Cit
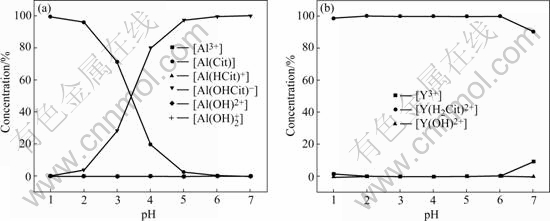
Fig.2 Evaluated concentration of aluminum and yttrium species in solution with 3?1 molar ratio of citric acid to total cations: (a) Al3+- H3Cit; (b) Y3+-H3Cit
4 Results and discussion
4.1 TG-DTA analyses of precursor
The TG-DTA curves of the dry Al1.0Y1.0W3O12 precursor with pH=4 and [H3Cit]T/{[Y]T+[Al]T}=3?1 are shown in Fig.3. The mass loss can be divided into two temperature regions, namely, from room temperature to 225 ℃, and from 225 ℃ to 751 ℃. These two regions correspond to mass loss of about 40%, and 25% respectively. At higher temperature, there is almost no change in mass, which can be attributed to the formation of a pure oxide system. The exothermic peak in the first temperature region (about 225.0 ℃) can be contributed to the decomposition of the citrate Y(H2Cit)2+, Al((OH)Cit-), Al(Cit), (NH4)5H5[H2(WO4)]6?H2O and the formation of the aluminum and yttrium carbonate. The broad exothermic peaks in the second temperature region from about 400 ℃ to 900 ℃ may be caused by the decomposition of the carbonate and the formation of Al2W3O12 and Y2W3O12.
z
4.2 XRD analyses of Al2-xYxW3O12 powders
The X-ray diffraction patterns of prepared Al2-xYxW3O12 calcined at 900 ℃ for 10 h are shown in Fig.4. It can be seen that, 1) pure Al2W3O12 and Y2W3O12 are prepared by citrate sol-gel methods; and 2) Al2-xYxW3O12 can not form a complete solid solution as x values vary continuously from 0.0 to 2.0. The solid solubility limit of Y2O3 in Al2W3O12 is less than 0.5 (molar fraction), and so is that of Al2O3 in Y2W3O12.
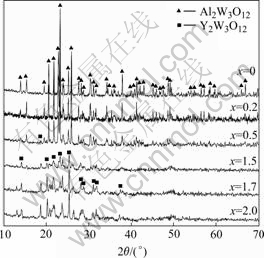
Fig.4 XRD patterns of Al2-xYxW3O12 precursors annealed at 900 ℃ for 10 h
The XRD patterns of series A and series B of Al2W3O12 and Y2W3O12 specimens are shown in Fig.5. It is shown that all the diffraction peaks of series B samples (even the sample re-annealed at 800 ℃) shift to high degree, indicating that the crystal cell volume of series B samples is less than that of series A samples. This result shows that water molecules can be present in the crystallographic voids and cause shrinkage of the structure. The difference in the volume of the hydrated (series B samples) and the unhydrated (series A samples) phase indicates that the water molecules cause shrinkage of the framework structure. It is possible that the Y–O–W linkages are bent away from 180? angle in the hydrated phase. As the water is lost, the Y–O–W angle approaches the 180? linkage angle.
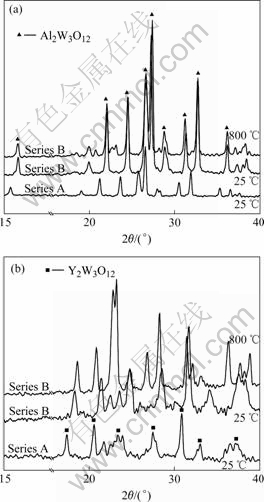
Fig.5 XRD patterns of Al2W3O12 and Y2W3O12 under different condition: (a) Al2W3O12; (b) Y2W3O12
4.3 Moisture absorbing properties of Al2W3O12 and Y2W3O12 powders
TG-DTA analysis of B series Al2W3O12 and Y2W3O12 samples is shown in Fig.6. Based on calculation, the molecule number of absorbed water in B series Y2W3O12 sample hold in air for 6 months is about 3.2, which is in good agreement with the results of KARMAKAR et al[13]. The molecule number of absorbed water in B series Al2W3O12 sample is about 0.17.
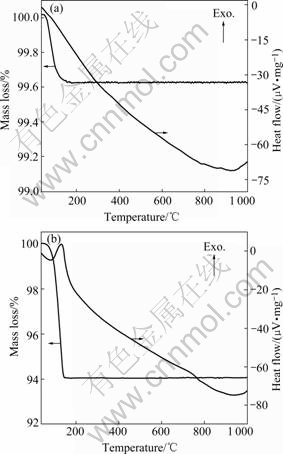
Fig.6 TG-DTA results of B series Al2W3O12 (a) and Y2W3O12 (b) samples
4.4 SEM characterization of Al2W3O12 and Y2W3O12 powders
The SEM micrographs of Al2W3O12 and Y2W3O12 samples annealed at 900 ℃ for 10 h are shown in Fig.7. The particles of Al2W3O12 and Y2W3O12 powders are widely distributed with the larger ones of more than 40mm and the smaller ones of just 3-5 mm. It is also seen from Fig.7 that the particle size of Al2W3O12 is much larger than that of Y2W3O12.
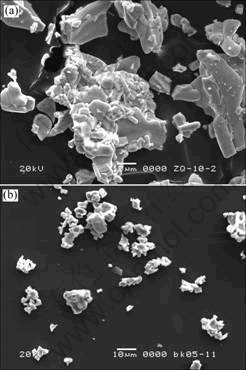
Fig.7 SEM photographs of Al2W3O12 (a) and Y2W3O12 (b) powder prepared at 900 ℃ for 10 h
5 Conclusions
1) The calculation results show that increasing the concentration of citric acid and pH values can increase the amount of yttrium and aluminum citrate, which plays a positive role in the formation of Al2-xYxW3O12 gel. The concentration of those different cation citrate complexes decided by citric acid and pH values in the starting solution influences the formation of sol and further affects precipitation and segregation during gelling and charring.
2) Al2-xYxW3O12 powders are formed by citrate sol-gel process using yttrium nitrate, aluminum nitrate, ammonium tungstate and citric acid as starting materials. The optimum conditions for Al2-xYxW3O12 powder synthesis are [H3Cit]T/{[Y] T+[Al]T}=3?1, pH=4 and calcining temperature of 900 ℃.
3) The maximum solid solubility of Y2O3 in Al2W3O12 and Al2O3 in Y2W3O12 is less than 0.5. Y2W3O12 powders absorb much more water than Al2W3O12 powders in air under the same condition. Y2W3O12?3.2H2O and Al2W3O12?0.17H2O form from Y2W3O12 and Al2W3O12 respectively after being left in air for 6 months.
References
[1] SLEIGHT A W. Negative thermal expansion materials [J]. Current Opinion in Solid State & Materials Science, 1998, 3(2): 128-131.
[2] SUMITHRA S, TYAGI A K, UMARJI A M. Negative thermal expansion in Er2W3O12 and Yb2W3O12 by high temperature X-ray diffraction [J]. Materials Science and Engineering B, 2005, 116(1): 14-18.
[3] EVANS JOHN S O, MARY T A. Structural phase transitions and negative thermal expansion in Sc2(MoO4)3 [J]. International Journal of Inorganic Materials, 2000, 2(1): 143-151.
[4] STEVENS R, WOODELD B F, JULIANA B G, CRAWFORD M K. Heat capacities, third-law entropies and thermodynamic functions of the negative thermal expansion material Zn2GeO4 from T=0 to 400 K [J]. Journal of Chemical Thermodynamics, 2004, 36(5): 349-357.
[5] NAKAJIMA N, YAMAMURA Y, TSUJI T. Phase transition of negative thermal expansion Zr1-xHfxW2O8 solid solutions [J]. Journal of Thermal Analysis and Calorimetry, 2002, 70(2): 337-344.
[6] TSUJI T, YAMAMUR Y, NAKAJIM N. Thermodynamic properties of negative thermal expansion materials ZrW2O8 substituted for Zr site [J]. Thermochimica Acta, 2004, 416(1/2): 93-98.
[7] STEVENS R, LINFORD J, WOODFIELD B F, JULIANA B G, LIND C, WILKINSON A P, KOWACH G. Heat capacities, third-law entropies and thermodynamic functions of the negative thermal expansion materials, cubic α-ZrW2O8 and cubic ZrMo2O8, from T=(0 to 400) K [J]. Journal of Chemical Thermodynamics, 2003, 35(6): 919-937.
[8] AZADMANJIRI J. Preparation of Mn-Zn ferrite nanoparticles from chemical sol-gel combustion method and the magnetic properties after sintering [J]. Journal of Non-crystalline Solids, 2007, 353(44/46): 4170-4173.
[9] ZHANG X Q, YUAN D R, GUO S. Sol-gel preparation of a new compound with Ca3Ga2Ge4O14 structure: La3Al5.5Ta0.5O14 [J]. Journal of Crystal Growth, 2007, 308(1): 80-83.
[10] LAVELA P, TIRADO J L, VIDAL-ABARCA C. Sol-gel preparation of cobalt manganese mixed oxides for their use as electrode materials in lithium cells [J]. Electrochimica Acta, 2007, 52(28): 7986-7995.
[11] KUMAR M, YADAV K L. Magnetoelectric characterization of xNi0.75Co0.25Fe2O4-(1-x)BiFeO3 nanocomposites [J]. Journal of Physics and Chemistry of Solids, 2007, 68(9): 1791-1795.
[12] TYAGI A K, ACHARY S N, MATHEWS M D. Phase transition and negative thermal expansion in A2(MoO4)3 system (A=Fe3+, Cr3+and Al3+) [J]. Journal of Alloys and Compounds, 2002, 339(1/2): 207-210.
[13] KARMAKAR S, DEB S K, TYAGI A K, SHARMA S M. Pressure-induced amorphization in Y2(WO4)3: In situ X-ray diffraction and Raman studies [J]. Journal of Solid State Chemistry, 2004, 177(11): 4087-4092.
[14] WOODCOCK D A, PHILIP L, RITTER C. Negative thermal expansion in Y2(WO4)3 [J]. Journal of Solid State Chemistry, 2000, 149(1): 92-98.
[15] FORSTER P M, SLEIGHT A W. Negative thermal expansion in Y2W3O12 [J]. International Journal of Inorganic Materials, 1999, 1(2): 123-127.
[16] SUMITHRA S, UMARJI A M. Hygroscopicity and bulk thermal expansion in Y2W3O12 [J]. Materials Research Bulletin, 2005, 40(1): 167-176.
[17] LEE W J, FANG T T. The effect of the molar ratio of cations and citric-acid on the synthesis of barium ferrite using a citrate process [J].Journal of Materials Science, 1995, 30(17): 4349-435.
Foundation item: Project(0512002400) supported by the Fund for Distinguished Young Scholars of Henan Province, China
Corresponding author: ZHANG Hai-jun; Tel: +86-371-67761908, Fax: +86-371-67763822; E-mail: zhizdl@yahoo.com.cn, zhanghaijun@zzu.edu.cn
(Edited by YANG Bing)