Trans. Nonferrous Met. Soc. China 23(2013) 2989-2993
Preparation and properties of novel Cu-based bulk metallic glasses Cu55-xZr37Ti8Inx
Jin-hong PI1,2, Ye PAN1, Ji-li WU1, Lu ZHANG1, Xian-cong HE2
1. Jiangsu Key Laboratory for Advanced Metallic Materials, School of Materials Science and Engineering, Southeast University, Nanjing 211189, China;
2. School of Materials Engineering, Nanjing Institute of Technology, Nanjing 211167, China
Received 12 September 2012; accepted 18 May 2013
Abstract: The glassy rods were successfully fabricated in the Cu-Zr-Ti-In alloy system by casting into a copper mold. The value of ΔTx reaches a maximum of 66 K for the BMG Cu50Zr37Ti8In5 alloy. The reasons for enhancing glass forming ability of Cu-based BMGs with the addition of indium were discussed from atomic size and thermodynamics. Alternatively, the BMG Cu52Zr37Ti8In3 exhibits the highest compressive strength (1981 MPa) and the best plasticity among glassy Cu55-xZr37Ti8Inx (x≤5). The total plastic deformation of Cu52Zr37Ti8In3 before fracture approaches 1.2%.
Key words: Cu-Zr-Ti-In alloy; indium; Cu-based alloy; bulk metallic glass; glass forming ability; mechanical properties
1 Introduction
Bulk metallic glasses (BMGs) have excellent properties such as high strength [1], large elastic elongation [2], excellent corrosion resistance [3], good soft magnetic properties [4], smooth surface and viscous deformability [5]. Now, lots of BMGs with different compositions have been developed in various systems such as Al-, Mg-, Zr-, Pd-, Ti-, Fe- and Cu-based alloys [6,7]. Compared with Zr- and Pd-based BMGs, Cu-based BMGs have attracted more and more attention, due to their relative high glass forming ability (GFA), excellent mechanical and chemical properties and low cost [8], and are also considered advanced engineering materials in future. Therefore, designing new compositions of Cu-based BMGs and improving the properties of Cu-based BMGs are worthy to be studied.
Up to date, Cu-based BMGs have been developed in systems, such as Cu-Zr-Ti [9], Cu-Zr-Al [10] and Cu-Zr-Ag [11]. Since the Cu-based BMGs in Cu-Zr-Ti system have relative high GFA [3] without noble metals, such as Pd and Ag, many researchers have shown interests in the investigation of this alloy system and in improving its GFA by minor alloying [12,13]. However, no Cu-based BMGs in Cu-Zr-Ti-In system were reported according to the published references. According to Ref. [14], the atomic radii of Cu, Zr, Ti, and In are 0.127, 0.160, 0.145, and 0.162 nm, respectively. And the mixture heat of In-Cu, In-Zr and In-Ti are 10, -25 and -5 kJ/mol, respectively [15]. As we know, the GFA can be strongly affected by the large negative heat of mixing among the constituent elements [10]. Therefore, the atomic size range is expanded and the mixing heat may be reduced after the addition of element In, which may be favorable for improving GFA. Additionally, ZHENG et al [16] successfully enhanced the ductility of Ti-based BMG by adding soft atom, element In. Therefore, a new series of BMGs in Cu-Zr-Ti-In systems were fabricated and characterized using Cu55Zr37Ti8, of which the critical size is 3 mm in diameter, as a starting material [17] in this work.
2 Experimental
Mixtures of pure elements (the purity is between 99% and 99.99%) with the nominal composition of Cu55-xZr37Ti8Inx (x≤5) were arc-melted under a Ti-gettered argon atmosphere. In order to ensure chemical homogeneity of ingots, each ingot was melted at least four times. Subsequently, the arc-melted ingot was re-melted and cast into a rod-shaped cavity in a copper mold with a diameter of 3-4 mm. The microstructures of cross-sections of the samples were characterized using X-ray diffraction with a Cu Ka radiation (XRD, D8 Discovery) and scanning electron microscopy (SEM, JEOL JSM-6360LV). The glass transition temperature, the onset temperature of crystallization and the melting temperature were measured by differential scanning calorimetry (DSC) (NETZSCHSTA 449F3) with a heating rate of 20 K/min. Room temperature compressive property of each sample (d3 mm×6 mm) was tested on materials testing machine (Instron MTS569) at a strain rate of 3×10-4 s-1.
3 Results and discussion
Figure 1 shows the X-ray diffraction patterns of the as-cast BMGs Cu55-xZr37Ti8Inx (x≤5). It can be seen that when x increases from 0 to 5, all XRD patterns, except 4 mm-diameter Cu54Zr37Ti8In1 alloy rod, exhibit only a broad diffraction peak without any detectable sharp peaks of crystalline phases, indicating the formation of amorphous structure. In the XRD pattern of 4 mm- diameter Cu54Zr37Ti8In1 alloy rod, some sharp diffraction peaks, which are identified as Cu10Zr7, superimpose on the broad diffraction peak, suggesting the presence of a little of crystalline phases. Therefore, it can be proposed that the GFA of BMGs Cu-Zr-Ti increases with the addition of In.
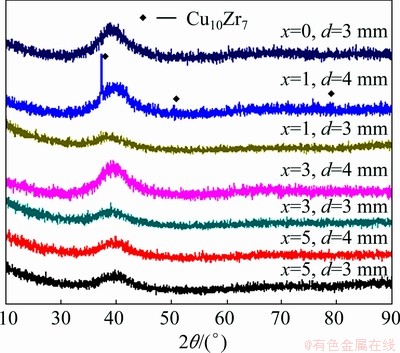
Fig. 1 XRD patterns of Cu55-x Zr37Ti8Inx (x≤5%) alloys
Figure 2 shows the DSC curves of the Cu55-xZr37Ti8Inx (x≤5) alloys (d=3 mm). The glass transition temperature (Tg), onset crystallization temperature (Tx), melting point of alloys (Tm) and liquidus temperature (Tl) are indicated by arrows in the DSC traces. The alloys exhibit distinct glass transitions, followed by super-cooled liquid regions, and then the exothermic reaction corresponding to crystallization. The values of Tg, Tx, ΔTx (ΔTx=Tx-Tg), Tm, Tl, Trg (Trg=Tg/Tl) and γ (γ=Tx/(Tg+Tl)) are listed in Table 1.
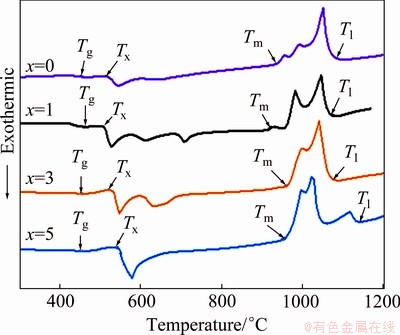
Fig. 2 DSC curves of Cu55-xZr37Ti8Inx (x≤5) BMGs at heating rate of 20 K/min
Table 1 Parameters of Tg, Tx, Tm, Tl, ΔTx, γ and Trg for BMGs Cu55-x Zr37Ti8Inx (x≤5)
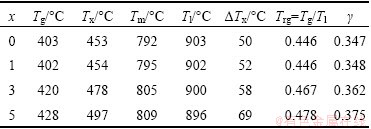
As we can see, both the Tg and Tx increase with increasing In content. The Tg increases from 402 °C at x=1 to 428 °C at x=5, while the Tx increases from 453 °C to 497 °C for In content, x, of 0-5. As a result, the super-cooled liquid region (ΔTx) increases from 50 °C to 69 °C with increasing x from 0 to 5, indicating enhancement of GFA.
Additionally, a higher Trg value indicates that competing crystalline phase can be restrained to form glassy phase at a relative low cooling rate, resulting in the high GFA [18]. It is reasonable to suppose that the addition of In element brings higher GFA because with In content increasing from 0 to 5%, Trg increases from 0.446 to 0.478.
The results can be also explained by a thermodynamic approach. Previous works demonstrated that the smaller Gibbs free energy difference (ΔGl-s) between the liquid and corresponding crystalline phases means the more restriction of competing crystalline phase and the better GFA. The value of ΔGl-s can be estimated by the following equation:
(1)
where ΔHmix is the mixing enthalpy, ΔSmix is the mixing entropy, and T is the temperature of the undercooled liquid. When predicting the GFA before experiments instead of evaluating the GFA after experiments, the temperature of liquid alloy is actually unknown. Accordingly, the value of ΔGl-s can not be calculated exactly with this expression. However, it is known that smaller ΔHmix and larger ΔS result in smaller value of ΔGl-s, which is favored to higher GFA. Therefore, considering the value of ΔS is positive, the liquid stability can be evaluated by the value of ΔHmixΔS when the value of ΔHmix is negative, that is, the more negative the value of ΔHmixΔS is, the higher the GFA is expected.
The value of ΔHmix can be calculated based on the regular melt model [19]:
(2)
where Ωij is the interaction parameter between the ith and jth elements in regular solutions;
is the enthalpy of mixing of atomic pairs between the ith and jth elements; ci and cj are the atomic fraction of the ith and jth element respectively. The values of
of Cu-Zr, Cu-Ti, and Zr-Ti are -23, -9 and 0 kJ/mol, respectively [15].
ΔS can be determined by
(3)
Accordingly, the values of calculated ΔHmix, ΔS and ΔHmixΔS of BMGs Cu55-xZr37Ti8Inx (x≤5%) are listed in Table 2. It can be easily seen that the value of ΔHmix·ΔS increases with x increasing from 0 to 5, meaning an increase of GFA from the viewpoint of thermodynamics. Small amount of In addition (x=1) does not obviously improve the GFA of Cu55Zr37Ti8, which means that simply increasing the amount of components in BMGs does not assure higher GFA. It can improve the GFA of BMGs only when the addition of alloying elements results in obviously smaller value of ΔGl-s.
Table 2 Values of calculated ΔHmix, ΔS and ΔHmixΔS of BMGs Cu55-xZr37Ti8Inx (x≤5)
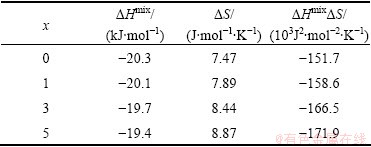
What’s more, with the addition of large atom In, the packing efficiency of atoms is improved, the complexity and mismatch of the alloy system are increased, and finally the GFA is improved.
Figure 3 shows the room temperature compressive curves of Cu55-xZr37Ti8Inx (x≤5). The room temperature compressive strength of BMG Cu52Zr37Ti8In3 is high up to 1981 MPa, which is the highest compressive strength among the tested BMGs in this work. It can also be seen that the total plastic deformation of Cu52Zr37Ti8In3 before fracture is about 1.2%. Further increase of In addition does not apparently help to strengthen the BMGs in our experimental conditions. It should be noted that compositions with different as-quenched structural configurations may show different degrees of densification, which can be reflected by the relative change of strength.
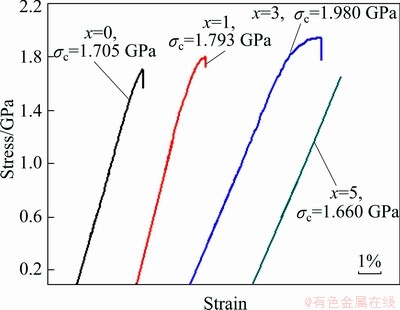
Fig. 3 Room temperature compressive curves of BMGs Cu55-xZr37Ti8Inx (x≤5)
The fracture morphologies of Cu55-xZr37Ti8Inx are shown in Fig. 4. The glassy Cu50Zr37Ti8In5 rod was crushed into pieces under compressive load.
From Fig. 4, typical vein patterns can be observed on the fracture surfaces. And when x increases from 0 to 3, the amount of shear bands on the specimen surfaces increases, and the vein patterns become more consecutive. As we know, the deformation of BMGs under compression is characterized by the formation of shear bands, their rapid propagation, and the sudden fracture of the sample. According to Ref. [8], a very high amount of shear is focused in the shear bands, since when the specimen fractures, a significant amount of energy is released, leading to a large temperature rise, and thus resulting in local softening or melting. The clearly resolidified droplets (pointed by arrows in Fig. 4) on the fracture prove that localized melting occurred. Clearly, more droplets can be found on the fracture of glassy Cu54Zr37Ti8In1 and Cu52Zr37Ti8In3. And the preferential breaking of local atomic clusters rather than inter-atomic bonds in crystalline solids leads to crack growth. Since the shear bands are large planar bands of “shear transformation zone” (STZ) [1], more shear bands means that more STZs are involved during deformation, and more homogenous deformation occurs accordingly. That’s why glassy samples with more shear bands show better plasticity.
Many shear bands with an average spacing of around 5 μm can be clearly observed on the outer surface of Cu52Zr37Ti8In3. That’s why glassy Cu52Zr37Ti8In3 exhibits clear plastic deformation during the fracture process, as shown in Fig. 4. Less shear bands can be observed on the outer surface of other samples, meaning less plasticity.
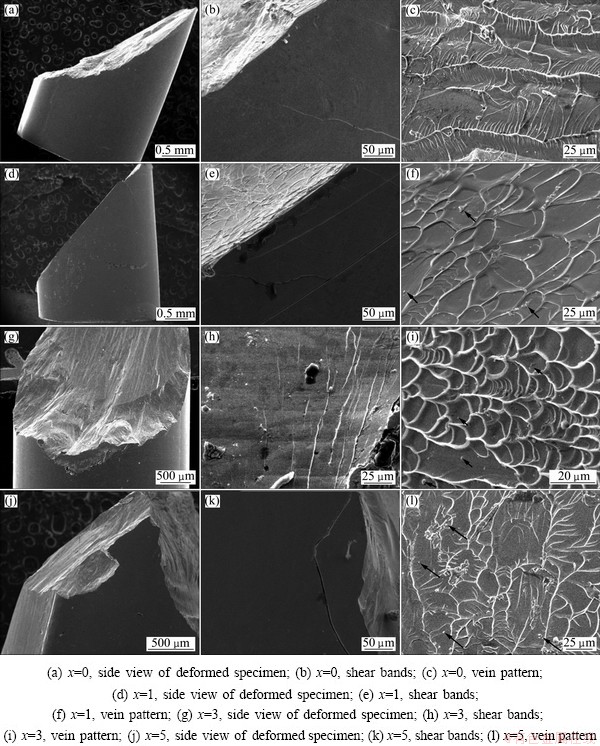
Fig. 4 Fracture morphologies of Cu55-xZr37Ti8Inx (x≤5) glassy rods under compressive deformation mode
4 Conclusions
Fully amorphous structure was successfully fabricated by conventional arc-melting and copper mold suction-casting method in the novel alloy system Cu55-xZr37Ti8Inx (x≤5) with diameter no less than 3-4 mm. The tested BMGs exhibit large temperature intervals of the super-cooled liquid region. The glass forming ability of Cu-based BMGs increases with the addition of In. The reasons are that the introduction of In element increases the complexity and mismatch of the alloy system and lowers the value of ΔHmixΔS, which results in lower Gibbs free energy difference between the liquid and the crystalline phases. Meanwhile, the glassy Cu52Zr37Ti8In3 exhibits the highest compressive strength (1981 MPa) among the tested BMGs, and the total elastic deformation of Cu55-xZr37Ti8Inx (x≤5) before fracture increases with x.
References
[1] TREXLER M M, THADHANI N N. Mechanical properties of bulk metallic glasses [J]. Prog Mater Sci, 2010, 55: 759-839.
[2] Schneider S. Bulk metallic glasses [J]. J Phys: Condens Matter , 2001, 13: 7723-7736.
[3] KOU H C, LI Y, ZHANG T B, LI J, LI J S. Electrochemical corrosion properties of Zr- and Ti-based bulk metallic glasses [J]. Transactions of Nonferrous Metals Society of China, 2011, 21: 552-557.
[4] KOVALENKO N P, KRASNY Y P, KREY U. Physics of amorphous metals [M]. Weinheim: Wiley-VCH Verlag GmbH, 2001: 135-179.
[5] LI H, JIAO Z, GAO J, LU Z. Synthesis of bulk glassy Fe-C-Si-Ga alloys with high glass-forming ability and good soft-magnetic properties [J]. Intermetallics, 2010, 18: 1821-1825.
[6] KUZMIN O V, PEI Y T, HOSSON J T M D. Size effects and ductility of Al-based metallic glass [J]. Script Materialia, 2012, 67: 344-347.
[7] INOUE A, TAKEUCHI A. Recent development and application products of bulk glassy alloys [J]. Acta Materialia, 2011, 59(6): 2243-2267.
[8] SURYANARAYANA C, INOUE A. Bulk metallic glasses [M]. New York: CRC Press, 2011: 431-433.
[9] FU H, ZHANG H, WANG H, HU Z. Cu-based bulk amorphous alloy with larger glass-forming ability and supercooled liquid region [J]. J Alloys Compd, 2008, 458: 390-393.
[10] XU H W, DU Y L, DENG Y. Effects of Y addition on structural and mechanical properties of CuZrAl bulk metallic glass [J]. Transactions of Nonferrous Metals Society of China, 2012, 22: 842-846.
[11] WANG Q, QIANG J, WANG Y, XIA J, DONG C. Bulk metallic glass formation in Cu-Zr-Ti ternary system [J]. J Non Cryst Solids, 2007, 353: 3425-3428.
[12] MILLER M K, LIAW P K. Bulk metallic glasses [M]. New York: Springer, 2007: 13-16.
[13] KONG J, XIONG D S, YUAN Q X, YE Z T. Strengthening bulk metallic glasses with minor alloying additions [J]. Transactions of Nonferrous Metals Society of China, 2006, 16: s598-s602.
[14] XIA J H, QIANG J B, WANG Y M, WANG Q, DONG C. Cu-Zr-Ag bulk metallic glasses based on Cu8Zr5 icosahedron [J]. Mater Sci Eng A, 2007, 449: 281-284.
[15] GAL W F, TOTEMEIER T C. Smithells metals reference book [M]. Burlington: Elsevier Butterworth-Heinemann, 2004: 236-286.
[16] ZHENG N, QU R, PAULY S, CALIN M, GEMMING T, ZHANG Z. Design of ductile bulk metallic glasses by adding “soft” atoms [J]. Appl Phys Lett, 2012, 100(14): 141901.
[17] WU J L, PAN Y, PI J H, ZHANG L. Fabrication of Cu-rich bulk metallic glass composites via solidification method [C]//International Union of Materials Research Society—international Conference in Asia (IUMRS-ICA-2012). Switzerlan: TTP Ltd, 2012.
[18] GENG J Y, SUN Y F, WANG L G, ZHU S J, LIU L Z, GUAN S K. Effect of Al addition on formation and mechanical properties of Mg-Cu-Gd bulk metallic glass [J]. Transactions of Nonferrous Metals Society of China, 2007, 17: 907-912.
[19] ZHANG Q, ZHANG H, DENG Y, DING B, HU Z. Bulk metallic glass formation of Cu-Zr-Ti-Sn alloys [J]. Scripta Mater, 2003, 49: 273-278.
新型铜基块体非晶合金Cu55-x Zr37Ti8Inx的制备及性能
皮锦红1,2,潘 冶1,吴继礼1,张 露1,贺显聪2
1. 东南大学 材料科学与工程学院,江苏省先进金属材料重点实验室,南京 211189;
2. 南京工程学院 材料工程学院,南京 211167
摘 要:利用铜模吸铸法合成Cu-Zr-Ti-In非晶棒。块体非晶合金Cu50Zr37Ti8In5的ΔTx 值最大,为66 K。从原子尺寸大小和热力学角度分析在铜基非晶合金中添加适量In元素后能够提高其非晶形成能力的原因。在所测的块体非晶合金Cu55-xZr37Ti8Inx (x≤5)中,Cu52Zr37Ti8In3表现出最高的抗压强度(1981 MPa)和最佳的塑性,其在压缩断裂前的总塑性变形量约为1.2%。
关键词:Cu-Zr-Ti-In合金;铟;Cu基合金;块体非晶合金;非晶形成能力;力学性能
(Edited by Hua YANG)
Foundation item: Project (50971041) support by the National Natural Science Foundation of China
Corresponding author: Ye PAN; Tel: +86-25-52090681; E-mail: panye@seu.edu.cn
DOI: 10.1016/S1003-6326(13)62825-3