
Effects of electric parameters on corrosion resistance of anodic coatings formed on magnesium alloys
ZHANG Shu-fang(张淑芳)1, 2, HU Guang-hui(胡光辉)3, ZHANG Rong-fa(张荣发)1, 2, JIA Zhi-xiang(贾志翔)2, WANG Li-jun(王丽君)2, WANG Yi-jun(王义君)2, HU Chang-yuan(胡长员)1, 2, HE Xiang-ming(何向明)1, 2
1. Jiangxi Key Laboratory of Surface Engineering, Jiangxi Science and Technology Normal University,Nanchang 330013, China;
2. School of Materials Science and Engineering, Jiangxi Science and Technology Normal University,Nanchang 330013, China;
3. School of Electronics, Jiangxi University of Finance and Economics, Nanchang 330013, China
Received 23 September 2009; accepted 30 January 2010
Abstract: Anodic coatings were obtained by micro-arc oxidation on AZ91HP magnesium alloys in a solution containing 10 g/L NaOH and 8 g/L phytic acid. The effects of electric parameters including frequency, final voltage, duty cycle and current density on the corrosion resistance of anodic coatings formed on the magnesium alloys were investigated by using an orthogonal experiment of four factors with three levels. The results show that the final voltage plays a main role on the coating properties. The orders of affecting corrosion resistance and coating thickness are separately ranked from high to low as, final voltage>duty cycle>current density>frequency and final voltage>current density>frequency>duty cycle. The final voltage influences the corrosion resistance of the anodized samples mainly by changing the surface morphology and coating thickness.
Key words: magnesium alloys; micro-arc oxidation; electric parameters; corrosion resistance
1 Introduction
Micro-arc oxidation (MAO), developed under the traditional anodization, is an effective method to improve the corrosion and wear resistance of the magnesium alloys. The coating properties depend on several factors, such as the compositions of the substrate[1], electric parameters[2-6], and concentrations and compositions of the electrolytes[4, 7]. The applied electric parameters have great influence on the properties of the resulted anodic coatings because they are related with the coating thickness, structure and surface morphologies[2-6].
So far, the effects of electric parameters on the coating properties were often studied by the conventional approach, namely, one factor is varied while at the same time, all the other factors are kept constant. The optimum conditions achieved by the conventional approach may not be a true optimum if the interactions between the factors are present[8]. In addition, because of several electric parameters such as treating time, current density, final voltage, frequency and duty cycle, a large number of experiments will be carried out to achieve the optimum conditions if the conventional approach is applied. The Taguchi method uses a special design of orthogonal arrays to study all the designed factors with a minimum of experiments at a relatively low cost[9].
Recently, an environmentally friendly process containing phytic acid (C6H18O24P6) was developed in MAO of magnesium alloys[10-11]. The influence of C6H18O24P6 concentration on the morphologies, compositions and corrosion resistance of anodic coatings was studied. The results showed that the coatings formed in the solution containing 8 g/L C6H18O24P6 exhibited the best pore uniformity and corrosion resistance[10]. In order to obtain the anodic coatings with excellent properties, it is significant to further investigate the effects of electric parameters on the coating properties. However, there is less report in this area.
In this work, the effects of electric parameters on the coating properties were studied by using an orthogonal experiment. The surface morphology was observed using an environmental scanning electron microscope (ESEM). The corrosion resistance of magnesium alloys after MAO was evaluated by immersion test in 3.5% NaCl for 24 h.
2 Experimental
An ingot of AZ91HP magnesium alloy was employed and its chemical compositions are as follows (mass fraction, %): Al 8.93, Zn 0.47, Mn 0.22, Si 0.03, Cu 0.002, Ni 0.001, Fe 0.001 and Mg balance.
Samples for MAO treatment were cut into 5 cm× 7 cm×1 cm and anodized after they were ground, washed, degreased and dried. The base solution was 10 g/L NaOH and 8 g/L C6H18O24P6.
The samples pretreated as above were anodized by MAO equipment (made in China), which consisted of a power supply, a stainless steel barrel and a stirring and cooling system. The power supply can provide a unipolar (positive) pulse or bipolar pulses (with a positive pulse and a negative pulse). In the experiment, the unipolar pulse was used under a constant current control mode. The frequency, final voltage and duty cycle of the power supply can be adjusted from 100 to 3 000 Hz, 80 to 800 V and 5% to 40%, respectively.
The coating thickness was measured by a TT230 current instrument, which was calibrated with the same base metal. The surface morphologies of the anodized samples were observed by a scanning electron microscope (SEM QUANTA 200) after they were washed, dried and coated with gold. The anodized samples were tested under immersion conditions in a 3.5% (mass fraction) NaCl solution to evaluate the corrosion performance. After 24 h, the samples were taken out from the solution, washed with distilled water, and dried by hot wind. The developed corrosion pits were calculated carefully by the grid method[12].
3 Results and discussion
3.1 Arrangement of orthogonal experiment and result analysis
In this experiment, the orthogonal experiment of four factors with three levels including frequency, final voltage, duty cycle and current density, was used to comprehensively investigate the effects of electric parameters on the corrosion resistance and coating thickness on AZ91HP magnesium alloys. The factors and levels of the orthogonal experiment are listed in Table 1.
Table 1 Factors and levels of orthogonal experiment
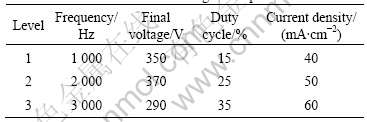
The orthogonal experimental array and experimental results are listed in Table 2.
It can be seen from Table 2 that among the nine samples, experiment No. 1, 4 and 8 achieve good corrosion resistance. In order to systematically investigate the effects of electric parameters on the corrosion resistance of the anodic coatings, the experimental data were treated by the method of intuitionistic analysis, which were described in detail in Refs.[2-3]. Based on the value of the differences, the rank of affecting factors of the corrosion resistance of
Table 2 Results of orthogonal experiment for corrosion resistance and coating thickness
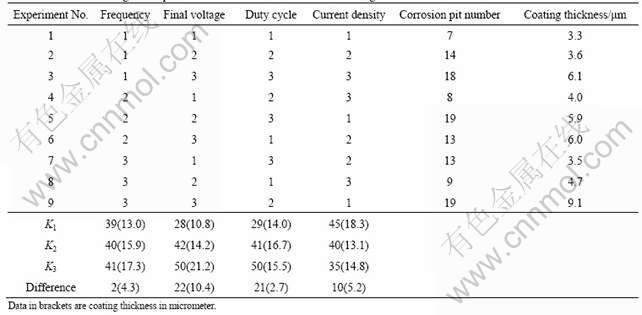
anodized samples from high to low is: final voltage>duty cycle>current density>frequency. The optimum processing parameters for the corrosion resistance are frequency 1 000 Hz, final voltage 350 V, duty cycle 15% and current density 50 mA/cm2.
Table 2 also shows that experiment No. 9 achieves the thickest coating among all the anodized samples. The data about coating thickness (listed in brackets in Table 2) are dealt with the same method as corrosion resistance and the order of influencing coating thickness from high to low is as final voltage>current density>frequency> duty cycle.
Fig.1 shows the effects of the four factors on the corrosion resistance of anodic coatings plotted based on the results in Table 2, such as the effect of frequency, effect of final voltage, effect of duty cycle and effect of current density.
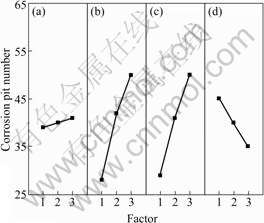
Fig.1 Effects of factors on corrosion resistance of anodic coatings: (a) Frequency; (b) Final voltage; (c) Duty cycle; (d) Current density
It can be seen from Fig.1 that with the increase of final voltage and duty cycle, the number of corrosion pits increases, which indicates the decrease of corrosion resistance. However, with the increase of current density, the corrosion resistance is improved.
The effects of the four factors on the coating thickness are shown in Fig.2. It can be seen from Fig.2 that the most important affecting factor on the coating thickness is the final voltage. With the increase of final voltage, the coating thickness increases greatly. Other factors, such as frequency, duty cycle and current density have minor effects on the coating thickness.
3.2 Influence of final voltage on coating morphology and compositions
Figs.1 and 2 show that the final voltage is an important factor affecting the coating properties. The final voltage not only greatly improves the coating thickness, but also influences the corrosion resistance considerably. Table 2 shows that experiment No. 3, 6 and 9, which are obtained by the highest final voltage, achieve thick anodic coatings but poor corrosion resistance.
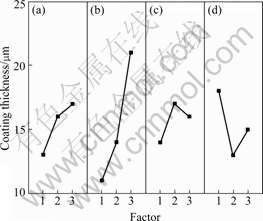
Fig.2 Effects of factors on coating thickness: (a) Frequency; (b) Final voltage; (c) Duty cycle; (d) Current density
The final voltage can affect the compositions, thickness, morphologies and corrosion resistance of anodic coatings[2, 6]. In the experiment, how does the final voltage affect coating morphologies and compositions is essential to be studied in these areas.
The surface morphologies of anodic coatings formed at different voltages are shown in Fig.3. It can be seen from Fig.3(a) that the pore size of the anodic coatings obtained at final voltage of 350 V is about 1 μm. The micro cracks can be seen on the coating surface. These cracks form during the cooling of the anodic film, due to the difference in the thermal coefficient of expansion between the alloy and the oxide film[13]. With the increase of final voltage, the pore size becomes larger (see Figs.3(b) and 3(c)). In addition, the coating surface becomes rougher, especially as shown in Fig.3(c).
EDX analysis shows that the anodic coatings contain C, O, Mg, Al and P. Element P in the coatings is attributed to C6H18O24P6, which indicates that C6H18O24P6 takes part in the coating formation.
The chemical compositions of the anodic coatings obtained at different voltages don not change significantly (see Table 3), which indicates that the final voltage affects the corrosion resistance mainly by
Table 3 Chemical compositions of anodic coatings obtained at different final voltages (molar fraction, %)
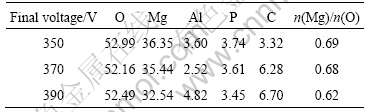
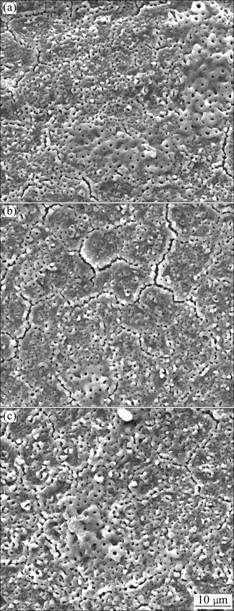
Fig.3 Surface morphologies of AZ91HP alloys anodized to three voltages of 350 V (a), 370 V (b) and 390 V (c) under frequency 1 000 Hz, duty cycle 15% and current density 60 mA/cm2
changing the coating thickness and surface morphology.
In the MAO process, anions in the solution such as phytic acid ions and OH- move to the anode under the electric field. When the phytic acid ions and OH- ions arrive at the anode/electrolyte interface, they will combine with positively charged metal ions to form compounds. As the main kind of anions on the anode, magnesium ions will react with the phytic acid ions to produce the insoluble magnesium phytate[14] and hydroxide[15] according to reactions as follows:
C6H18O24P6+Mg2++H2O→C6H6O24P6Mg6+2H3O (1)
Mg2++OH-→Mg(OH)2 (2)
Under an extremely high thermal energy generated by sparks, the hydroxide was dehydrated to form an oxide compound according to the following reaction:
Mg(OH)2→MgO+H2O (3)
Previous results have shown that anodic coatings formed in the C6H18O24P6 containing solution consisted of MgO[11]. The mole ratio of Mg to O in magnesium phytate is 0.25, while the value in MgO is 1.00. With the increase of final voltage, the molar ratio of Mg to O in the coatings slightly decreases, which might be resulted from the larger content of C6H6O24P6Mg6 in the coatings.
The corrosion resistance of anodic coatings depends on a lot of factors such as the coating composition[7], thickness[2] and surface morphology[1]. The results show that the preparation of anodic coatings with higher chemical stability compounds offers an opportunity to produce layers that could provide better corrosion protection[7]. In addition, the corrosion resistance of the anodized samples is improved with the coating thickening under the constant coating porosity[2], but decreases with increasing coating porosity[1].
In the experiment, with the increase of final voltage, the coating thickness and pore size of anodic coatings increase. The chemical compositions of the anodic coatings have minor changes at different voltages, which indicates that the final voltage affects the corrosion resistance mainly by changing the coating thickness and surface morphology. At higher final voltage, although the coating thickness increases, the diameter of micropores on the coatings increases. Therefore, in the corrosive environment, the corrosive medium can easily penetrate the pores and reaches the substrate, which decreases the corrosion resistance of the anodized samples.
4 Conclusions
1) The affecting orders of factors on the corrosion resistance and thickness of anodic coatings from high to low are ranked as: final voltage>duty cycle>current density>frequency and final voltage>current density> frequency>duty cycle, respectively.
2) With the increase of final voltage, the porosity and the thickness of anodic coatings increase.
3) The final voltage influences the corrosion resistance mainly by changing the surface morphology and the thickness of anodic coatings.
References
[1] SHI Z M, SONG G L, ATRENS A. Influence of the β phase on the corrosion performance of anodised coatings on magnesium- aluminium alloys [J]. Corrosion Science, 2005, 47: 2760-2777.
[2] ZHANG R F, SHAN D Y, CHEN R S, HAN E H. Effects of electric parameters on properties of anodic coatings formed on magnesium alloys [J]. Materials Chemistry and Physics, 2008, 107: 356-363.
[3] MA Y, NIE X, NORTHWOOD D, HU H. Systematic study of the electrolytic plasma oxidation process on a Mg alloy for corrosion protection [J]. Thin Solid Films, 2006, 494: 296-301.
[4] CHAI L Y, YU X, YANG Z H, WANG Y Y, OKIDO M. Anodizing of magnesium alloy AZ31 in alkaline solutions with silicate under continuous sparking [J]. Corrosion Science, 2008, 50: 3274-3279.
[5] SHI Z M, SONG G L, ATRENS A. Influence of the anodising current on the corrosion resistance of anodised AZ91D magnesium alloy [J]. Corrosion Science, 2006, 48: 1939-1959.
[6] MU W Y, HAN Y. Characterization and properties of the MgF2/ZrO2 composite coatings on magnesium prepared by micro-arc oxidation [J]. Surface and Coatings Technology, 2008, 202: 4278-4284.
[7] LIANG J, SRINIVASAN P B, BLAWERT C, DIETZEL W. Comparison of electrochemical corrosion behaviour of MgO and ZrO2 coatings on AM50 magnesium alloy formed by plasma electrolytic oxidation [J]. Corrosion Science, 2009, 51: 2483-2492.
[8] SRINIVASAN C B, NARASIMHAN N L, ILANGO S V. Development of rapid-set high-strength cement using statistical experimental design [J]. Cement and Concrete Research, 2003, 33: 1287-1292.
[9] MA Y Y, HU H, NORTHWOOD D, NIE X Y. Optimization of the electrolytic plasma oxidation processes for corrosion protection of magnesium alloy AM50 using the Taguchi method [J]. Journal of Materials Processing Technology, 2007, 182: 58-64.
[10] ZHANG R F, ZHANG S.F, DUO S.W. Influence of phytic acid concentration on coating properties obtained by MAO treatment on magnesium alloys [J]. Applied Surface Science, 2009, 255: 7893-7897.
[11] ZHANG R.F, XIONG G Y, HU C Y. Comparison of coating properties obtained by MAO on magnesium alloys in silicate and phytic acid electrolytes [J]. Current Applied Physics, 2010, 10: 255-259.
[12] SHI Z M, SONG G L, ATRENS A. The corrosion performance of anodized magnesium alloys [J]. Corrosion Science, 2006, 48: 3531-3546.
[13] VERDIER S, BOINET M, MAXIMOVITCH S, DALARD F. Formation, structure and composition of anodic films on AM60 magnesium alloy obtained by DC plasma anodising [J]. Corrosion Science, 2005, 47: 1429-1444.
[14] NOTOYA T, OTIENO-ALEGO V, SCHWEINSBERG D P. The corrosion and polarization behaviour of copper in domestic water in the presence of Ca, Mg, and Na-salts of phytic acid [J]. Corrosion Science, 1995, 37: 55-65.
[15] HSIAO H Y, TSAI W T. Characterization of anodic films formed on AZ91D magnesium alloy [J]. Surface and Coatings Technology, 2005, 190: 299-308.
(Edited by LONG Huai-zhong)
Foundation item: Projects(GJJ08363, GJJ09573) supported by the Scientific Research Fund of Jiangxi Provincial Education Department, China
Corresponding author: ZHANG Rong-fa; Tel: +86-791-3831266; E-mail: rfzhang-10@163.com