J. Cent. South Univ. Technol. (2011) 18: 1371-1376
DOI: 10.1007/s11771-011-0848-6
Surfactant-assisted synthesis and magnetic properties of monodispersed manganese ferrite nanocrystals
SHI Rong-rong(史蓉蓉), QIU Guan-zhou(邱冠周), LIU Xiao-he(刘小鹤)
Department of Inorganic Materials, School of Minerals Processing and Bioengineering, Central South University, Changsha 410083, China
? Central South University Press and Springer-Verlag Berlin Heidelberg 2011
Abstract: Monodispersed manganese ferrite (MnFe2O4) nanocrystals could be successfully synthesized in large quantities via a facile synthetic technique based on the pyrolysis of organometallic compound precursor, in which octadecene was used as solvent, and oleic acid and oleylamine were used as capping ligands. MnFe2O4 nanocrystals were obtained with size in a tunable range of 4- 15 nm and their morphologies could be tuned from spherical to triangle-shaped by varying the surfactants. The phase structure, morphology, and size of the products were characterized in detail by X-ray diffraction (XRD) and transmission electron microscopy (TEM). Magnetic properties of MnFe2O4 nanocrystals with different morphologies were measured using a superconducting quantum interference device (SQUID). Both monodisperse MnFe2O4 nanocrystals with spherical and triangle-shapes are superparamagnetic at room temperature while ferromagnetic at 2 K. The pyrolysis method may provide an effective route to synthesize other spinel ferrites or metal oxides nanocrystals.
Key words: pyrolysis; monodisperse nanocrystal; manganese ferrite; magnetic properties
1 Introduction
Monodispersed ferrites have shown great potential applications, ranging from point-of-use water purification, electronic devices to medical diagnostics and drug delivery [1-3]. Especially for biomedical applications, magnetic nanocrystals are required to have a well-defined composition, controlled size or shape, density of reactive surface groups, and dispersibility in desired solvents because these features control effectively their intrinsic chemical and physical properties. During the past decades, various synthetic methods were continually improved for the controlled synthesis of magnetic nanocrystals. Among these methods, the most versatile strategy has been based on the decomposition of molecular precursors in high-boiling organic solvents. In a typical reaction, a metalorganic precursor, such as cupferronate [4], carbonyl [5], acetylacetonate [6], or fatty metal salt [7], is dissolved in long-chain ether [8], amine [9], or alkene [10] and thermally decomposed in the presence of a surface-capping agent, such as amine or a carboxylic acid [11]. The thermolysis reaction is performed between 200 °C and 300 °C under a protective atmosphere that prevents the oxidation of the transition-metal ions and the formation of unwanted secondary phases [12-13].
HYEON’s group reported the direct synthesis of monodisperse ferrite nanocrystals via thermal decomposition of metal-surfactant complexes followed by mild chemical oxidation [14]. Similarly, ZENG and co-workers produced monodisperse ferrite nanocrystals via a high-temperature solution phase reaction of metal acetylacetonate with 1,2-hexadicanediol in the presence of surfactants [15]. Recently, ADIREDDY et al obtained monodisperse ferrite nanocrystals by the thermolysis of transition-metal acetates in oleyl alcohol solutions under aerobic conditions [16]. On the basis of our previous works [11,17], herein, monodisperse MnFe2O4 nano- crystals with narrow size distribution and different morphologies were synthesized. The synthetic strategy presented here may have a good prospect for large scale application and provide an effective route to synthesize different spinel ferrites or other metal oxides nanocrystals with tunable shape and size. Monodisperse MnFe2O4 nanocrystals obtained with cheap or nontoxic agents and tunable shape are the important advantageous features reported here.
2 Experimental
The synthesis was handled under standard airless techniques in an argon atmosphere. All chemicals used in this experiment, such as iron(III) acetylacetonate (Fe(acac)3), manganese(III) acetylacetonate (Mn(acac)3), hexadecyl trimethyl ammonium bromide (CTAB), sodium oleate, polyvinyl pyrrolidone (PVP) and sodium dodecyl sulfate (SDS) were of analytical grade and used without any further purification. Octadecene (ODE, 90%, mass fraction), oleic acid and oleylamine were purchased from Acros.
A typical synthetic procedure was as follows: iron acetylacetonate (0.353 2 g, 1 mmol) and manganese acetylacetonate (0.128 6 g, 0.5 mmol) were weighed out accurately and charged into a reaction flask. Octadecene (20 mL), oleic acid (1.2 mL, 4 mmol) and oleylamine (3.3 mL, 10 mmol) were added to the reaction mixture with stirring. The mixture was degassed for 10 min at room temperature using high purity argon gas (Ar2). The mixture solution was firstly heated to 200 °C for 30 min, and then to 260 °C for 2 h before cooling down to room temperature by removing the heat source. The product was precipitated with ethanol and hexane followed by centrifugation, and uniform MnFe2O4 nanocrystals were obtained. Different surfactants, molar ratios of oleic acid to oleylamine and injection approaches were discussed in the present work. And other samples were prepared by the similar approach with changing certain conditions.
Obtained specimens were characterized on a D/max2550 VB+ X-ray powder diffractometer (XRD) with Cu Kα radiation (λ=0.154 178 nm). The operation voltage and current were kept at 40 kV and 40 mA, respectively. The size and morphology of the as-synthesized products were determined by a JEM-200CX transmission electron microscope (TEM) at 160 kV. Magnetic measurements were conducted using a Quantum Design MPMS XL-5 superconducting quantum interference device (SQUID).
3 Results and discussion
X-ray diffraction (XRD) was carried out to determine the chemical composition and crystallinity of the as-prepared products. Figure 1 shows the typical XRD pattern of MnFe2O4 nanocrystals synthesized with molar ratio of 4:10 for oleic acid to oleylamine and reacted firstly at 200 °C for 30 min and then 260 °C for 2 h. All diffraction peaks in this pattern can be readily indexed to cubic spinel structure of MnFe2O4 with calculated lattice parameter of 0.848 nm, which agrees well with the diffraction pattern (data from JCPDS file 74-2403). Similar XRD patterns for the products prepared under different conditions were also obtained, which can be readily indexed to spinel structure of MnFe2O4. No other impurity phase was observed, indicating pure MnFe2O4 nanocrystals were synthesized
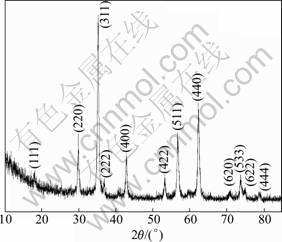
Fig.1 XRD pattern of MnFe2O4 nanocrystals prepared with molar ratio of 4:10 for oleic acid to oleylamine and reacted at 200 °C for 30 min and then 260 °C for 2 h
under the current mild conditions.
Figure 2 shows the TEM image of MnFe2O4 nanocrystals prepared with a molar ratio of 4:10 for oleic acid to oleylamine and incubated at 200 °C for 30 min and then 260 °C for 2 h. It reveals that a large number of well-defined MnFe2O4 nanocrystals have been successfully synthesized under the current conditions, and the particles are uniform with mean size about 14 nm. The high-magnification TEM image (see Fig.2(b)) illustrates that the overall inorganic diameter is 14 nm, which is calculated statistically from sixty particles selected from the TEM image. And well-aligned two dimension (2D) self-assembly demonstrates the uniformity of the product nanocrystals.
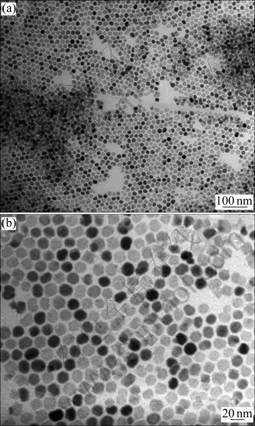
Fig.2 TEM images of MnFe2O4 nanocrystals synthesized with molar ratio of 4:10 for oleic acid to oleylamine and recuted at 200 °C for 30 min and then 260 °C for 2 h: (a) Low magnification; (b) Higher magnification
How to reveal the formation of monodisperse nanocrystals is always very interesting and challenging for materials scientists. LAMER and his colleagues pioneered the concept of “burst nucleation” [18]. In this progress, many nuclei are generated at the same time, and then these nuclei start to grow without additional nucleation. The “burst nucleation” process makes it possible to control the size distribution of the ensemble of particles as a whole during growth and has been adopted as an important concept in the synthesis of monodisperse nanocrystals. As a synthetic strategy, this method is often referred to as “the separation of nucleation and growth” [19]. “Hot-injection” technique is one of the most efficient methods to produce “burst nucleation”, which has been widely used to synthesize nanocrystals of metal chalcogenides [20], transition metals [21], and noble metals [22]. In this work, the approach of rapid injection of reagents into hot surfactant solution is utilized to achieve the purpose of separating nucleation and growth. Figure 3 shows the TEM images of the as-synthesized MnFe2O4 nanocrystals injected with 4 mmol oleic acid and 10 mmol oleylamine at different temperatures. When oleic acid and oleylamine were injected at 120 ?C and then raised to 260 ?C for 2 h, the morphology of the obtained MnFe2O4 sample is shown in Fig.3(a). Overall, the particle size of MnFe2O4 samples is smaller than that of samples prepared without injection approach and the mean size of the particles in square areas is 4 nm calculated with the selected particles in special regions. This result demonstrates that injection technique can separate the nucleation and growth of the crystals efficiently. The injection at lower temperature (120 ?C) can inhibit the growth of the grains even at the same incubating temperature (260 ?C) and reaction time (2 h). When increasing the injection and incubation temperature point to 200 ?C and 300 ?C, respectively, the morphology of the as-synthesized MnFe2O4 samples is shown in Fig.3(b). It can be concluded that higher- temperature injection broadens the deviation of the particle size distribution. At the same time, the mean size of the particle is also increased. In the experiment, oleylamine acts as reductant and can accelerate the decomposition of precursor acetylacetonate salts. Injection of oleic acid and oleylamine at higher temperature delays the nucleation time of product crystals, which will make the process of nucleation and growth occur simultaneously and also promote the second stage of growth. Furthermore, it is difficult to organize into 2D assembly with wide size distribution. In this regard, synthesis approach has great influence on particle size and appropriate injection temperature is beneficial for controlling particle size and its deviation.
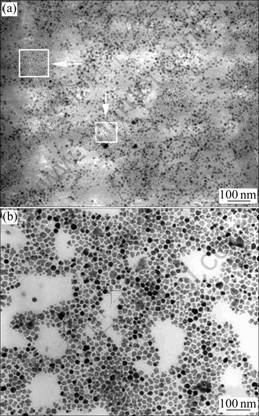
Fig.3 TEM images of as-synthesized MnFe2O4 nanocrystals: (a) Injected with 4 mmol oleic acid and 10 mmol oleylamine at 120 ?C and then raise to 260 ?C for 2 h; (b) Injected with 4 mmol oleic acid and 10 mmol oleylamine at 200 ?C react for 2 h and then raise to 300 ?C for 1 h
In addition, the influence of surfactants on the morphology and size of MnFe2O4 nanocrystals is investigated. An equal amount of surfactants, such as CTAB, PVP, SDS and sodium oleate, was used to synthesize MnFe2O4 nanocrystals prepared at 200 °C for 30 min and then 260 °C for 2 h with molar ratio of 4:10 for oleic acid to oleylamine. Figure 4(a) shows the TEM image of the as-synthesized MnFe2O4 nanocrystals with 0.1 mmol CTAB. The products display irregular spherical nanocrystals accompanied with triangles and quadrilateral shapes. TEM image of products obtained in the case of 0.03 g PVP is shown in Fig.4(b), which has uniform size with the mean diameter of 14.5 nm, as shown in its particle core size histogram (inset of Fig.4(b)). In Fig.4(c), quasi-cubic particles can be seen when 0.1 mmol SDS is used. And the triangle nanocrystals are obtained with 0.1 mmol sodium oleate used as surfactant, as shown in Fig.4(d). This reveals that surfactant molecules can modulate the growth rate along certain directions with high surface energy, which results in a variety of nanocrystals shapes [23]. However, in our previous work, surfactants facilitate the formation of triangle and rod-like shapes in the synthesis process of iron oxides. For example, a proper amount of CTAB surfactant often induces the synthesis of triangle Fe3O4 nanocrystals [11]. Here, MnFe2O4 nanocrystals with triangle morphology are prepared with sodium oleate surfactant. It is much more difficult to elucidate the mechanism for shape transformation decided by surfactant in detail, but the reactant metal ions may play certain roles according to our experimental result.
The molar ratio of oleic acid to oleylamine is also a crucial factor for the production of monodisperse MnFe2O4 nanocrystals. Different ratios of oleic acid to oleylamine are attempted, and 4:10 molar ratio of oleic acid to oleylamine is the most proper ratio. Figure 5 demonstrates the effect of molar ratio of oleic acid to oleylamine on the as-obtained products. On one hand, by increasing the amount of oleylamine to 16 mmol, a large number of nanoparticles with diameter less than 10 nm were obtained, as shown in Fig.5(a). Increasing the concentration of oleylamine would accelerate the decomposition rate of precursor acetylacetonate salts, which leads to the rapid consumption of the monomers and the subsequent termination of the nucleation process, so that nucleation can be separated from growth and smaller particles can be obtained. On the other hand, when the molar ratio of oleic acid to oleylamine is reduced to 1:10, the products display irregular flakes, as shown in Fig.5(b). In pyrolysis process, oleic acid is commonly used as capping ligand to stabilize magnetic nanoparticles, and studies have proved that strong chemical bond is formed between the carboxylic acid and the amorphous iron oxide nanocrystals [24]. In this sense, decreasing the amount of oleic acid might lead to aggregation of magnetic nanocrystals for reducing its high surface energy.
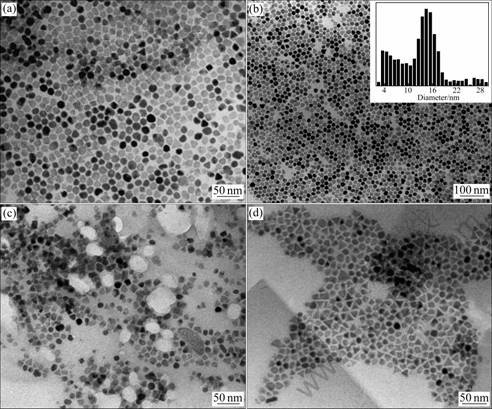
Fig.4 Effect of surfactants on morphologies and sizes of MnFe2O4 nanocrystals prepared with molar ratio of 4:10 for oleic acid to oleylamine at 200 °C for 30 min and then 260 °C for 2 h: (a) 0.1 mmol CTAB; (b) 0.03 g PVP; (c) 0.1 mmol SDS; (d) 0.1 mmol sodium oleate (Inset shows particle core size histogram of Fig.3(b))
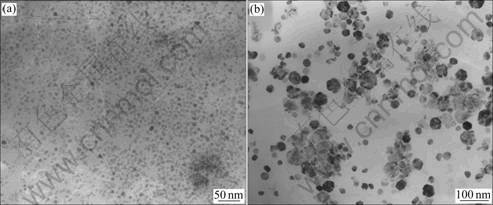
Fig.5 Effects of different molar ratios of oleic acid to oleylamine on morphology of MnFe2O4 nanocrystals: (a) 4:16; (b) 1:10
Magnetic properties of MnFe2O4 nanocrystals with different morphologies were measured by a super- conducting quantum interference device. Figures 6(a) and (c) show the plot of the magnetization versus temperature at an applied magnetic field of 7.96×103 A/m for the spherical and triangle shaped MnFe2O4 nanocrystals prepared with PVP and sodium oleate, respectively. For triangle-shaped MnFe2O4 nanocrystals, the ZFC curve shows a maximum of the magnetization at T=230 K (see Fig.6(c)). MnFe2O4 products display superparamagnetic performance above the blocking temperature (TB) where the anisotropy energy barrier equals the thermal energy. Unlike the triangle-shaped products, no maximum is detected in ZFC curve of spherical MnFe2O4 nanocrystals (see Fig.6(a)), indicating that, in this case, the blocking temperature is equal to or above 300 K. The magnetization of spherical MnFe2O4 is close to 9.2 A·m2/kg, whereas that of the triangle shaped MnFe2O4 nanocrystals is 16.5 A·m2/kg. The hysteresis loops of the as-prepared MnFe2O4 nanocrystals with different morphologies are shown in Figs.6(b) and 6(d), respectively. At 300 K, both spherical and triangle-shaped MnFe2O4 nanocrystals are superparamagnetic, with zero remanence and a very low coercivity. The saturation magnetizations of spherical and triangle-shaped MnFe2O4 nanocrystals are 43.4 and 57.9 A·m2/kg, respectively. The lower value of saturation magnetization suggests a higher degree of surface disorder in the case of spherical MnFe2O4 nanocrystals as compared to the triangle-shaped ferrite. At 2 K, both spherical and triangle-shaped MnFe2O4 nanocrystals are ferromagnetic, with coercivities of Hc=1.35×104 A/m for spherical MnFe2O4 and 3.34×104 A/m for triangle-shaped MnFe2O4. The higher coercivity indicates that triangle- shape has prominent magnetic anisotropy [13].
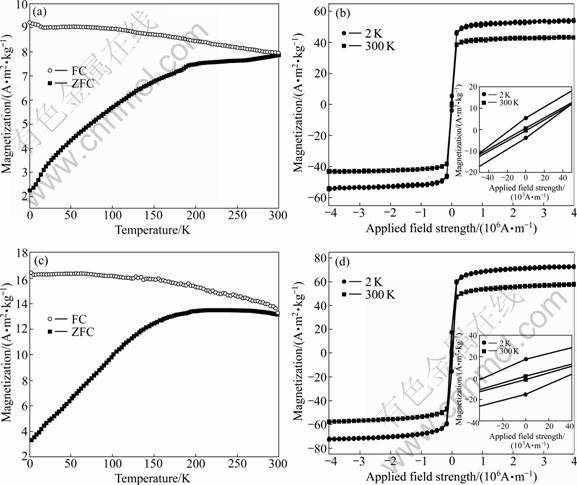
Fig.6 ZFC and FC ((a) and (c)) curves and hysteresis loops ((b) and (d)) of as-prepared MnFe2O4 nanocrystals with different shapes measured under applied magnetic field of 7.96×103 A/m at 2 K and 300 K: (a)-(b) Monodispersed MnFe2O4 nanocrystals prepared with 0.03 g PVP; (c)-(d) Triangle-shaped MnFe2O4 nanocrystals prepared with 0.1 mmol sodium oleate (Insets are enlarged hysteresis loops)
4 Conclusions
1) Manganese ferrite nanocrystals with different shapes are successfully synthesized via a thermal decomposition route with octadecene as a solvent and oleic acid and oleylamine as capping ligands.
2) The shape of final products could be readily tuned from sphere to triangle or quadrilateral shapes by adjusting the surfactants. Spherical MnFe2O4 nano- crystals can be obtained by adding PVP to the reaction and triangle-shaped products are obtained with the aid of sodium oleate.
3) Both MnFe2O4 nanocrystals with spherical and triangle-shapes exhibit superparamagnetic behavior at room temperature with the saturation magnetizations of 43.4 A·m2/kg and 57.9 A·m2/kg, while ferromagnetic behavior at 2 K with the coercivities of 1.35×104 A/m and 3.34×104 A/m and for spherical triangle MnFe2O4, respectively.
4) The formation mechanism of monodisperse MnFe2O4 nanocrystals can be elucidated by the concept of “burst nucleation”.
References
[1] RAJ K, MOSKOWITZ R. Commercial applications of ferrofluids [J]. J Magn Magn Mater, 1990, 85(1/2/3): 233-235.
[2] HYEON T. Chemical synthesis of magnetic nanoparticle [J]. Chem Commun, 2003(8): 927-934.
[3] XU Chen-jie, SUN Sou-heng. Monodisperse magnetic nanoparticles for biomedical applications [J]. Polym Int, 2007, 56(7): 821-826.
[4] GHOSH M, BISWAS K, SUNDARESAN A, RAO C N R. MnO and NiO nanoparticles: Synthesis and magnetic properties [J]. J Mater Chem, 2006, 16(1): 106-111.
[5] SUN Shou-heng, MURRAY C B, WELLER D, FOLKS L, MOSER A. Monodisperse FePt nanoparticles and ferromagnetic FePt nanocrystal superlattices [J]. Science, 2000, 287(5460): 1989-1992.
[6] LI Y, AFZAAL M, O’BRIEN P. The synthesis of amine-capped nagnetic (Fe,Mn,Co,Ni) oxide nanocrystals and their surface modification for aqueous dispersibility [J]. J Mater Chem, 2006, 16(22): 2175-2180.
[7] JANA N R, CHEN Yong-fen, PENG Xiao-gang. Size- and shape- controlled magnetic (Cr,Mn,Fe,Co,Ni) oxide nanocrystals via a simple and general approach [J]. Chem Mater, 2004, 16(20): 3931-3935.
[8] SUN Shou-heng, ZENG H. Size-controlled synthesis of magnetite nanoparticles [J]. J Am Chem Soc, 2002, 124(28): 8204-8205.
[9] SEO W S, JO H H, LEE K, KIM B, OH S J, PARK J T. Size-dependent magnetic properties of colloidal Mn3O4 and MnO nanoparticles [J]. Angew Chem Int Ed, 2004, 43(9): 1115-1117.
[10] YU W W, FALKNER J C, YAVUZ C T, COLVIN V L. Synthesis of monodisperse iron oxide nanocrystals by thermal decomposition of iron carboxylate salts [J]. Chem Commun, 2004(20): 2306-2307.
[11] SHI Rong-Rong, GAO Guan-Hua, YI R, ZHOU Ke-Chao, QIU Guan-Zhou, LIU Xiao-He. Controlled synthesis and characterization of monodisperse Fe3O4 nanoparticles [J]. Chin J Chem, 2009, 27(4): 739-744.
[12] CROUSE C A, BARRON A R. Reagent control over the size, uniformity, and composition of Co-Fe-O nanoparticles [J]. J Mater Chem, 2008, 18(35): 4146-4153.
[13] XIE J, PENG S, BROWER N, POURMAND N, WANG S X, SUN Shou-Heng. One-pot synthesis of monodisperse iron oxide nanoparticles for potential biomedical applications [J]. Pure Appl Chem, 2006, 78(5): 1003-1014.
[14] KANG E, PARK J, HWANG Y, KANG M, PARK J G, HYEON T. Direct synthesis of highly crystalline and monodisperse manganese ferrite nanocrystals [J]. J Phys Chem B, 2004, 108(37): 13932-13935.
[15] ZENG H, RICE P M, WANG S X, SUN Shou-heng. Shape- controlled synthesis and shape-induced texture of MnFe2O4 nanoparticles [J]. J Am Chem Soc, 2004, 126(37): 11458-11459.
[16] ADIREDDY S, LIN Cui-kun, PALSHIN V, DONG Yu-ming, COLE R, CARUNTU G. Size-controlled synthesis of quasi-monodisperse transition-metal ferrite nanocrystals in fatty alcohol solutions [J]. J Phys Chem C, 2009, 113(49): 20800-20811.
[17] SHI Rong-rong, LIU Xiao-he, GAO Guan-hua, YI R, QIU Guan-zhou. Large-scale synthesis and characterization of monodisperse Fe3O4 nanocrystals [J]. J Alloys Compd, 2009, 485(1/2): 548-553.
[18] LAMER V K, DINEGAR R H. Theory, production and mechanism of formation of monodisperse hydrosols [J]. J Am Chem Soc, 1950, 72(11): 4847-4854.
[19] PENG Z A, PENG Xiao-gang. Nearly monodisperse and shape-controlled CdSe nanocrystals via alternative routes: nucleation and growth [J]. J Am Chem Soc, 2002, 124(13): 3343-3353.
[20] TALAPIN D V, ROGACH A L, KORNOWSKI A, HAASE M, WELLER H. Highly luminescent monodisperse CdSe and CdSe/ZnS nanocrystals synthesized in a hexadecylamine-trioctylphosphine oxide-trioctylphospine mixture [J]. Nano Lett, 2001, 1(4): 207-211.
[21] HAMBROCK J, BECKER R, BIRKNER A, WEIB J, FISCHER R A. A non-aqueous organometallic route to highly monodispersed copper nanoparticles using [Cu(OCH(Me)CH2NMe2)2] [J]. Chem Commun, 2002(1): 68-69.
[22] JANA N R, PENG Xiao-gang. Single-phase and gram-scale route toward nearly monodisperse Au and other noble metal nanocrystals [J]. J Am Chem Soc, 2003, 125(47): 14280-14281.
[23] JUN Y, CHOI J, JINWOO C. Shape control of semiconductor and metal oxide nanocrystals through nonhydrolytic colloidal routes [J]. Angew Chem Int Ed, 2006, 45(21): 3414-3439.
[24] ZHANG L, HE R, GU Hong-Chen. Oleic acid coating on the monodisperse magnetic nanoparticles [J]. Appl Surf Sci, 2006, 253(5): 2611-2617.
(Edited by HE Yun-bin)
Foundation item: Project(2010QZZD008) supported by the Prospect Key Projects of Fundamental Research Funds for the Central Universities; Project (2007FJ3008) supported by the Hunan Provincial Key Science and Technology Program of China
Received date: 2010-05-12; Accepted date: 2011-03-07
Corresponding author: LIU Xiao-he, Associate Professor, PhD; Tel: +86-731-88830543; E-mail: liuxh@mail.csu.edu.cn