Trans. Nonferrous Met. Soc. China 22(2012) 2726-2729
Influence of pyrolysis temperature on structure and dielectric properties of polycarbosilane derived silicon carbide ceramic
DING Dong-hai1, 2, ZHOU Wan-cheng1, ZHOU Xuan1, LUO Fa1, ZHU Dong-mei1
1. State Key Laboratory of Solidification Processing, Northwestern Polytechnical University, Xi’an 710072, China;
2. College of Materials and Mineral Resources, Xi’an University of Architecture and Technology, Xi’an 710055, China
Received 17 October 2011; accepted 16 January 2012
Abstract: β-SiC ceramic powders were obtained by pyrolyzing polycarbosilane in vacuum at 800-1200 °C. The β-SiC ceramic powders were characterized by TGA/DSC, XRD and Raman spectroscopy. The dielectric properties of β-SiC ceramic powders were investigated by measuring their complex permittivity by rectangle wave guide method in the frequency range of 8.2-18 GHz. The results show that both real part ε′ and imaginary part ε″ of complex permittivity increase with increasing pyrolysis temperature. The mechanism was proposed that order carbon formed at high temperature resulted in electron relaxation polarization and conductance loss, which contributes to the increase in complex permittivity.
Key words: silicon carbide ceramic; polycarbosilane derived SiC; dielectric properties; pyrolysis temperature; free carbon; complex permittivity
1 Introduction
The needs for protecting people or devices from electromagnetic wave pollution and for keeping military vehicle from being detected by microwave radar result in an growing investigation of microwave absorbing materials [1]. In the case of dielectric absorber, the complex permittivity (εr=ε′-jε″) is a critical parameter that determines the absorbing properties of absorber. SiC powders have attracted much attention as a dielectric absorber for microwave because of its adjustable electric properties and excellent high temperature stability. By far, much effort has bee donated to investigate the complex permittivity of doped SiC powders. ZHAO et al [2] and JIAO et al [3] prepared nanometer N-doped SiC powders by laser-induced gas phase reaction method and by chemical vapor deposition method, respectively. SU et al [4] and LI et al [5] synthesized B-doped and Al-doped powders by the combustion synthesis and solid-state reaction, respectively. Also, JIN et al [6] prepared Al-doped SiC powder by microwave synthesis via reaction of fine powders of Si, C and Al under Ar atmosphere. In addition, Ni-doped SiC powders were successfully produced using the mechanically activated self-propagating high temperature synthesis method by LI et al [7]. Generally, the doped SiC powders have the better microwave dielectric loss in the frequency range of 8.2-12.4 GHz due to the n-type doping of N/Ni or p-type doping of Al /B.
In the past several decades, much attention has been focused on the polymer derived ceramics (PDCs) including SiC, SiCN, SiCO, Si3N4, SiCNO, BN, AlN and so on [8]. Generally, PDCs show excellent high-temperature structural properties such as oxidation and creep resistance, and are always used as thermal structural materials in form of fibers, composites, coatings or porous ceramic. Among PDCs, SiC fibers [9,10] and SiC matrix [11] derived from polycarbosilane (PCS) are known well in SiCf/SiC and Cf/SiC composites. Besides mechanical properties, the electrical properties of SiC derived from PCS have been reported recently [12-14]. In this work, SiC powders were prepared by pyrolysis of PCS in vacuum at 800-1200 °C. We investigate the complex permittivity of PCS derived SiC (PCS-SiC) powders for the following reasons. Firstly, preceramic polymer pyrolysis route process requires lower temperature compared with combustion synthesis, solid-state reaction and thermal diffusion. Secondly, recent investigations have shown that the polymer derived ceramics including SiC possess semi-conductor characteristic due to their special nano-structure, so the dielectric loss of PCS-SiC ceramics may be used as absorber. Thirdly, although there are some reports on the electrical property of PDCs, the relationship between the complex permittivity and the processing parameters of PDCs is not known well up to date. Thus, the purpose of this work is to study the effect of process temperature on the complex permittivity of PCS-SiC in a frequency range of 8.2-18 GHz at room temperature. In order to understand the relation between the dielectric property and the process temperature, XRD and Raman spectroscopy were used to characterize the microstructure of the PCS-SiC powders.
2 Experimental
PCS powders with relative molecular mass of ~1800 and softening point of ~210 °C were provided by the National University of Defense Technology (China). The as-received PCS powders were analyzed by TGA and DSC. A heating rate of 5 °C/min was applied up to 1150 °C.
For measurement of the complex permittivity, the PCS powders were heated at a rate of 5 °C/min in a vacuum sintering furnace (ZRS-150, Sante, Jinzhou, China) up to 800, 900, 1000, 1100, 1200 °C, separately, and then held for 2 h. The cooling rate was about 2 °C/min. During the heating, holding and cooling processes, the chamber pressure was lowered to 0.1 Pa using a rotary pump. After pyrolyzing PCS powders at 800-1200 °C, black expanded products were obtained. This highly porous structure is due to the loss of organic oligomers and gaseous by-products in pyrolysis process. The pyrolytic products were ground to get powders with diameter of about 10 μm for subsequent characterization. The crystalline phases of the as-prepared powders were identified by XRD (X′Pert PRO MPD, PANalytical, Almelo, the Netherlands). A Raman spectrometer (LabRAM HR800) was utilized with the 514.5 nm line of an argon-ion laser as the excitation source for spectroscopic measurements. The ε′ and ε″ of the complex permittivity were tested by the rectangle wave guide technique with mode TE10 in the frequency range of 8.2-18 GHz (X band and Ku band), using a PNA network analyzer (E8362B, Agilent Technologies, Palo Alto, CA). The samples for testing the complex permittivity were prepared by mixing the powders with paraffin, whose ε′ and ε″ are 2.26 and 0, in a mass ratio of 1:1, and then the mixtures were molded into a brass flange to fabricate rectangular composite samples. The dimensions of the samples for X and Ku band testing were 10.16 mm (width) × 22.86 mm (length) × 2 mm (thickness) and 15.96 mm (width) × 7.9 mm (length) × 2 mm (thickness), respectively.
3 Results and discussion
The TGA and DSC curves of the as-received PCS are shown in Fig. 1. The TGA curve shows a mass loss of 26% up to 1150 °C. The mass loss is in agreement with the results previously reported by JANG et al [15] but lower than those of BOUILLON et al [16] and SHUKLA et al [17]. The distinction of mass loss may be due to the difference in molecular mass or oxygen content. The TGA curve could be separated into three regions: 1) 50-300 °C, the main mass loss resulted from thermal degradation of the PCS and evaporation of the low molecular mass components. 2) 300-750 °C, the mass loss occurred due to the cleavage of the side chains of the PCS. The conversion of the polymer into inorganic structure also occured in this region. 3) >750 °C, a mass loss of only 0.4% was attributed to the loss of residual hydrogen [15]. The corresponding DSC curve shows three broad exothermic peaks at 650, 930 and 1100 °C. The peak at 650 °C is derived from the cleavage of the side chains of the PCS. The peaks at 930 and 1100 °C may be due to the transition from amorphous phase to SiC crystalline and order process of free carbon. The evolution of XRD pattern with pyrolysis temperature is shown in Fig. 2. The products pyrolized at 800 and 900 °C show a very broad peak at 2θ=36°, corresponding to the (111) peak of β-SiC. With increasing pyrolysis temperature, the (111) gradually developed and the peaks (220) and (311) of β-SiC are visible at temperature higher than 1000 °C. The peak shapes indicate an amorphous to nanocrystalline transition of SiC with increasing temperature. In addition, the occurrence of sharp peaks at 2θ=23° and 27° indicate the presence of α-SiO2.
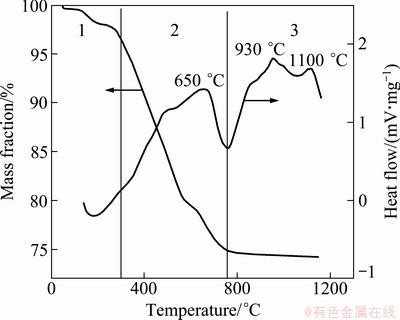
Fig. 1 TGA-DSC diagram of PCS up to 1150 °C under argon atmosphere
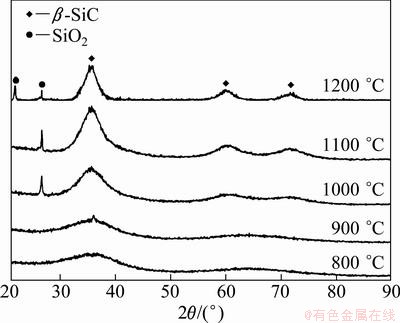
Fig. 2 XRD patterns of SiC ceramic products derived from PCS powders at 800-1200 °C
It has been recognized that carbon with graphite structure is electrically active in PDCs [8]. It is necessary to know the state of carbon that exists in the PCS-SiC. The Raman spectroscopy is an important nondestructive tool for the examination of the structural evolution of the free carbon phase in PDCs [8]. Figure 3 shows the Raman spectra of the PCS-SiC powders pyrolyzed at 800-1200 °C. The peaks of SiC cannot be observed due to its insensitivity to Raman scattering while the peaks of carbon can be identified obviously. For products pyrolized at 800 and 900 °C, asymmetric band is observed at about 1500 cm-1, suggesting that carbon still bounds to hydrogen. The splitting of this band into classical G and D bands, which begins at 1000 °C, indicates the formation of true free carbon. With rising pyrolysis temperature, the G-peak position shifted to 1584 cm-1, indicating that an ordering process occurred within the free carbon phase. It is recognized that the PCS-SiC ceramic comprises SiC and C nanocrystals embedded in amorphous phase [8,9]. Based on previous studies and our characterization, the microstructural model for PCS-SiC ceramic is proposed in Fig. 4.
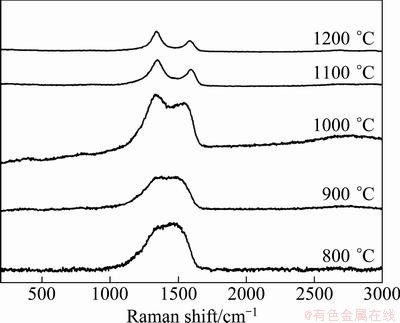
Fig. 3 Raman spectra of SiC ceramic products derived from PCS powders at 800-1200 °C
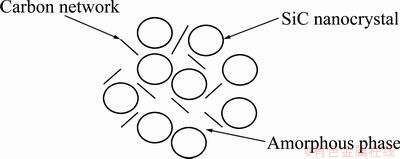
Fig. 4 Structure model of SiC ceramic product derived from PCS
Figure 5 and 6 show the ε′ and ε″ of complex permittivity of the PCS-SiC powders pyrolyzed at 800-1200 °C. It is observed that both the values of ε′ and ε″ increase with the rising of pyrolysis temperature. Before 1000 °C, the ε′ and ε″ increase slightly, but increase obviously after 1000 °C. In addition, all the spectra of ε″ exhibit a broad peak in the frequency range of 11-16 GHz. The spectra of ε′ change separately. The ε′ spectra of the powders pyrolyzed at 800-1000 °C remain nearly invariable over the range of 8.2-11.5 GHz, then slightly ascend with frequency in 11.5-14 GHz and remain nearly invariable again in 14-18 GHz; the ε′ spectra of the powders pyrolyzed at 1100 °C show slightly decreasing over 8-18 GHz; the ε′ spectra of the powders pyrolyzed at 1200 °C slightly decrease over 8-14 GHz and remain nearly invariable in 14-18 GHz. In addition, it is worth to notice that the variation trends of ε′ and ε″ with pyrolysis temperature accord with the Raman spectra. In GHz band, the relaxation polarization and reorientation polarization are the possible mechanisms for the polarization in a dielectric material, whereas dielectric loss and conductance loss contribute to the ε″ of complex permittivity. The influence of pyrolysis temperature on the complex permittivity of PCS-SiC ceramic should be related to the presence of order carbon. It was known that the PCS-SiC ceramic consists of the SiC nanocrystal and amorphous phases as insulating section and order carbon as conducting section. The insulating section acts like a high resistive path for flow of free electrons; however, some electrons may still conduct by tunneling effect [18]. In the other hand, sp2-carbon nanodomain with free electron can induce the electron relaxation polarization according to Debye theory. In summary, the rising of order carbon size with the increasing of pyrolysis temperature enhances the relaxation polarization and plays a key role in enhancing conductivity of PCS-SiC, which in turn increases the ε′ and ε″, respectively.
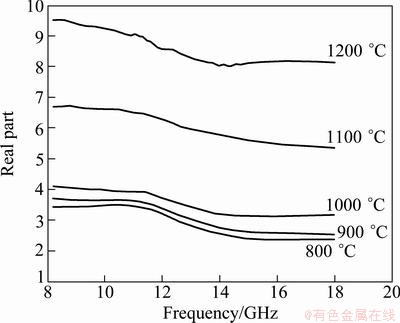
Fig. 5 Real part of complex permittivity ε′ of SiC ceramic products derived from PCS powders at 800-1200 °C
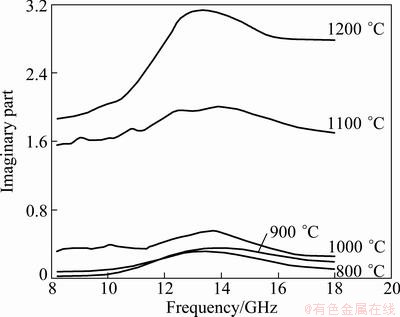
Fig. 6 Imaginary part of complex permittivity ε″ of SiC ceramic products derived from PCS powders at 800-1200 °C
4 Conclusions
The microstructure and dielectric properties in the frequency of 8.2-18 GHz of SiC ceramic derived from PCS were studied. The experimental results show that both ε′ and ε″ of complex permittivity increase with increasing the pyrolysis temperature. There are sp2-carbon dispersed PCS-SiC ceramics when the pyrolysis temperature is higher than 1000 °C. It is believed that sp2-carbon contribute to increasing of ε′ and ε″ of complex permittivity due to the electron relaxation polarization and conductance loss, respectively. The proper dielectric loss of PCS-SiC ceramics makes it as potential microwave absorbing materials.
References
[1] HUO J, WANG L, YU H J. Polymeric nanocomposites for electromagnetic wave absorption [J]. J Mater Sci, 2009, 44: 3717-3927.
[2] ZHAO D L, LUO F, ZHOU W C. Microwave absorbing property and complex permittivity of nano SiC particles doped with nitrogen [J]. J Alloys Compd, 2010, 490: 190-194.
[3] JIAO H, ZHOU W C, LUO F. Synthesis and dielectric properties of nano Si/C/N powders [J]. J Mater Chem, 2002, 12: 2459-2462.
[4] SU X L, ZHOU W C, LI Z M, LUO F, DU H L, ZHU D M. Preparation and dielectric properties of B-doped SiC powders by combustion synthesis [J]. Mater Res Bull, 2009, 42: 880-883.
[5] LI Z M, ZHOU W C, SU X L, LUO F, ZHU D L, LIU P L. Preparation and characterization of aluminum-doped silicon carbide by combustion synthesis [J]. J Am Ceram Soc, 2008, 91: 2607-2610.
[6] JIN H B, CAO M S, ZHOU W, AGATHOPOULOS S. Microwave synthesis of Al-doped SiC powders and study of the dielectric properties [J]. Mater Res Bull, 2010, 45: 247-250.
[7] LI D, JIN H B, CAO M S, CHEN T, DOU Y K, WEN B. Production of Ni-doped SiC nanopowders and their dielectric properties [J]. J Am Ceram Soc, 2011, 94: 1523-1527.
[8] COLOMOB P, MERA G, RIEDEL R, SORARU G D. Polymer-derived ceramics: 40 years of research and innovation in advanced ceramics [J]. J Am Ceram Soc, 2010, 93:1805-1837.
[9] BUNSELL AR, PIANT A. A review of the development of three generations of small diameter silicon carbide fibres [J]. J Mater Sci, 2006, 40: 823-839.
[10] LIU H T, CHENG H F, WANG J , TANG G P. Effects of the single layer CVD SiC interphases on the Mechanical Properties of the SiCf/SiC composites fabricated by PIP process [J]. Ceram Inter, 2010, 36: 2033-2037.
[11] CORDELAIR J, GREIL P. Electrical conductivity measurements as a microprobe for structure transitions in polysiloxane derived Si-O-C ceramics [J]. J Eur Ceram Soc, 2000, 20: 1947-1957.
[12] HALUSCHKA C, ENGEL C, RIEDEL R. Investigation of electrical properties of silicon carbonitride ceramics derived from polysilazanes part II [J]. J Eur Ceram Soc, 2000, 20: 1355-1364.
[13] TRASSL S, KLEEBLE H J, STRORMER H, MOTZ G, ROSSLER E, ZIEGLER G. Characterization of the free-carbon phase in Si-C-N ceramics: Part II, comparison of different polysilazane prysilazane precursors [J]. J Am Ceram Soc, 2000, 85: 1268-1274.
[14] TRASSL S, PUCHINGER M, ROSSLER E, ZIEGLER G. Electrical properties of amorphous SiCxNyHz-ceramics derived from polyvinylsilazane [J]. J Eur Ceram Soc, 2003, 23: 781-789.
[15] JANG Y S, JANK M, MAIER V, DURST K, TRAVITZKY N, ZOLLFRANK C. SiC ceramic micropatterns from polycarbosilanes [J]. J Eur Ceram Soc, 2010, 30: 2773-2779.
[16] BOUILLON E, LANGLAIS F, PAILLER R, NASLAIN R, CRUEGE F. Conversion mechanism of a polycarbosilane precursor into an SiC-based ceramic material [J]. J Mater Sci, 1991, 26: 1333-1345.
[17] SHUKLA S K, TIWARI R K, RANJAN A, SAXENA A K, MATHUR G N. Some thermal studies of polysilanes and polycarbosilanes [J]. Thermochim Acta, 2004, 424: 209-217.
[18] WENGGE Z, WONGAND S C, SUE H J. Transport behavior of PMMA/expanded graphite nanocomposites [J]. Polymer, 2002, 43: 6767-6773.
热解温度对聚碳硅烷转化SiC陶瓷结构及介电性能的影响
丁冬海1, 2,周万城1,周 璇1,罗 发1,朱冬梅1
1. 西北工业大学 凝固技术国家重点实验室,西安 710072;
2. 西安建筑科技大学 材料与矿资学院,西安 710055
摘 要:采用真空高温裂解聚碳硅烷法制备β-SiC陶瓷粉末,并对热解产物进行TGA/DSC、XRD和拉曼光谱表征。通过矩形波导法测量β-SiC陶瓷粉末与石蜡复合材料在8.2~18 GHz下的复介电常数来研究其介电性能。结果表明:复介电常数的实部与虚部均随着热解温度的升高而增大。高温下产生的石墨碳引起的电子松弛极化及电导损耗是复介电常数的实部与虚部增大的主要原因。
关键词:SiC陶瓷;聚碳硅烷转化SiC;介电性能;热解温度;自由碳;复介电常数
(Edited by YANG Hua)
Foundation item: Project (50572090) supported by the National Natural Science Foundation of China; Project (KP200901) supported by the Fund of the State Key Laboratory of Solidification Processing, China
Corresponding author: DING Dong-hai; Tel: +86-29-82207830; E-mail: 290610692@qq.com
DOI: 10.1016/S1003-6326(11)61524-0