
Microstructure and properties of high silicon aluminum alloy with 2% Fe prepared by rheo-casting
ZHONG Gu(钟 鼓), WU Shu-sen(吴树森), AN Ping(安 萍), MAO You-wu(毛有武), LI Shi-zhao(李世钊)
State Key Laboratory of Materials Processing and Die & Mould Technology,
Huazhong University of Science and Technology, Wuhan 430074, China
Received 13 May 2010; accepted 20 June 2010
Abstract: The morphology changes of both Fe-containing intermetallic compounds and the primary Si phase of Al-20Si-2Fe- 2Cu-0.4Mg-1.0Ni-0.5Mn (mass fraction, %) alloy produced by semi-solid rheo-diecasting were studied. The semi-solid slurry of high silicon aluminum alloy was prepared by direct ultrasonic vibration (DUV) which was imposed on the alloy near the liquidus temperature for about 2 min. Then, standard test samples of 6.4 mm in diameter were formed by semi-solid rheo-diecasting. The results show that the DUV treatment suppresses the formation of needle-like β-Al5(Fe,Mn)Si phase, and the Fe-containing intermetallic compounds exist in the form of fine Al4(Fe, Mn)Si2 particles. Additionally, the primary Si grows up as fine and round particles with uniform distribution in α(Al) matrix of this alloy under DUV treatment. The tensile strengths of the samples at the room temperature and 573 K are 230 MPa and 145 MPa, respectively. The coefficient of thermal expansion (CTE) between 25 °C and 300 °C is 16.052 8×10-6 °C-1, and the wear rate is 1.55%. The hardness of this alloy with 2% Fe reaches HB146.3. It is discovered that modified morphology and uniform distribution of the Fe-containing intermetallic compounds and the primary Si phase are the main reasons for reducing the CTE and increasing the wear resistance of this alloy.
Key words: high silicon aluminum alloy; ultrasonic vibration; rheo-casting; microstructure; mechanical properties
1 Introduction
The characteristics of low coefficient of thermal expansion, excellent wear resistance and corrosion resistance, as well as high specific strength of high silicon (w(Si)>18%) Al-Si alloy, attracted considerable interest in trying to prepare heat or wear-resistant parts with this alloy for automotive and aerospace applications[1-3]. Generally, alloying elements, such as Cu and Mg, were added deliberately to improve the room temperature strength substantially by solution and precipitation hardening. However, strengthening phases like Al2Cu and Al2CuMg are relatively stable below 150 °C, and Mg2Si will aggregate and coarsen over 180 °C gives rise to sharp decrease of high temperature strength[1]. Therefore, the high temperature performance needs to be further enhanced with such elements as Fe and Co, since the formation of Fe-containing intermetallic compounds are still stable at temperature of 300-400 °C[1, 4-7]. Unfortunately, conventional casting technologies produce coarse plate Fe-bearing intermetallic compounds like β-Al5FeSi, resulting in inferior mechanical properties.
In recent years, the processes of modifying the harmful Fe-bearing intermetallic compounds by rapid solidification[1, 5-7], neutralization elements addition [1, 6-7] and melt superheating were widely studied. Nevertheless, low efficiency, complicated process or high cost are accompanied with the above approaches. It has been known that ultrasonic vibration has the effects of refining microstructure of metals[8-9]. OSAWA et al[10] studied the effect of ultrasonic vibration on the morphology of intermetallic compounds of Al-xSi-4Fe (x=6, 12, 18) alloys. Recently, the morphology changing mechanism of the Fe-containing intermetallic compounds in Al-20Si-2.0Fe-2.0Cu-0.4Mg-1.0Ni alloy under direct ultrasonic vibration (DUV) treatment was conducted[11]. The results demonstrated that the formation of needle-like β phase of this kind of alloy with DUV treatment near liquidus temperature was suppressed, leading to the formation of block δ phase.
In order to utilize the advantages such as good thermal stability and excellent wear resistance of high silicon aluminum alloy with alloying element Fe, the tensile samples were made from the alloy in this study. The changes of Fe-containing intermetallic compounds and the primary Si of Al-20Si-2Fe-2Cu-0.4Mg-1.0Ni- 0.5Mn alloy produced by semi-solid rheo-diecasting were studied. The effects of the modified microstructure on the mechanical properties were also investigated.
2 Experimental
The installation of DUV for high silicon Al-Si alloy in Ref.[12] was employed in this experiment. The applied ultrasonic power was 1.8 kW, and the frequency of DUV was 20 kHz. The ultrasonic vibrator was composed of firmly connected transducer and amplitude made of titanium alloy.
The alloys used were Al-20Si-2Fe-2Cu-0.4Mg-1Ni- 0.5Mn (mass fraction, %), which were prepared by using raw materials of Al-25.8%Si and Al-10%Mn (mass fraction) master alloys, commercial pure Al (99.7%), pure Cu (99.99%), pure Mg (99.9%), pure Ni (99.99%), and pure Fe (99.9%). The final real compositions of the die casting samples analyzed with fluorescent analyzer were 20% Si, 2.25% Fe, 2.1% Cu, 0.13% Mg, 1.02% Ni, 0.534% Mn, 0.27% Ce, 0.18% La, 0.044% Zn, 0.024% Ti and balance Al (mass fraction). The liquidus and the solidus temperatures of this alloy determined by DSC are about 702 °C and 533 °C, respectively.
The alloy was melted in a resistance furnace at 820-850 °C with complex modification of 0.08% P (in the form of Cu-14% P) and 0.6% RE (in the form of Al-15% RE), in which the RE was composed of 63% Ce and 36% La. Then, the melt was held at 740-780 °C, and a metal cup was preheated simultaneously to about 690 °C. Subsequently, about 600 g liquid metal was poured into the preheated metal cup. The DUV was applied on the melt with the ultrasonic vibrator immersed into the melt 15-20 mm deep, when the liquid metal was cooled down to about 715 °C. After the melt was treated with DUV for a certain time between 715 and 690 °C, the slurry with a certain solid fraction was obtained. Then, it was poured into the chamber followed by die-casting with the injection speed of 4 m/s, and the specific injection pressure of 40 MPa. And standard test samples of 6.4 mm in diameter were obtained. For comparison, traditional liquid die-casting samples were also made without DUV at the pouring temperature of 790 °C.
Same tensile specimens were T6 heat treated with solution treatment at 510 °C for 7 h, and water quenched at 70 °C immediately, followed by aging treatment at 190 °C for 10 h. The room temperature tensile tests were carried out using a WDW3200 type test machine with crosshead rate of 0.5 mm/min. And the high temperature tensile tests were carried out using a MTS810 type high-temperature test system with crosshead rate of 1 mm/min after holding for 10 min at 573 K. The microstructure of the specimens was revealed by etching with 0.5% hydrofluoric acid solution, and was examined with an Axiovert 200MAT optical microscope. For comparison, all d6 mm×10 mm cylindrical specimens were cut from the middle of tensile tested samples for wear resistance testing by MG-2000B type high-speed and high-temperature friction and wear test machine. The load was 200 N, the speed was 200 r/min, the testing time was 10min, and the standard disc for comparison was 45# quenched steel RC (40-45). Also, the same positions from the end of specimens were machined into d8 mm×8 mm cylindrical samples. And the average Brinell hardness value of three points on the sample surface was determined by HBE-3000A electronic Brinell hardness tester.
3 Results and discussion
3.1 Microstructure of semi-solid rheo-diecast high silicon aluminum alloy
Figs.1(a) and (b) show the typical as-cast microstructures of the alloy containing 2% Fe under semi-solid rheo-diecasting with DUV treatment and liquid die-casting, respectively. As it can be seen, they are mainly composed of white α(Al), dark gray primary Si particulates, fibrous eutectic Si, and light gray fine block or needle-like Fe-containing intermetallic compounds. It can be concluded by comparing Fig.1(a) with (b) that the primary Si particles of liquid die-cast alloy are relatively coarse in heterogeneous distribution. However, besides some relatively round primary Si-1 particles (Fig.1(a)), Si-2 particles in irregular shape and many fine Si-3 particles which distribute uniformly, are in co-existence in the matrix of this rheo-cast alloy. The differences of the microstructure are illustrated by the following factors. When DUV was applied to the alloy for some time in semi-solid temperature range, the pre-formed primary Si particles tend to grew to Si-1 in mold filling and subsequent solidification process. Some stripy or polygonal Si-2 particles could be formed in the process of slurry transferring or cool chamber. While the fine Si-3 grains should be formed in the rapid solidification after filling in the mold, due to the low temperature of semi-solid slurry. Based on the early study[11], Mg2Si, AlCuNi, Al-Si-RE-Cu-Ni intermetallic compounds also present in this alloy.
The light gray compounds in Figs.1(a) and (b) are Fe-bearing phases. The one in Fig.1(a) presents small block about 8-10 μm in size of the rheo-diecast alloy with DUV treatment, while the one in Fig.1(b) is in long needle-like shape of 30-50 μm in length of the liquid die-cast alloy. According to the previous research[11], the fine block phase is δ-Al4(Fe, Mn)Si2, and the long acicular phase is β-Al5(Fe, Mn)Si. The following factors contribute to the above variations. Actually, the solidification in this mold would follow the sequence of equilibrium solidification events basically. And, most of the formed δ phases transform into β phase via the peritectic reaction L+δ-Al4FeSi2→β-Al5FeSi+Si. In the solidification process of this alloy, Fe atoms aggregate in the solidification front due to the large difference of solubility of Fe between liquid and solid Al, even a small amount of Fe in the alloy forms harmful acicular β phase. It was verified[4] that the start-freezing temperature of β phase was variable, which decreased with decreasing iron content, increasing cooling rate and increasing melt superheat temperature until it merged at the temperature of Al-Si eutectic reaction eventually. Among these factors, Fe content has the greatest impact on the start-freezing temperature of β phase, which ascends sharply with increasing the iron content. And longer growth time is obtained because of enlarged forming temperature range, causing much coarser β phase. With the application of DUV on the melt before solidification, namely near the liquidus temperature, the effect of acoustic cavitation and acoustic streaming generated by DUV is favorable to form homogenous solute distribution field and temperature field in the melt. As a result, the level of Fe solute concentration in solidification front decreases extremely[11]. So, the start-freezing temperature of β phase decreases and Fe-containing intermetallic compounds form in the form of δ phase in subsequent solidification process. About 0.5% Mn is added as neutralizing agent for Fe, and it involves in the formation of Fe-phases in the solidification process not with reactions but just a replacement of atoms due to the low Mn content, leading to coexistence of Fe and Mn atoms in the lattice of δ phase. As a result, only a small amount of Fe atoms are substituted by Mn atoms, forming the Al4(Fe,Mn)Si2 compound. Fig.2 shows the SEM image of rheo-diecast alloy containing 2% Fe with DUV treatment. And the size, morphology and distribution of δ phase are presented clearly.
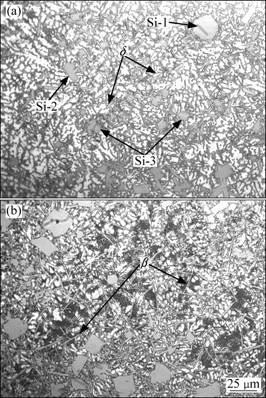
Fig.1 As-cast optical microstructures of high silicon aluminum alloy with 2% Fe: (a) Semi-solid rheo-cast; (b) liquid die-cast
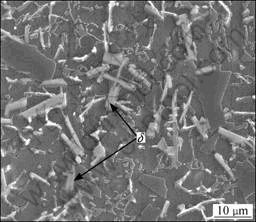
Fig.2 SEM image of rheo-diecast alloy showing δ particles
The studies on this kind of alloys with or without DUV treatment cast in gravity in metal mold were conducted previously. The results have shown that the Fe-bearing phase of the alloy without DUV was mainly 100-200 μm-long needle-like β-Al5(Fe,Mn)Si phase with staggered distribution in the matrix, while that of the alloy with DUV for 2 min presented in the form of fine Al4(Fe, Mn)Si2 particles about 20-30 μm[11]. It is shown in Fig.1(b) that the Fe-bearing phase of the die-cast alloy without DUV still presents in needle-like β phase in spite of obtaining significant refinement. It is indicated that the cooling rate produced by the die-casting refines the Fe-containing intermetallic compound apparently, but cannot transfer the acicular β phase into more favorable δ phase.
3.2 Mechanical properties of rheo-cast high silicon aluminum alloys with 2% Fe
The tensile strengths at the room and elevated temperature of both rheo-cast and liquid die-cast alloys are shown in Fig.3. Both the room and elevated temperature tensile strengths of rheo-cast alloy are much higher than those of liquid die-cast alloy. The room temperature tensile strength is 230 MPa, and the elevated temperature tensile strength at 573 K is 145 MPa, which are 38.1% and 63.4% higher than those of the liquid die-cast alloy, respectively.
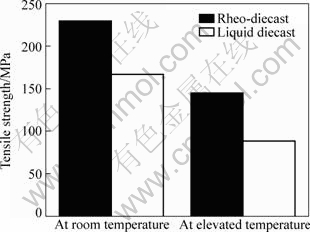
Fig.3 Tensile strengths of high silicon aluminum alloy containing 2% Fe with T6 treatment
The mechanical properties of this alloy are determined by the morphology, size and distribution of the primary Si, eutectic Si and other major intermetallic compounds. Firstly, acoustic cavitation and acoustic streaming generated by DUV are beneficial to the homogenization of solute distribution field and the temperature field of the melt, causing the primary Si to grow round in a uniform distribution. Secondly, it can be found by comparing Fig.1(a) and (b) that the Fe-containing phase of alloy with DUV is fine block δ phase rather than long needle-like β phase, which decreases the effect of cutting matrix caused by acicular β phase significantly. As a result, the tensile strengths at the room and high temperature are improved. On the other hand, the pre-formed acicular β phase exists as obstacles for the melt flow, which creates holes difficult to be fed, leading to porosity and inferior properties. Meanwhile, there are amounts of air entrapment in liquid die-casting process because of the high forming temperature and unstable flow, resulting in holes formed in the samples after T6 treatment. However, denser microstructure forms in this alloy with rheo-casting process, as shown in Fig.4. In addition, the forming temperature of semi-solid rheo-casting is low, contributing more fine primary Si particles to form which enhance the properties to some degree. Compared with the tensile strength of semi-solid rheo-cast Al-20Si alloy without Fe (σb=310 MPa, σb, 300 °C=167 MPa), the room temperature strength of the alloy with 2% Fe formed with the same process is reduced remarkably[13]. The main reason could be the existence of block δ phases that still have the effect of cutting matrix. However, the better plasticity of the alloy is obtained in high- temperature state because the lower matrix shear stress greatly weakens the stress concentration caused by the sharp corner of Fe-bearing phase. As a result, the alloys containing 2% Fe still keep excellent heat resistance with only a small decrease of high temperature strength.
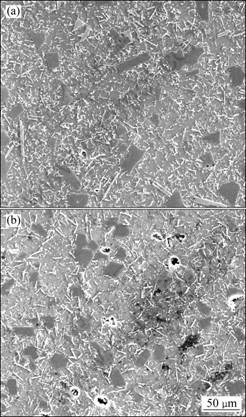
Fig.4 SEM images of alloys with T6 treatment: (a) Rheo-cast; (b) Liquid die-cast
3.3 Thermal expansion property
The coefficient of thermal expansion (CTE) at 25-300 °C of this semi-solid rheo-cast alloy is 16.052 8×10-6 °C-1. Obviously, the dimensional stability of this kind of alloy is much better than that of the traditional piston alloys A336 or A354, whose CTE value is approximately 23×10-6 °C-1. Compared with the Al-20Si alloy without Fe studied earlier (the CTE is 17.415 7×10-6 °C-1)[13], the CTE of the alloys contain- ing 2% Fe studied in this work is 7.8% smaller. The thermal expansion property of Al-Si alloys is mainly determined by the relative amount of α(Al), Si and Fe-bearing phases. And the CTE of pure Si is very small, which is (2.8-7.2)×10-6 °C-1. The Si content of the alloy in this study is 20%, much higher than that of A336 or A354 in which the silicon content is 10%-13%[14], contributing to the smaller CTE. Moreover, a large number of fine Fe-containing intermetallic compounds exist in the matrix of the rheo-cast alloy containing 2% Fe with DUV treatment. The Fe-bearing phase, which has high melting point and high hardness, is still in good thermal stability even at 400 °C. Therefore, dispersedly distributed fine block Fe-containing particles limit the expansion of α(Al) matrix at high temperatures, leading to more excellent heat resistance with lower CTE value.
3.4 Hardness and wear resistance
Table 1 summarizes the hardness and wear performance test results of samples with T6 treatment. As it can be seen from Table 1, the rheo-cast alloy with 2% Fe possesses high hardness and excellent wear resistance. The primary Si is hard particulate with micro-hardness of HV 1 000-1 300, while the aluminum is soft with micro-hardness of HV 60-100. The embedded hard particles in the soft matrix increase the hardness of the alloy, so as to improve the wear resistance greatly. Moreover, the hardness and wear resistance of the alloy are further improved by the fine δ phases which have the similar hardness with Si particles[15]. The Fe-bearing phase of liquid die-cast alloy with 2% Fe presents in long needle-like brittle β phase, and stress concentration exists in the tip of acicular Fe-bearing phase, reducing the hardness and wear resistance of the alloy.
Table 1 Wear resistance and hardness of T6 treated samples
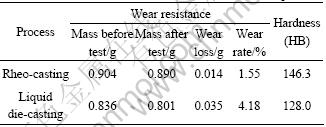
4 Conclusions
1) The primary Si particles are refined in the microstructure of rheo-diecast high silicon aluminum alloy containing 2% Fe with DUV treatment. The Fe-bearing phases of this alloy are refined effectively by rheo-casting and DUV treatment. The Fe-bearing phases of the rheo-diecast alloy with DUV treatment for 2 min present in the form of fine Al4(Fe, Mn)Si2 particles of 8-10 μm in size, while that of the liquid die-cast alloy is mainly 30-50 μm long needle-like β-Al5(Fe,Mn)Si phase.
2) The tensile strength of reho-cast alloy at room temperature and 573 K are 230 MPa and 145 MPa, respectively, which are 38.1% and 63.4% higher than those of the liquid die-cast alloy, respectively.
3) The coefficient of thermal expansion (CTE) of this alloy between 25 °C and 300 °C is 16.052 8×10-6 °C-1, and the hardness reaches HB 146.3 with good wear resistance.
References
[1] WANG F, ZHANG J S, XIONG B Q, ZHANG Y G. Effect of Fe and Mn additions on microstructure and mechanical properties of spray-deposited Al-20Si-3Cu-1Mg alloy [J]. Materials Characterization, 2009, 60(5): 384-388.
[2] CHEN C, LIU Z X, REN B, WANG M X, WENG Y G, LIU Z Y. Influences of complex modification of P and RE on microstructure and mechanical properties of hypereutectic Al-20Si alloy [J]. Transactions of Nonferrous Metals Society of China, 2007, 17: 301-306.
[3] JIANG J H, MA A B, SONG D, SAITO N, YUAN Y C, NISHIDA Y. Corrosion behavior of hypereutectic Al-23%Si alloy (AC9A) processed by severe plastic deformation [J]. Transactions of Nonferrous Metals Society of China, 2010, 20: 195-200.
[4] ANANTHA N L, SAMUEL F H, GRUZLESKI J E. Crystallization behavior of iron-containing intermetallic compounds in 319 aluminum alloy [J]. Metallurgical and Materials Transactions A, 1994, 25(8): 1761-1773.
[5] SRIVASTAVA A K, SRIVASTAVA V C, GLOTER A, OJHA S N. Microstructural features induced by spray processing and hot extrusion of an Al-18% Si-5% Fe-1.5% Cu alloy [J]. Acta Materialia, 2006, 54(7): 1741-1748.
[6] HUANG H J, CAI Y H, CUI H, HUANG J F, HE J P, ZHAN J S. Influence of Mn addition on microstructure and phase formation of spray-deposited Al-25Si-xFe-yMn alloy [J]. Materials Science and Engineering A, 2009, 502(1/2): 118-125.
[7] SEIFEDDINE S, JOHANSSON S, SVENSSON I L. The influence of cooling rate and manganese content on the β-Al5FeSi phase formation and mechanical properties of Al-Si-based alloys [J]. Materials Science and Engineering A, 2008, 490(1/2): 385-390.
[8] ESKIN G I. Ultrasonic treatment of light alloy melts [M]. Russia: Overseas Publishers Association, 1998: 1-18
[9] QIAN M, RAMIREZ A, DAS A. Ultrasonic refinement of magnesium by cavitation: Clarifying the role of wall crystals [J]. Journal of Crystal Growth, 2009, 311(14): 3708-3715.
[10] OSAWA Y, TAKAMORI S, KIMURA T. Morphology of intermetallic Compounds in Al-Si-Fe alloy and its control by ultrasonic vibration [J]. Materials Transactions, 2007, 48(9): 2467-2475.
[11] ZHONG G, WU S S, JIANG H W, AN P. Effects of ultrasonic vibration on the iron-containing intermetallic compounds of high silicon aluminum alloy with 2% Fe [J]. Journal of Alloys and Compounds, 2010, 492: 482-487.
[12] ZHAO J W, WU S S, WAN L, CHEN Q H, AN P. Evolution of microstructure of semi-solid metal slurry in ultrasound field [J]. Acta Metallurgical Sinica, 2009, 45(3): 314-319. (in Chinese)
[13] WU S S, ZHONG G, WAN L, AN P, MAO Y W. Microstructure and properties of rheo-diecast AlSi20Cu2Ni1Mg0.4 alloy with direct ultrasonic vibration process[C]//The 11th International Conference on Semi-Solid Processing of Alloys and Composites. Beijing, 2010.
[14] JOHN E H. Aluminum properties and physical metallurgy [M]. Metals Park, Ohio: American Society for Metals, 1984.
[15] CHEN C L, RICHTER A, THOMSON R C. Mechanical properties of intermetallic phases in multi-component Al-Si alloys using nanoindentation [J]. Intermetallics, 2009, 17: 634-641.
(Edited by YANG Bing)
Foundation item: Project(2007AA03Z557) supported by the National High-tech Research and Development Program of China; Project(50775086) supported by the National Natural Science Foundation of China
Corresponding author: WU Shu-sen; Tel: +86-27-87556262; E-mail: ssw636@hust.edu.cn
DOI: 10.1016/S1003-6326(09)60346-0