
Structure and properties of SEBS/PP/OMMT nanocomposites
WANG Xia1, PANG Shao-long1,2, YANG Jun-he1 , YANG Fan2
1. Department of Materials Science and Engineering, Shanghai Institute of Technology, Shanghai 200235, China;
2. Chemistry Department, East China Normal University, Shanghai 200062, China
Received 10 April 2006; accepted 25 April 2006
Abstract: Nanocomposites based on styrene-b-(ethylene-co–butylenes)-b-styrene triblock copolymer(SEBS), polypropylene (PP) and organomontmorillonite (OMMT) were prepared using melt intercalation by a twin mill. The nanostructure of SEBS/PP/OMMT hybrids was characterized by X-ray diffractometry(XRD) and transmission electron microscopy(TEM). The d001 space of OMMT and SEBS/PP/OMMT hybrid increases from 1.30 nm to 3.52 nm and 4.06 nm, respectively which indicate, that the C16 and SEBS/PP could intercalate in the silicate layers and expand the basal spacing. The formation of intercalated structure was also confirmed by TEM. The results show that the individual silicates are well dispersed in the elastomer matrix. The glass transition temperature (tg) of PS block of SEBS was investigated by dynamic mechanical analysis (DMA). It is shown that there are remarkable enhancements in tg of PS block containing a small mass fraction of OMMT. tg of PS in SEBS of the nanocomposites has been increased about 39 ℃ when containing 4% OMMT. The influence of OMMT on the dynamic storage moduli is temperature dependent. Below 50 ℃ the SEBS/PP/OMMT composites with higher OMMT contents show higher dynamic storage moduli. OMMT has the function of reinforcing SEBS/PP elastomers. However, the influence of OMMT on the mechanical properties of the nanocomposites is not significant according to the tensile test and tear test.
Key words: SEBS; polypropylene; organomontmorillonite; nanocomposites
1 Introduction
Polymeric nanocomposites are a new class of materials with at least one ultrafine phase dimension, typically a few nanometers. Polymer nanocomposites have received considerable attentions because of the intriguing properties and unique structures[1]. The PA6/clay nanocomposite was firstly synthesized by a Toyota research group[1,2]. Individual layers (about 1 nm thickness) of clay particles were exfoliated in a polymer matrix (nylon-6). The nanostructure was characterized by X-ray diffractometry(XRD) and transmission electron microscopy(TEM)[3,4]. Polymer/ clay nanocomposites can improve dramatically the mechanical reinforcement and high temperature durability [1-7], provide enhanced barrier properties[8, 9] and reduce flammability[5]. The optimal performance of such composites is achieved when the clay fillers are uniformly dispersed in the polymer matrix. The major difficulty of fabricating such homogeneous mixture arises from the inherent structure of the clay particles, which are composed of broad, closely stacked silicate sheets. The diameter of the stacked sheets lies typically between 20 and 200 nm, depending on the specific type of clay, and each sheet is roughly 1 nm in thickness. The spacing (or gallery) between the sheets is also on the order of 1 nm, which is smaller than the radius of gyration of typical polymers. Consequently, there is a large entropic barrier that inhibits the polymer from penetrating the gap and intermixing with the clay. Dispersion of the silicate layers in the polymer or precursor often requires replacement of the exchange cations of the native clay by organophilic cations to compatibilize the interlayer galleries with polymer matrix[1,10].
Thermoplastic elastomers form a new class of polymeric materials that have large number of applications because of their unique combination of both mechanical properties and processability. Their modulus level is comparable to that of the reinforced rubber vulacnizate under the using conditions, which covers the range from the low temperature near the glass transition temperature of the rubbery component to the higher temperature approaching the melting or softening point of the plastic component. At the processsing temperature, thermoplastic elastomers are in the melt state such that they can be processed with plastic processing equipment. Among the thermoplastic elastomers, the triblock copolymer is a special kind.Typical examples are styrene-b-butylenes-b-styrene (SBS), styrene-b-isoprene-b-styrene(SIS), and styrene (SEBS) triblock copolymers. SEBS is the hardest and most resistant to degradation amongst the three[10].The major applications of SEBS are in adhesives, sealants, coatings, footwear industry, automotive parts, and wire insulation, etc. Blending with PP, one of the most common plastic, the mechanical and processing properties of the SEBS/PP blend have been greatly improved[12-14].
In this paper, nanocomposites based on SEBS, PP and hexadecyltrimethylammomum bromide (C16) exchanged montmorillonite[15] (MMT) were prepared using melt mixing. The results of the intercalated structure, thermal and mechanical properties of SEBS/PP/ OMMT nanocomposites were reported. Structure, thermal and mechanical properties of SEBS/PP/OMMT nanocomposites were reported and discussed.
2 Experimental
2.1 Materials
SEBS (3151) was purchased as powders from TSRC Corporation. PP (S700) was supplied as pellets by Sinopec Yangzi Petrochemical Co. Ltd. The pristine montmorillonite was kindly provided by Shanghai. Weisheng Company, which contain exchangeable cations of primarily Na+. Hexadecyltrimethyl-ammomum bromide (C16) was provided by Shanghai Lingfeng Chemical Reagent Co. Ltd. The processing oil was supplied by Shanghai Daliang Chemical Co.Ltd.. Antioxidant1010 was kindly provided by Shanghai Fromtier Elastomer Co. Ltd.
2.2 Preparation of organomontmorillonite
Organophilic montmorillonite was prepared by cation exchange of natural counterions with ammonium surfactants according to the method of KAWASUMI et al [16]. The ammonium salts used was hexadecyl trimethyl ammomum bromide (C16). In this paper, OMMT was prepared as follows: The C16 was initially solubilized in distilled water at 50 ℃, then the wetted MMT was added into a blender, and the blend was milled at a speed of 30 r/min for 5 h. The organophilic clay was dried under the vacuum at 80 ℃ for several hours before further use. The interlayer spacing for MMT and C16 functionalized MMT was characterized by X-ray diffraction.
2.3 Preparation of SEBS/PP/OMMT nanocomposites
SEBS/PP/OMMT nanocomposites were prepared by melt-mixing in twin mill (TE-35, JiangShu, China). The processing oil, 100 parts per 100 parts of SEBS (3151), was first soaked up in the SEBS.The oil-soaked SEBS powders were dry-mixed with polypylene, OMMT and antioxidant (1010). The dry-mixtures were fed in acounter-ratating, intermeshing twin-screw extruder.The mixing temperature was maintained at 220 ℃; the rotor speed was 150 r/min.
2.4 Characterization of nanocomposites
The intercalated structure was characterized by X-ray diffractometry (XRD) and transmission electron microscopy (TEM). The XRD experiments were performed on a Japan Rigaku D/max 2550 VB/PC X-ray diffractometer with Cu (l=0.154 06 nm) irradiation at a scan rate of 4(?)/min. Bragg’s law, λ=2dsinθ, was used to calculate the d001 space of MMT and OMMT in the blends.
Transmission electron microscope (TEM) images of nanocomposites were obtained by a Hitachi-800 transmission electron microscope at an acceleration voltage of 175 kV. The nanocomposite samples for TEM observation were ultrathin-sectioned using an ultramicrotome (Reichert-Jung) equipped with a diamond knife at -100 ℃.
Dynamic mechanical properties were measured using a dynamic mechanical analyzer (Netzsch DMA 242C) with a dual cantilever bending mode. The DMA specimens (32 mm×6 mm×2 mm) were cut from the center of the samples. A temperature sweep at 3 ℃/min from -80 ℃ to 200 ℃ at a vibration frequency of 1 Hz and 2 Hz was used to determine the dynamic storage modulus G′ and the tan δ. The ratio of the loss modulus (E″) to the storage modulus (E′) is known as a mechanical loss factor (tan δ).
Tensile properties and Tear properties tests were performed using a universal tester (Sun 500, Italy Galdablni) according to GB/T 528s-1998 and GB/T 529-1999, respectively. The tensile tests and tear strength tests were conducted at a crosshead speed of 500 mm/min.
3 Results and discussion
The surface of the pristine montmorillonite is hydrophilic. The small inroganic cations such as Na+, Ca+ can be exchanged by organic cations[17]. After cation exchange by a surfactant, the clay surface becomes hydrophobic. The resulting organomontmorill- onite is dispersed easily in organic media. Fig.1 shows the XRD patterns of pristine MMT, OMMT and SEBS/PP/OMMT hybrids. The silicate layer (001) reflection has peaks at 2θ=6.80?, 2θ=2.51? and 2θ =2.17? for MMT, OMMT and SEBS/PP/OMMT, respectively (Fig.1). The d001 spacing was calculated from Bragg’s law: d001=l/(2sinθ), where l is the X-ray wavelength (0.154 06 nm). After intercalated into the galleries, the d001 space of OMMT and SEBS/PP/OMMT hybrid increased from 1.30 to 3.52 and 4.07 nm, respectively (Table 1). The results indicate that the C16 and SEBS/PP
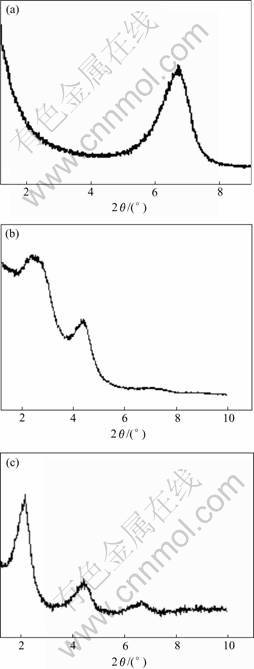
Fig.1 XRD patterns of MMT(a), OMMT(b) and SEBS/PP/OMMT(c)
Table 1 d001 space of samples
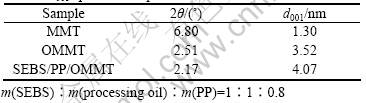
could intercalate in the silicate layers and expand the basal spacing.
The intercalated structure was also confirmed by TEM as shown in Fig.2. SEBS/PP/MMT materials are conventional composites that contain clay tactoids with the layers aggregated in an un-intercalated form (Fig.2(a)). The image comfirmed that SEBS/PP/OMMT nanocomposites are formed, as the individual silicates are well-dispersed (exfoliated) in the polymer matrix (Fig.2(b)).
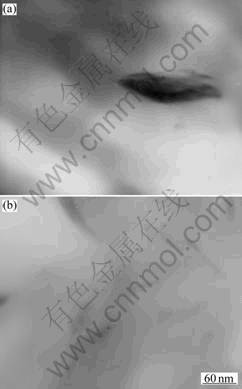
Fig.2 TEM images of SEBS/PP/MMT-3%(a) and SEBS/PP/ OMMT-3%(b)
The glass transition temperatures of the PS block domains in SEBS were difficult to observe in DSC measurements on the SEBS/PP/OMMT nanocomposites. They were taken instead from the temperature of the tanδ peakobtained by DMA, as illustrated the results obtained with the nanocomposites (Fig.3). Results for the different SEBS/PP/OMMT nanocomposites at different clay contents are summarized in Table 2. There are remarkable enhancements in tg of PS ascribed to exfoliated silicate layers. block containing a small weight fraction of OMMT. The tg of the nanocomposites containing 4% OMMT is about 39 ℃ higher than that of SEBS/PP, which can be ascribed to exfoliated silicate layers.
Table 2 Glass transition temperature of PS block of SEBS at different mass fraction of OMMT
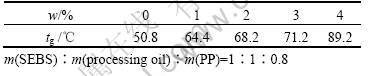
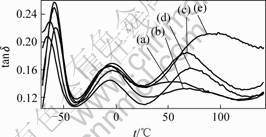
Fig.3 DMA scanning patterns of SEBS/PP/OMMT nano- composite: (a) No OMMT; (b) 1% OMMT; (c) 2% OMMT; (d) 3% OMMT; (e) 4 % OMMT
Dynamic storage moduli of the SEBS/PP/OMMT nanocomposites were measured by DMA from -40-140 ℃. The influence of OMMT on the dynamic storage moduli is temperature dependent. As indicated by Fig.4 and Table 3, below 50 ℃ OMMT can improve the dynamic storage moduli. The SEBS/PP/OMMT nanocomposites with higher OMMT contents shows higher the dynamic storage moduli. OMMT has the function of reinforcing SEBS/PP elastomers. Whereas dynamic storage moduli of the SEBS/PP/OMMT nanocomposites are decreased by increasing the contents of OMMT above 50 ℃. The possible reason is that the mobility of molecular chain has been increased with the rising of the temperature. This led to the weakeness of intermolecular interaction. The addition of OMMT further decreases the intermolecular interaction. The addition of OMMT causes the decrease of dynamic storage as compared with non-OMMT modified SEBS/PP.
Effect of OMMT content on mechanical properties
Table 3 Dynamic storage moduli of SEBS/PP/OMMT nano- composites(MPa)
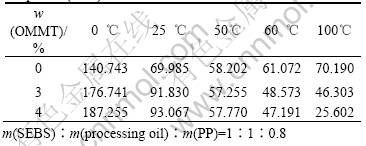
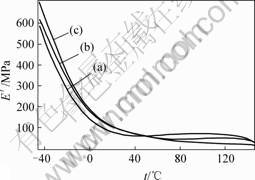
Fig. 4 DMA scanning patterns of SEBS/PP/OMMT nanoco-mposite: (a) No OMMT; (b) 3 % OMMT; (c) 4% OMMT
Table 4 Mechanical properties of SEBS/PP /OMMT nano- composites
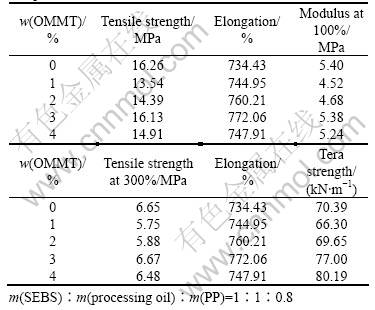
of SEBS/PP/OMMT nanocomposites was studied and the results are shown in Table 3. However, the influence of OMMT on the mechanical properties of the nanocomposites was not significant. The results are different from what have been reported[1-3]. And the reasons are under investigation.
4 Conclusions
SEBS/PP/OMMT nanocomposites have been prepared by melt intercalation with hexadecyltrimethyl- ammomum bromide (C16) exchanged montmorillonite. The nanocomposite strcture is demonstrated by XRD and TEM. The tg of the PS blocks of SEBS and the mechanical properites of the nanocomposites were characterized by DMA and an universal tester. There are significant enhancements in the glass transition temperature (tg) for PS block of SEBS containing a small mass fraction of OMMT. The influence of OMMT on the dynamic storage moduli is temperature dependent. However, the influence of OMMT on the mechanical properties of the nanocomposites was not pronounced.
References
[1] USUKI A, KOJIMA Y, KAWASUMI M, OKADA A, FUKUSHIMA Y, KURAUCHI T, KAMIGAITO O. Mechanical properties of nylon 6-clay hybrid [J]. J Mater Res, 1993, 8: 1185-1189.
[2] USUKI A, KOJIMA Y, KAWASUMI M, OKADA A,FUKUSHIMA Y, KURAUCHI T, KAMIGAITO O. Synthesis of nylon 6-clay hybid[J]. J Mater Res, 1993, 8: 1179-1184.
[3] ZHENG X J, LI J Y, ZHOU Y C. X-ray diffraction measurement of residual stress in PZT thin films prepared by pulsed laser deposition [J]. Acta Mater, 2004,52(11): 3313-3322.
[4] ZHENG X J, YANG Z Y, ZHOU Y C. Residual stresses in Pb(Zr0.52Ti0.48)O3 thin films deposited by metal organic decomposition[J]. Scripta Mater, 2003, 49: 71-76.
[5] GIANNELLIS E P. Polymer layered silicate nanocomposites[J]. Adv Mater, 1996, 8: 29-35.
[6] LAN T, PINNAVAIA T J. Clay-reinforced epoxy nanocomposites [J]. Chem Mater, 1994, 6: 2216-2219.
[7] KELLY P, AKELAH A, QUTUBUDDIN S, MIET A. Reduction of residual stress in montmorillonite/epoxy compounds[J]. J Mater Sci, 1994, 29: 2274-2280.
[8] MESSERSMITH P B, GIANNELIS E P. Synthesis and characterization of layered silicate-epoxy nanocomposites[J]. Chem Mater, 1994, 6: 1719-1725.
[9] KOJIMA Y, FUJUSHIMA A,USUKI A, OKADA A, KURAUCHI T. Gas permeabilities in rubber-clay hybrids[J]. J Mater Sci Lett, 1993, 12: 889-890.
[10] STRAWHECKER K, MANIAS E. Surcture and proerties of Poly (vinyl alcohol ) Na+ montmorillonite nanocomposites[J]. Chem Mater, 2000, 12: 2943-2949.
[11] ALLEN N S, EDGE M, WILKINSON A, LIAUW C M, MOURELATOU D, BARRIO J, MART?NEZ-ZAPORTA M A. Degradation and stabilization of styrene–ethylene–butadiene–styrene (SEBS) block copolymer[J]. Polymer Degradation and Stability, 2000, 71: 113-122.
[12] OHLSSON B, HASSANDER H, T?RNELL B. Blends and thermoplastic interpenetrating polymer networks of polypropylene and polystyrene-block-poly (ethylene-stat-butylene)-block- polystyrene triblock copolymer(1) morphology and structure-related properties[J]. Ploym Eng Sci, 1996, 36(4): 501-510.
[13] OHLSSON B, T?RNELL B. Melt miscibility in blends of polypropylene, polystyrene-block-poly (ethylene-stat-butylene) -block-polystyrene, and processing oil from melting point depression [J]. Polym Eng Sci, 1996, 36(11): 1547-1556.
[14] CHEN H L, ZHOU L Y, YE Q, CHEN J. Study on the properties of SEBS blends[J]. Modern Plastics Processing and Applications, 2004, 16 (4): 42-44.(in Chinese)
[15] MACKENZIE R C. The Differential Thermal Investigation of Clays [M]. London: Mineralogical Society, 1957. 56.
[16] KAWASUMI M, HASEGAWA N, KATO M, USUKI A, OKADA A. Preparation and mechanical properties of polypropylene-clay hybrids [J]. Macromolecules, 1997, 30: 6333-6338.
[17] THENG B K G. The Chemistry of Clay-organic Reactions[M]. London: AdamHilger, 1974.
(Edited by LONG Huai-zhong)
Foundation item: Project(040A02) supported by Key Science Foundation of Shanghai Higher Education; Project(P1502) supported by Shanghai Leading Academic Discipline.
Corresponding author: WANG Xia; Tel: +86-21-64942815; Fax: +86-21-64942815; E-mail: wangxia@sit.edu.cn