Article ID: 1003-6326(2005)05-1077-04
Impact toughness of tungsten films deposited on martensite stainless steel
HUANG Ning-kang(黄宁康), YANG Bin(杨 斌), WANG De-zhi(汪德志)
(Key Laboratory of Radiation Physics and Technology, Ministry of Education,
Institute of Nuclear Science and Technology, Sichuan University, Chengdu 610064, China)
Abstract: Tungsten films were deposited on stainless steel Charpy specimens by magnetron sputtering followed by electron beam heat treatment. Charpy impact tests and scanning electron microscopy were used to investigate the ductile-brittle transition behavior of the specimens. With decreasing test temperature the fracture mode was transformed from ductile to brittle for both kinds of specimens with and without W films. The data of the crack initiation energy, crack propagation energy, impact absorbing energy, fracture time and deflection as well as the fracture morphologies at test temperature of -70℃ show that W films can improve the impact toughness of stainless steel.
Key words: W film; stainless steel; Charpy impact test CLC number: TH140.7; O484.5; TF125.2
Document code: A
1 INTRODUCTION
Low-Z materials like carbide are being considered a plasma facing material for fusion reactors[1, 2]. Apart from those above, high-Z tungsten has a higher energy threshold for physical sputtering, it does not form hydrides, and it has the highest melting point among all metals, the lowest vapor pressure, good thermal conductivity and high temperature strength. These features make it useful as a coating for the plasma-facing wall in a fusion reactor. Nevertheless there remain several problems to be solved before its application as a plasma facing material, for example, its brittle nature, difficult machining, erosion and plasma contamination, significant re-crystallization with grain growth at a high temperature operation, and uncertainty in tritium retention and hydrogen effects[3-5].
High-Z refractory metals are usually produced by powder metallurgy. W is very brittle below a ductile-brittle transition temperature(DBTT) which is usually near or slightly above room temperature(RT), hence, machining is very difficult at RT. Due to the lack of ductility the utilization of tungsten as a structural material does not seem easy nor reliable[6]. Improvement of the brittle nature of W by alloying is in progress. An alternative is to deposit W as thin layer on some structure materials.
Based on the above, W coatings on the fusion reactor wall materials are being developed. There are many methods for W film preparation, such as chemical vapor deposition, plasma spray, deposition with ion, electron, and laser beams[ 6, 7]. However, the thermal expansion coefficient of W is so small that matching of the thermal expansion coefficient of W and substrate materials is difficult. Consequently, efforts have been made to find some suitable coating methods and their technologies to avoid this phenomenon. It has been found that W films with columnar grain structure have good resistance to cracking on stainless steel, and it has also been found that the improvement in the degree of mismatch in thermal coefficients between W and substrate materials can be achieved by using a suitable transition layer between the film and the substrate. In this paper, we report the ductile-brittle transition behavior of stainless steel specimen, on which W film is deposited, in order to study the effect of W film on the impact toughness of stainless steel.
2 EXPERIMENTAL
Standard Charpy V notched substrates were made of martensitic type stainless steel for instrumented impact test. The composition of the stainless steel was: Cr 12%-14%, C 0.08%-0.15%, and Fe balance, the substrates were heat-treated with oil quenching at 1000-1050℃ followed tempering at 700-790℃, and then polished, degreased in benzene using ultrasonic cleaning, rinsed in de-ionized water and finally dried. W films on substrates were prepared by DC magnetron sputtering, followed by 30keV Ar+ ion bombardment in the multifunction deposition system[8]. The chamber had a base pressure of 6×10-4Pa but the pressure increased to 0.1Pa during DC magnetron sputtering deposition due to argon feeding the discharge. The film of about 12nm thickness was deposited by DC magnetron sputtering, then was bombarded by 30keV Ar+ ion beam. The density of the deposited W films is usually smaller than that of bulk tungsten based on the TRIM-92 code calculation. Deposition was continued to get the second layer with the same thickness on the surface of first layer, and followed by Ar+ bombardment with the same condition as the former. Such a process was repeated until the thickness of W films was about 200nm. Each process of Ar+ ion beam bombardment was with a dose of (0.6-1)×1016 ion/cm2. After that, electron beam with a power density of about 5×103W/cm2 was used for heating these samples, and this step was repeated until the W films were about 3μm thick. The vacuum during the Ar+ ion bombardment or electron beam heating was about (5-6)×10-3Pa.
Instrumented Charpy impact tests were performed on a Tinius Olson machine equipped with an automatic specimen positioning and a data collection system. The striker was instrumented with strain gauges and an optical sensor was installed on the pendulum mass for displacement measurement. The transfer time from the furnace to impact was measured equal to 1.85s. Then series of tests with instrumented specimens were performed at losses after 1.85s. Usually, specimens were brought to a bath and maintained for half an hour before testing. The bath tem-perature was controlled, where low temperature was obtained by liquid-nitrogen. The temperature uncertainty was ±1℃. The micrographs of the fracture surfaces of specimens were obtained with an AMRAY 1845 type scanning electron microscope.
3 RESULTS AND DISCUSSION
Fig.1 shows a schematic curve of impacting load(L) vs displacement (Δ). Here displacement means change of specimen in size during impact process. Ei, shown in Fig.1, is the crack initiation energy which includes elastic crack initiation energy and plastic crack initiation energy. After that, crack starts growth. Ep, shown in Fig.1, represents the crack propagation energy, which includes stable crack growth energy and un-stable crack growth energy.
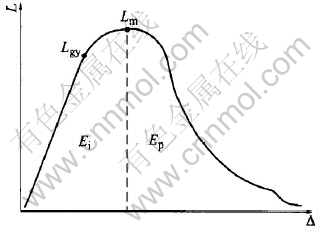
Fig.1 Schematic curve of impacting load vs displacement
Fig.2 shows the curves of crack initiation energy Ei at different test temperatures. It can be seen that the curves for the two kinds of samples are similar, but the energy is greater for the sample with W films. Both curves show that Ei is the lowest at about -120℃, then increases with temperature to a peak value between -60℃ and -50℃. Usually, the magnitude of Ei indicates the difficulty of crack initiation. This suggests W film on the surface of stainless steel might effectively impede crack initiation.
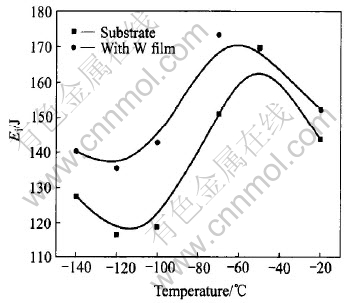
Fig.2 Curves of crack initiation energy Ei vs test temperature for stainless steel substrate
with and without W film
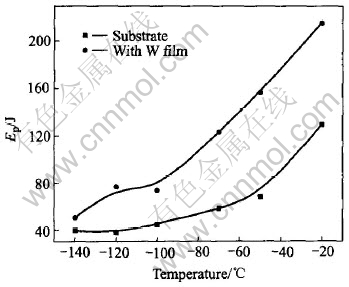
Fig.3 Curves of crack propagation energy Ep vs test temperature for stainless steel substrate
with and without W film
Fig.3 shows the curves of crack propagation energy Ep at different test temperature. The Ep vs temperature trends are similar for the two specimen types, but, Ep is always larger for the specimens with W films. This suggests that crack propagation is also more difficult for stainless steel with deposited W film.
An impact absorbing energy Et consists of crack initiation energy Ei and crack propagation energy Ep, i.e. Et=Ei+Ep. The Et value is always larger for the samples with W films, suggesting that deposition of W film on stainless steel can effectively improve the impact toughness of stainless steel.
Fig.4 shows the deflection Dx curves at different test temperatures. Deflection Dx is defined as the total displacement of the specimen under load at some temperature by a Charpy impact test. Usually, the larger the deflection, the better the impact toughness. Again there is not much difference in Dx at -140--22℃, but Dx becomes larger for the specimen with W film at -70--20℃. This suggests that the toughness of specimen with W film is better than that of stainless steel substrates.
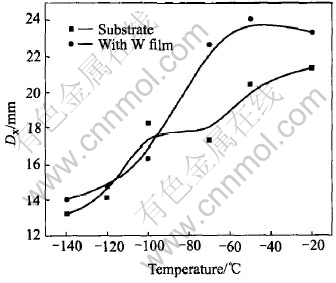
Fig.4 Deflection Dx vs test temperature for stainless steel substrate with and
without W film
The ductile-brittle transition temperature T0 was determined by fitting the following equation to each data set:
E=A+Btanh[(T-T0)/C]
where (A+B) is the upper shelf energy, (A-B) is the lower shelf energy, B/C is the slope of E(T), T is temperature, T0 is the ductile-brittle transition temperature (DBTT), and tanh[(T-T0)/C] is the hyperbolic tangent function. A schematic curve of the hyperbolic tangent adjustment is shown in Fig.5. Table 1 gives the corresponding fit parameters for each data set. The result shows that the ductile-brittle transition temperature T0 is -74℃ for the specimen with W film, while -66℃ for the stainless steel.
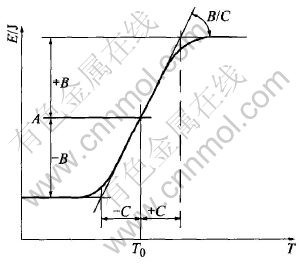
Fig.5 Schematic curve of hyperbolic tangent adjustment
Fig.6 shows the fracture surfaces at -70℃ for stainless steel substrates with and without W films by SEM. The fracture surfaces indicate characteristic dimple fracture in a net-like distribution. The difference between the both specimens is that the dimples for the specimens with W film are larger and deeper than those for substrate. The larger and deeper the dimples, the better the toughness of specimens. This observation confirms that W film on stainless steel can improve impact toughness of stainless steel.
Table 1 Characteristic parameters of hyperbolic tangent adjustment

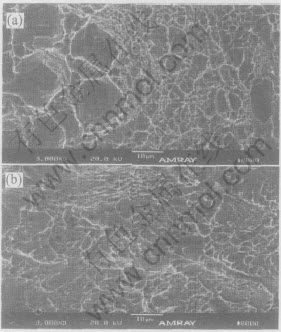
Fig.6 Fracture morphologies of stainless steel substrate with(a) and without(b) W film at -70℃
4 CONCLUSIONS
1) The variations with temperature of crack initiation energy Ei and propagation energy Ep, impact absorbing energy Et, fracture time and deflection Dx are similar for both types of specimens, but the values are systematically greater for specimens with W films, suggesting the films improve impact toughness.
2) Estimation of DBTT show that T0 is about-74℃ for the specimen with W film, while about -66℃ for the stainless steel substrate.
3) Larger and deeper dimples can be found in the specimens with W films compared with those in substrate. This further confirms that W films can effectively improve the impact toughness of stainless steel.
REFERENCES
[1]HUANG N K, XIONG Q, WANG D Z. Study in chemical bonding states of SiC films before and after hydrogen ion irradiation [J]. J Nucl Mater, 2003, 321: 152-157.
[2]HUANG N K, YANG B, XIONG Q. Behaviors of hydrogen in C-SiC films with IR and SIMS analyses [J]. Nucl Instr & Meth, 2002, B195: 344-349.
[3]Reuther T C. A perspective on the history, status and future of fusion materials research in the United States [J]. J Nucl Mater, 1991, 179-181: 9-12.
[4]Mori S. Prospects and strategy for the development of fusion reactors [J]. J Nucl Mater, 1992, 191-194: 3-6.
[5]Jung P. Hydrogen inventory and embrittlement in low activation steels [J]. J Nucl Mater, 1998, 258-263: 124-129.
[6]WANG D Z, CHEN J X, ZHANG H L, et al. Influence of ionic species on phase formation in ion mixing of carbon film on tungsten [J]. Nucl Instr & Meth, 2000, B171: 465-469.
[7]Mattas R F, Smith D L, Wu C H, et al. Materials issues in the design of the ITER first wall, blanket, and divertor [J]. J Nucl Mater, 1992, 191-194: 139-145.
[8]HUANG N K, WANG M H, SHEN Y. Multifunction equipment combining ion, electron and atom beams [J]. Meas Sci Tech, 1993, 3: 879-883.
Foundation item: Project(59781002) supported by the National Natural Science Foundation of China; Project(98061001) supported by Specialized Research Fund for the Doctoral Program of Higher Education
Received date: 2004-11-05; Accepted date: 2005-04-20
Correspondence: HUANG Ning-kang, Professor; Tel: +86-28-85412230; Fax: +86-28-85410252; E-mail: huang_072@163.com
(Edited by YUAN Sai-qian)