
Extrusion behavior of micro-nanostructured Al-18%Si alloy powders
LIANG Hong-yu(梁红玉)1, 2, OUYANG Zhi-ying(欧阳志英)1, MAO Xie-min(毛协民)1, HU Zhi-heng(胡志恒)1
1. School of Materials Science and Engineering, Shanghai University, Shanghai 200072, China;
2. Department of Mechanical and Electronic Engineering, Zhongbei University, Taiyuan 030008, China
Received 28 July 2006; accepted 15 September 2006
Abstract: The extrusion of Al-Si alloy powders with different particle sizes allows manufacture of different products with unique microstructures and therefore with unique mechanical properties. The effects of powder size on the extrusion behavior and process defect of Al-18%Si alloy were studied by means of microscopy (optical, scanning electron) and density determination. The main objective of the work is to demonstrate the influence of the powder material characteristics on final density and quality of bar. The results show that the bigger the powder particles, the better the performance of cold compacting. The surface of alloy bar extruded from big particles has good quality without cracking. While the smaller the powder particles, the higher the density and the better the microstructure and mechanical properties. For practice application, the mixed powders are better than single powder.
Key words: rapid solidification; gas atomization; micro-nanostructure; extrusion; cold compacting; mixed powders
1 Introduction
Micro-nanostructured high silicium aluminium alloys have promising mechanical and wear resistant properties. In particular, ultimate tensile strength exceeding 500 MPa was reported at ambient temperature and even exceeding 400 MPa was achieved at 150 ℃[1-2]. Unfortunately, currently available micronano- structured Al-Si alloys can be produced only in ribbon, flake or powder forms, due to a high critical cooling rate necessary to achieve micro-nanophases [3]. Therefore, consolidation of powders or flakes is needed to make usable bulk micro-nanostructured Al-Si alloys materials.
In spite of difficulties in mass production, the melt spinning process is most popular in preparing specimens for scientific research due to its easy process control and suitable specimen geometry for analysis. However, mass production is essential for the practical application of the rapidly solidified micro-nanostructured Al-Si alloys to the engineering area. From this point of view, a gas atomization process seems to be a promising route because it is suitable for mass production and appropriate consolidation techniques have been developed recently [4-7].
Most of the studies on micro-nanostructured Al-Si alloys were focused on crystallization behavior, microstructure analysis and mechanical properties of melt spun or heat treated ribbons[8-9]. However, there was seldom reports on the extrusion behavior of powder particles during consolidation.
In the present Al-18%Si alloy powders, a broad range of microstructures, varying within the powder particles, has been noted. The extrusion of Al-Si alloy powders with different sizes allows manufacture of different products with unique microstructures and mechanical properties. It is, therefore, of interest to examine the behavior of the powder particles during extrusion as a function of the powder size. The main objective of this work is to demonstrate the influence of the powder characteristics on final density and quality of bar.
2 Experimental
A master alloy of Al-18%Si was prepared by medium-frequency vacuum induction melting of commercially pure metals in graphite crucibles. Alloy powders were manufactured by remelting the master alloy in a graphite crucible at 60 K above the liquidus temperature, and bottom pouring through a ceramic melt delivery nozzle of 6.4 mm in diameter into a confined air atomizer operated at a pressure of 0.3 MPa. The gas flow rate was about 34 m3/h, the angle of gas nozzle was 25?, and the slot width of the gas nozzle was 0.55 mm. The schematic diagram of gas-atomizer arrangement is shown in Fig.1.
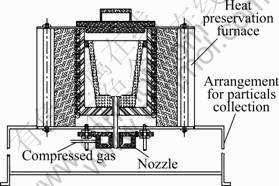
Fig.1 Schematic diagram of gas-atomizer arrangement
The size distribution of the alloy powders was measured by a conventional mechanical sieving technique. In order to examine the effects of powder size on the solidification microstructure, the powders were divided into six groups depending on their sizes: group A, powders smaller than 43 μm; group B, powders between 43 and 61 μm; group C, powders between 61 and 74 μm; group D, powders between 74 and 104 μm; group E, powders between 104 and 147 μm; and group F, powders bigger than 147 μm. Each group of alloy powders was consolidated by cold compacting and finally hot extruding in an Al can with an area reduction ratio of 4?1 and 19?1 and die angle of 90? at 450 ℃ into bars of 8 mm and 16 mm in diameter. Ram speed was kept at 5 mm/s. Die was preheated at 250 ℃.
Some extrusion operations were stopped to examine the behavior of the original powder particles in the course of the deformation with optical microscopy.
3 Results and discussion
Fig.2 shows the scanning electron micrograph taken from the mixed powders, revealing typical morphology of powders with different sizes. The shape of the smaller powders is mainly spherical and has a smooth surface. With increasing powder size, the powder particles become elliptical in shape, the number of satellites on the powder surface increases, and the powder surface becomes rough. It is common that a wide size distribution is observed in the gas atomized powder. The cooling rate during gas atomization is roughly proportional to 1/d, where d is the powder particles size[10]. The cooling rate of hypoeutectic Al-Si alloys under the experimental conditions is estimated to be in the range of 103-105 K/s.
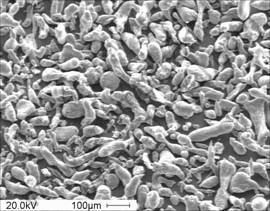
Fig.2 Morphology of gas-atomized powders
Table 1 lists the density of various powder particles. From the table we can find the density of powders increases with decreasing powder size. The average actual and relative density of various powders are 1.13 g/cm3 and 40.9%, respectively.
Table 1 Density of various powder particle
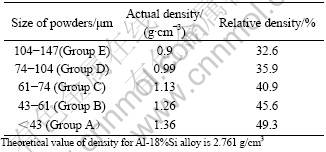
Figs.3(a)-(e) show the optical micrographs taken from the groups A-E powder particles, respectively. The optical micrographs from the groups B-E powders show a distribution of crystalline phases particles with a size below 5 μm. Based on observation, it can be concluded that the precipitate particles are of Si embedded in α(Al) matrix. The optical micrograph from group A powder shows a microstructure of Si supersaturated in α(Al) matrix.
3.1 Performance of cold compacting
During cold compacting, different powder particles are compacted as ingot by double direction cold compacting respectively. The results show that powders from group D and E represent good performance of cold compacting and the ingot could be withdrew from the die easily. The surfaces of the ingot of powders from group A, B and C are rough, and the ingot is withdrew from the die difficultly, even broken into pieces.
The morphology of gas-atomized powders shows that the smaller the powders, the more smooth and spherical the surface. Because of the large specific surface area, the percentage of oxygen of small powders is higher than that of big powders and the plasticity of small powders is worse than that of big powders. On the other hand, the film of oxide makes the small and spherical powders be compacted difficultly, whilst the big and irregular powders can be compacted easily by the way of coherence and grip.
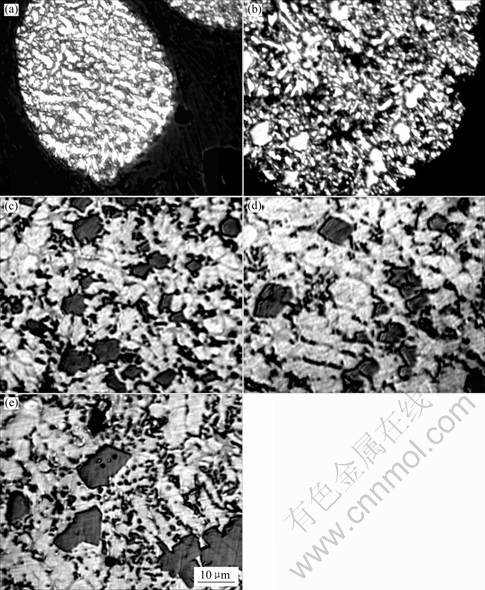
Fig.3 Microstructures of alloy powders with different sizes: (a) <43 μm (Group A); (b) 43-61 μm (Group B); (c) 61- 74 μm (Group C); (d) 74-104 μm(Group D); (e) 104-147 μm(Group E)
3.2 Resistance to plastic flow
Figs.4(a) and (b) show the optical morphologies exhibiting the structure of the longitudinal and transverse section of the Al-18%Si alloy bar extruded from the groups of A and E powders coated with aluminum film. Here, the resistance to plastic flow is found to be almost equal for the different powders. During extrusion, the flow of the powder particles occurs from the periphery to the center of the billet prior to entering into the extrusion die. The center part of the billet moves forward more rapidly than the peripheric part[10]. As the latter units enter the deformation zone, the powder particles are first of all compressed and then pass diagonally with heavy shearing into the die. Hence, the central elements undergo minimum deformation mainly by compression of the powder particles corresponding to the extrusion ratio; whilst the layers near the surface undergo greater shear deformation and are stretched out along the periphery of the bar.
3.3 Density of alloy bar
Table 2 lists the density of alloy bar of various powders with different processes. From the table we can find the density of alloy bar increases with decreasing powder size in hot press. The average relative density of various powders is 98.4%, and the relative density of the mixed powders is 98.2%. In hot extrusion, the density of alloy bar increases with decreasing powder size when the area reduction ratio(λ) is 4, but the density of alloy bar decreases abnormally for powders smaller than 43 μm(Group A), which may be attributed to the cracking of the bar. The average relative density of various powders is 98.7%, and the relative density of the mixed powders is 98.2%. Average relative density of alloy bar is 99.5% from group B, C and D powders and 98.1% from group E and F powders when λ is 19, and the relative density of the mixed powders is 99.4%.
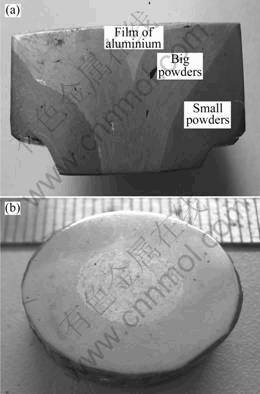
Fig.4 Optical morphologies of longitudinal (a) and transverse (b) section of alloy bar extruded from different powders with reduction ratio of 4
Table 2 Density of alloy bar with different processings
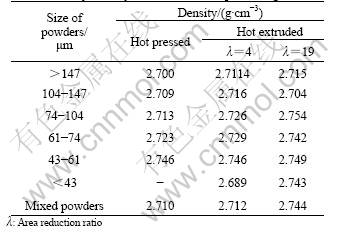
The variation of density of alloy bar with different processes shows that in hot press, the film of oxide wrapped on the surface of powder particles can not be broken completely, which makes that the powder particles can not touch each other with fresh metallic surface, being the trapped oxide, so the density of alloy bar is small. In hot extrusion, especially when area reduction ratio is 19 or more, the film of oxide is broken and the powder particles are compacted completely under intense three-dimensional compressive stress.
3.4 Process defect
Figs.5(a) and (b) show the cracking defect of the powder particles during extrusion process(λ=4), which is found to be sensitive to the powder particle size.
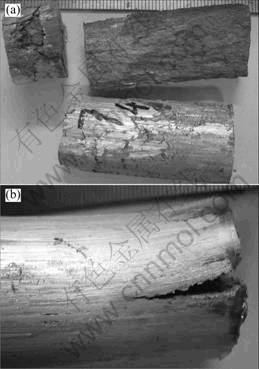
Fig.5 Process defect of alloy bar from powders <43μm(Group A) (a) and 74-104 μm(Group D) (b) with area reduction ratio of λ=4
Fig.5(a) shows that the alloy bar from group A is broken into pieces. Fig.5(b) shows that cracking always occurs at the top piece of the alloy bar from group D. Actually, cracking of the bar from group B, C and D powders almost occurs at the top piece. The alloy bars from group E and F powders have good quality without cracking.
Consequently, from the view point of performance of cold compacting, the bigger the powder particles, the better the performance of cold compacting. In the meantime, the quality of alloy bar extruded from big powder particles is good enough without cracking. But from the view point of density, the smaller the powder particles, the higher the density of the alloy bar. On the other hand, it is reported that the microstructure and mechanical property of small powder particles are better than those of big particles.
For practice application, mixed powders are better than single powders. From the test, the mixed powders should be the powders from group A, B, C, D and E. In order to improve the quality of alloy bar, some methods should be used in hot extrusion, such as coating with aluminum film, lubricating, increasing temperature of extrusion and area reduction ratio (>19).
4 Conclusions
1) Gas atomized Al-18%Si alloy powders show different microstructures and morphologies depending on the powder size. The behavior of the powder particles during extrusion is a function of the powder size.
2) The bigger the powder particles, the better the performance of cold compacting. The quality of alloy bar from big powders is good enough without cracking.
3) The smaller the powder particles, the higher the density. The microstructure and mechanical properties of small powders are better those of big powders.
4) For practice application, mixed powders are better than single powders.
References
[1] KIM T S, HONG S J, KIM W T, WON C W, CHO S S, CHUN B S. Microstructures and mechanical properties of Al-20Si-xFe(x=3, 5, 7) alloys manufactured by rapid solidification processing[J]. Materials Transactions JIM, 1998, 39(12): 1214-1219.
[2] OTSUKI M, KAKEHASHI S, KOHNO T. Mechanical properties of powder forged, rapidly solidified aluminium alloy parts[J]. Metal Powder Report, 1991(4): 30-32.
[3] TAKEDA Y, HAYASHI T, ODANI Y, AMANO N, KUROISHI N. Hot forge ability of hot extruded P/M Al-Si-Fe-X alloys[A]. Proc Int Powder Metall Conf[C]. 1988: 533-545.
[4] GALANTY M, KAZANOWSKI P, KANSUWAN P, MISIOLEK W Z. Consolidation of metal powders during the extrusion process[J]. Journal of Materials Processing Technology, 2002, 125/126: 491-496.
[5] SENTHILVELAN T, RAGHUKANDAN K, VENKATRAMAN A. Development of nomograms for prediction of extrusion stress for P/M copper preforms at warm working temperatures[J]. Journal of Materials Processing Technology, 2003, 138: 28-33.
[6] SENKOV O N, MIRACLE D B, SCOTT J M, SENKOVA S V. Equal channel angular extrusion compaction of semi-amorphous Al85Ni10Y2.5La2.5 alloy powder[J]. Journal of Alloys and Compounds, 2004, 365: 126-133.
[7] ABOUELMAGD G. Hot deformation and wear resistance of P/M aluminium metal matrix composites[J]. Journal of Materials Processing Technology, 2004, 155/156: 1395-1401.
[8] MORSI K, MCSHANE H B, MCLEAN M. Processing defects in hot extrusion reaction synthesis[J]. Materials Science and Engineering A, 2000, 90: 39-45.
[9] SENKOV O N, SENKOVA S V, SCOTT J M, MIRACLE D B. Compaction of amorphous aluminum alloy powder by direct extrusion and equal channel angular extrusion[J]. Materials Science and Engineering A, 2005, 393: 12-21.
[10] HONG S J, CHUN B S. Extrusion behavior of gas atomized nanostructured Al88.7Ni7.9Mm3.4 alloy powders[J]. Materials Science and Engineering A, 2003, 348: 262-270.
(Edited by YANG Bing)
Foundation item: Project(514120203) supported by the Advanced Investigation Foundation of Weapon Equipment
Corresponding author: MAO Xie-min; Tel: +86-21-56331469; E-mail: xmmao@sh163.net