
Disposal of cuprous chloride waste water
XUE Juan-qin(薛娟琴), MAO Wei-bo(毛维博), WANG Yu-jie(王玉洁),
LI Jing-xian(李京仙), WU Ming(吴 明), LU Xi(卢 曦)
School of Metallurgical Engineering, Xi’an University of Architecture and Technology, Xi’an 710055, China
Received 6 July 2009; accepted 30 December 2009
____________________________________________________________________________________________
Abstract: The waste water system generated in the process of production of cuprous chloride was studied. The existing forms of copper in the system and the influence of temperature and pH on the existing forms of copper ion were analyzed and determined through calculating the coefficients of copper complex distribution. In the waste water system, the main forms of copper are CuSO4, Cu2+, CuCl+,
and
. Temperature has little influence on the distribution coefficient of Cu(Ⅱ), but has significant influence on distribution coefficient of Cu(Ⅰ). With the increase of temperature, the distribution coefficient of
increases significantly while the distribution coefficient of
decreases. The pH has nearly no influence on the distribution coefficients of various Cu(Ⅰ)-compounds, but has sizable influence on the distribution coefficients of Cu(Ⅱ)-compounds. With the increase of pH, the distribution coefficient of CuSO4(aq) increases while the distribution coefficients of Cu2+ and CuCl+ decrease. According to these results, the anion resin of 201×7 OH- and the cation resin of 732 Na were chosen to dispose the waste water solution of cuprous chloride. Finally, 97.9% copper in the waste water is recovered.
Key words: waste water solution; cuprous chloride; copper complex; coefficient of distribution; ion-exchange
____________________________________________________________________________________________
1 Introduction
Due to the unique physical and chemical properties, CuCl has been widely used. For example, it has been used as the catalyzer in organic synthesis, the reducer in lipid chemical industry, the discolorant, desulfurizer and deviator in oil chemical industry. In addition, it has also been widely used in the industry of dyes, metallurgy, plating as well as medicine and pesticide[1-2]. The production methods of CuCl are mainly bluestone deoxidization, direct oxidation of waste copper chlorine, bras wire oxidation and the waste copper hydrochloric acid, etc[3]. The waste water generated in the production process often contains copper of 2-3 g/L, which should be recycled. Currently, the widely used recycling method is first reverting bivalent copper into univalent copper by using industrial waste scrap iron, then removing the bivalent iron from the solution by using anti-infiltration membrane method and finally returning the disposed solution to production for recycling utilization. However, this method has some shortcomings, such as the complex working procedure, the poor disposal efficiency and the disturbing ions introduced into the solution. Therefore, this method has a bad economical efficiency and a bad environmental efficiency[4-5].
In order to find a better disposal method for CuCl waste water, which has a simple process and high recycling rate of copper, in this work, the compound bond theory was used to explain the reason why the CuCl solubility in water solution is low, and to study the structure and stability of the compound formed by Cl- with Cu(Ⅰ) and Cu(Ⅱ). From the solution thermo- dynamics and multi-ion balance theory, the distribution coefficients for the particles of different forms in the CuCl waste water system were calculated and analyzed, and the influence of the concentration of Cl- in the solution, the temperature and the pH value on the distribution coefficients of Cu compounds was studied.
2 Theory of Cu(Ⅰ)-Cl- and Cu(Ⅱ)-Cl- compound bond
Because the copper atom has the structure of 1s22s22p63s23p63d104s1, Cu+ with outermost layer electron structure of 3d10 is formed when the atom loses an electron. Here, the outermost layer is filled with electrons and thus Cu+ compound has some different structures and properties with other transition metal ion compounds. The solid CuCl has a similar ion distance with NaCl, whereas CuCl has a larger experimental crystal lattice energy. About the variation of hydration heat with ion radius, for non-transition elements, the ion radius and hydration heat have an inverse ratio; but for transition metal ions Cu(Ⅰ) and Na(Ⅰ), which have the same radius and electric charge, the hydration heat of copper is larger than that of natrium by 40% and their ionization potentials are also different (496.04 kJ for Na and 745.1 kJ for Cu(I))[6]. The main reason for the property difference between Cu(Ⅰ) and Na(Ⅰ) is the difference of 8 electrons from 18 electrons in the outermost layer. For the spherically symmetric distribution of electric charge, the electric charge locates outside at one point (relatively far from the charge center), which generates a lower static electric potential compared with the case that the electric charge locates at this point. Therefore, in a transition system, the increased positive electric potential caused by the increase of the nuclear electric charge is not possibly balanced by the added electron negative potential. This is called non-complete screening of nuclear electric charge. So, for a large distance Cu(Ⅰ) and Na(Ⅰ) have the same electric potential, while for a bond distance the copper has a larger effective ion charge. This is because the electrons on d-orbit tend to appear at a distance larger than bond distance from the copper nucleus. Cu(Ⅰ) has an effective charge q′ larger than +1. Even for ion bonding, this large charge can obviously affect the bonding energy. If the bonding element (say Cl-) is low electrically negative, the energy is in favor of the transition of the electron from the bonding element to the metal. Such transferred electric charge still obtains a large effective charge. In a word, the d-electron of the spherical d10 ion has non-complete screening for nucleus and results in the tendency of covalent bonding when it bonds with some low negatively charged element. This makes Cu+ and Cl- easily separate out from water solution(
is 1.7×10-7 for CuCl).
In the theory of soft-hard acid alkali[7], Cu+ belongs to soft acid and its bonding with Cl- results in a high polarization rate, which corresponds to a low ionization potential and makes for the strong transfer of electrons to Cu+ form Cu+-Cl- compound with obvious covalence stability. The bonding form of soft acid Cu+ with Cl- can be explained by π-bonding theory[6]. Cu+ has an electronic layer structure of 1s2 2s22p63s23p63d10, whose feature is the existence of the loosely bound outer d-orbit electrons. Since Cl- has an electronic layer structure of 1s22s22p63s23p63d10, the d-electrons of Cu+ can easily form into π-bond on the empty d-orbit of Cl-. In other words, in the formation of π-bond, Cu+ is the potential d-electron provider while Cl- is the accepter. In this way, the binding of Cu+ and Cl- not only forms σ-bond on the mixed orbit, but also leads to the tendency for the binding of feedback π-bond of d-electron of Cu+, as shown in Fig.1.
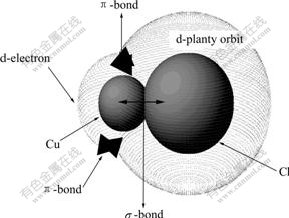
Fig.1 Bonding mode of coordinated complex of Cu(Ⅰ)-Cl-
Cu2+ has an electronic layer structure of 1s22s22p63s23p63d9 and belongs to medium acid in the theory of soft-hard acid alkali. When it binds with Cl-, unlike the case of Cu+, it cannot obtain extra stability. Therefore, although in the theory of valence the binding of both Cu2+ and Cu+ with two or three Cl- results in sp hybridization and sp2 hybridization, which forms the compound of linear type and plane triangle structure, their stabilities have much difference in the solution:
and
have obviously higher stability 

).
Among the compounds formed by Cu(Ⅰ) and Cu(Ⅱ) with Cl-, besides the existence of CuCl,
,
,
, CuCl2 and
, Cu(Ⅱ) can also bind with Cl- to form 

[8-9]) with plane square structure. These compounds are hard to explain in terms of valence theory. In the crystal lattice theory, unlike in the valence theory, d9 orbital Cu(Ⅱ) ion cannot form tetrahedron or octahedron compound. Due to John-Teller effect, the four radicals locate at one plane and close to Cu(Ⅱ) ion while other two radicals are perpendicular to the plane and far from the ion, which finally forms
with a square structure, as shown in Fig.2[8].
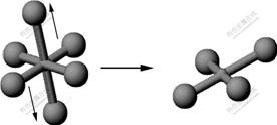
Fig.2 Structure of plane square coordinated complex formed by John-Teller effect
3 Calculation of distribution coefficient of Cu compound in cuprous chloride waste water
3.1 Calculation and analysis of distribution coefficient of Cu compound in Cu-Cl--H2O system
In the water solution Cu+ can be easily oxidized to Cu2+ and is unstable. Due to the strong binding power of Cu+ and Cl-[9-10], Cu+ can exist stably in the solution containing Cl-. Neglecting the oxidation of Cu+, the binding reactions between Cu2+ and Cl- and Cu+ and Cl- exist in the Cu-Cl--H2O solution. Such binding reactions and the balance constants at 25 ℃ and 60 ℃ are shown in Table 1.
Table 1 Bonding reaction balance constants for Cu(Ⅰ)-Cl- and Cu(Ⅱ)-Cl- at 25 ℃ and 60 ℃[8-9]
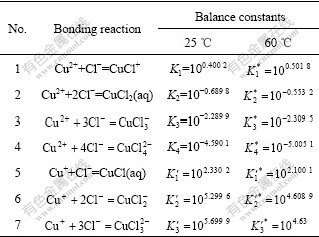
From Eqs.(1)-(7) the contents of Cu(Ⅱ), Cu(Ⅰ) and Cl- in the solution can be obtained as:


(8)

From the definition of distribution coefficient [11-12], with only two values of [Cl-]T, [Cu(Ⅱ)]T and [Cu(Ⅰ)]T, the relation for the other value was obtained with the distribution coefficient. In this work, the common deoxidation method[13] was used to make out cuprous chloride powder to obtain waste water solution through one experiment. Then the state-standard analysis method [14] was used to measure the concentration of [Cu(Ⅰ)]T. atom absorption method(AA-6800 atom absorption spectrophotometer) was used to measure the concentration of Cu. The AgNO3 method[12] was used to measure the concentration of Cl-. Through the calculation using Math-cad[15], the relation of the distribution coefficient for [Cl-]T and Cu(Ⅱ)-Cl-, Cu(Ⅰ)-Cl- at 25 ℃ and 60 ℃ with [Cu(Ⅱ)]T=0.007 6 mol/L and [Cu(Ⅰ)]T = 0.024 mol/L, are shown in Figs.3- 6.
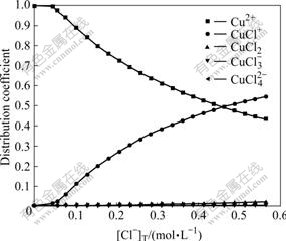
Fig.3 Effect of total concentration of Cl- on distribution coefficients of coordinated complexes of Cu(Ⅱ)-Cl- at 25 ℃
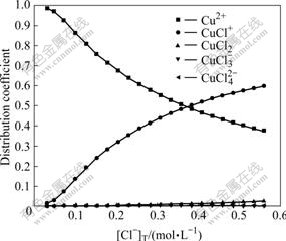
Fig.4 Effect of total concentration of Cl- on distribution coefficients of coordinated complexes of Cu(Ⅱ)-Cl- at 60℃
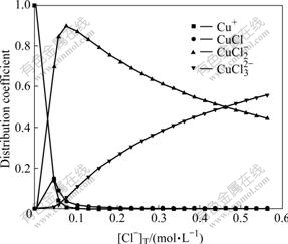
Fig.5 Effect of total concentration of Cl- on distribution coefficient of coordinated complexes of Cu(Ⅰ)-Cl- at 25 ℃
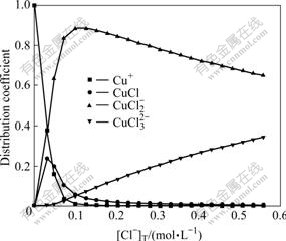
Fig.6 Effect of total concentration Cl- on distribution coefficient of coordinated complexes of Cu(Ⅰ)-Cl- at 60 ℃
From Figs.3 and 4, it can be seen that at 25 ℃, when [Cu(Ⅰ)]T is 0.024 mol/L, [Cu(Ⅱ)]T is 0.007 6 mol/L and when [Cl-]T is in the range of 0-0.566 mol/L, the distribution coefficient of Cu2+ drops with [Cl-]T increasing and is 0.58 at [Cl-]T=0.345 2 mol/L. The distribution coefficient of CuCl+ increases with [Cl-]T increasing and is about 0.41 at [Cl-]T= 0.345 2 mol/L. In addition, the distribution coefficients of CuCl2 is very small in the whole range, slowly going up with [Cl-]T increasing, and is 0.01 when [Cl-]T=0.345 2 mol/L; while the distribution coefficients of
and
are almost zero in the whole range. At 60 ℃, the distribution coefficients have no much difference with those at 25 ℃, which is slightly higher for CuCl+ and slightly lower for Cu2+. This indicates that as temperature goes up, it is easier for Cu2+ to bind with Cl- to form CuCl+. From these analyses, Cu(Ⅱ) mainly exists in the forms of Cu2+ and CuCl+ at 25 -60 ℃.
From Figs.5 and 6, it can be seen that, at 25 ℃, when [Cu(Ⅰ)]T is 0.024 mol/L, [Cu(Ⅱ)]T is 0.007 6 mol/L, Cl- starts to appear in the solution, and Cu+ binds with Cl- immediately. When [Cl-]T is 0.345 2 mol/L, the distribution coefficient of Cu+ is almost zero. The distribution coefficient of CuCl(aq) increases with [Cl-]T increasing, goes up at first (reaches 0.15 when [Cl-]T is 0.04 mol/L) and then drops and finally tends to zero gradually. When [Cl-]T is 0.345 2 mol/L, CuCl(aq) has a distribution ratio of 0.002. The distribution ratio of
first increases rapidly with [Cl-]T increasing (reaches 0.898 when the Cl- concentration is 0.074 mol/L), then drops down and becomes 0.58 when [Cl-]T is 0.345 2 mol/L. The distribution coefficient of
increases gradually with the [Cl-]T concentration and reaches 0.418 when [Cl-]T is 0.345 2. The distribution coefficient at 60 ℃ show little difference with the values at 25 ℃. When [Cl-]T is low, as the temperature goes up, the distribution coefficient of CuCl(aq) increases. When [Cl-]T is smaller than 0.1 mol/L,
has a slightly larger distribution coefficient at 25 ℃ than at 60 ℃. When [Cl-]T is larger than 0.1 mol/L,
has a much smaller distribution coefficient at 25 ℃ than at 60 ℃. In the whole range of [Cl-]T,
has an obviously larger distribution coefficient at 25 ℃ than at 60 ℃. So, from above results, it can be seen that Cu(I) exists in the forms of
and
in the waster water at 25-60 ℃.
3.2 Influence of other ions in waste water on existing form of Cu
In the waste water of cuprous chloride, besides the existence of Cu2+, Cu+, Cl-, there are
, Na+ and H+ with certain concentration which affect the existing forms of Cu(Ⅰ) and Cu(Ⅱ) in the solution[16]. These six ions have the reactions in Eqs.(1)-(7) and, in addition, have the following balance reactions as shown in Eqs.(11)-(14) with the balance constants at 25 ℃[8-9]. Then, five relations (Eqs.(15-19)) can be obtained. At the same time, assuming no loss of
and Na+ in the experimental process, [
]T=0.261 mol/L and [Na+]T=0.71 mol/L can be obtained through calculation. Through measurement, the initial pH value of the solution is 2.6(PB-10 acidity meter). Then, from the definition of distribution coefficient and through the calculation by Mathcad, the relation graphs shown in Figs.7 and 8 are obtained.
Na++Cl-=NaCl(aq), K(NaCl)=10-0.777 (11)
,
(12)
,
(13)
,
(14)


(15)

(16)

(17)

As shown in Fig.7, compared with the system of Cu-Cl--H2O, two kinds of ions
and Na+ have much influence on the distribution coefficients of Cu(Ⅱ) compounds. Except for the case with a low pH, in which Cu+and CuCl+ have large distribution coefficients, Cu(Ⅱ) mainly exists in the form of CuSO4(aq) in the solution. As the pH increases, the distribution coefficient of CuSO4(aq) increases.
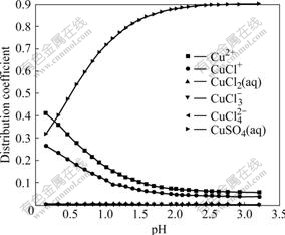
Fig.7 Effect of pH on distribution coefficients of coordinated complexes of Cu(Ⅱ)-Cl- at 25 ℃
The initial pH is 2.6 and the distribution coefficient of CuSO4(aq) is 0.9. Under the same condition, the distribution coefficients of Cu2+ and CuCl+ which take up 99% in the Cu-Cl-H2O system, are only 0.1. The main reason is that the binding power of Cu2+ and
is strong and the concentration of
in the solution is high.
As shown in Fig.8, the variation of pH has little effects on the distribution coefficients of Cu(Ⅰ) compounds. When pH is 2.6, the distribution coefficients of
and
are 0.599 and 0.398, respectively. As mentioned above, if there are no SO42- and Na+ in the solution,
and
have distribution coefficients of 0.58 and 0.418, respectively. This indicates that adding
and Na+ in the Cu-Cl-H2O system has little influence on the distribution coefficients of Cu(Ⅰ) compounds. The main reason is that the binding power of Cu(Ⅰ) with 2 or 3 Cl- is strong. In the solution Cu(Ⅰ) mainly exists in the form of
and
The above analytical results are in agreement with the experimental results[17-18].
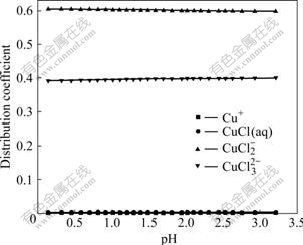
Fig.8 Effect of pH on distribution coefficients of coordinated complexes of Cu(Ⅰ)-Cl- at 25 ℃
4 Conclusions
1) From the study of matching bond theory, the non-complete screening of the d-electrons of the spherical d10 structure of Cu(Ⅰ) to the nucleus leads to the fact that its binding with low negatively charged Cl- tends to covalent bond. This makes CuCl have a low solubility and easy to separate out as sedimentation. Because the binding mode of Cu(Ⅰ) and Cl- can form the σ-bond on the mixing orbit and, at the same time, there exists the tendency of the feedback π-bond of the d-electron of Cu+, the compounds of Cu(Ⅰ) and Cl- have higher stability than the compounds of Cu(Ⅱ) and Cl-.
2) Through the calculation and analysis for the distribution coefficients of Cu compounds in the cuprous chloride waste water, the main existing forms of copper are
Cu2+, CuCl+, 
. As [Cl-]T increases, the distribution coefficients of CuCl+ and
increase while the distribution coefficients of Cu2+ and
decrease. The temperature has little influence on the distribution coefficient of Cu(Ⅱ) compounds. As the temperature increases, the distribution coefficient of CuCl+ increases slightly and the distribution coefficient of Cu2+ decreases slightly. The temperature has obvious influence on the distribution coefficients of Cu(Ⅰ) compounds. As the temperature increases, the distribution coefficient of
increases sizably and the distribution coefficient of
decreases sizably. The pH value has almost no influence on the distribution coefficients of Cu(Ⅰ) compounds, but has sizable influence on the distribution coefficients of Cu(Ⅱ) compounds. As pH increases, the distribution coefficient of
increases while the distribution coefficients of Cu2+ and CuCl+ decrease.
References
[1] WANG Pei-xi, WANG Yan, ZHAO Hong. The application and preparation technology progress of cuprous chloride[J]. Inorganic Chemicals Industry, 2004, 36(2): 10-12. (in Chinese)
[2] HUANG Ling-tao, LIU Ding-fu, ZENG Xiang-qin, WANG Long-mian. Study on the preparation of cuprous chloride from copper-containing waste water[J]. Inorganic Chemicals Industry, 2008, 40(4): 46-48. (in Chinese)
[3] LIU Tuan-chi, CHANG Chung-shui. Preparation of CuCl/C catalyst for the synthesis of dimethyl carbonate: Effects of calcination temperature[J]. Journal of the Chinese Institute of Chemical Engineers, 2007, 38: 29-34. (in Chinese)
[4] LEE J C, ZHU T, JHA M K, KIM S K, YOO K K, JEONG J. Solvent extraction of Cu(I) from waste etch chloride solution using tri-butyl phosphate (TBP) diluted in 1-octanol[J]. Separation and Purification Technology, 2008, 62: 596-601.
[5] ASTNER J, WEITZER M, FOXON S P, SCHINDLER S, HEINEMANN F W, MUKHERJEE J. Syntheses, characterization, and reactivity of copper complexes with tridentate N-donor ligands[J]. Inorganica Chimica Acta, 2008, 361: 279-292.
[6] DEBORDE M, von GUNTEN U. Reactions of chlorine with inorganic and organic compounds during water treatment—Kinetics and mechanisms: A critical review[J]. Water Research, 2008, 42: 13-51.
[7] ZHANG Xiang-lin, KANG Heng. Bonding Chemistry[M]. Changsha: Central South University of Technology Press, 1986. (in Chinese)
[8] Inorganic Chemistry Group, Dalian Institute of Technology. Inorganic Chemistry[M]. Beijing: Higher Education Press, 2001. (in Chinese)
[9] LIU Wei-hua, McPHAIL D C. Thermodynamic properties of copper chloride complexes and copper transport in magmatic-hydrothermal solutions[J]. Chemical Geology, 2005, 221: 21-39. (in Chinese)
[10] FU Cheng-shuo, ZHENG Di-ji. Thermodynamical analyses and electric potential-pH graph for Cu-Cl-H2O system[J]. Nonferrous Metal, 1980(2): 18-27. (in Chinese)
[11] ZHOU Xing-yao, REN Jian-guo. Ion balance in analytical chemistry[M]. Being: Science Press, 2000. (in Chinese)
[12] Wuhan University. Analytical Chemistry[M]. 4th Ed. Beijing: Higher Education Press, 2000. (in Chinese)
[13] PENG Ji-shi, FAN Xing-yong. A joint method for producing cuprous chloride: CN94105209.5 [P]. 1994-05-17. (in Chinese)
[14] LU Wei-lin, YANG Chun-sheng. Titration of cuprous chloride[J]. Analytical Chemistry, 1998(1): 117. (in Chinese)
[15] HAO Li-ren, LI Bao-lin, HE Feng-lan, HAO Zhe-ou. Mathcad 2001 and probability application [M]. Beijing: Chinese Hydroelectric Press, 2002. (in Chinese)
[16] OISHI T, YAGUCHI M, KOYAMA K, TANAKA M, LEE J C. Hydrometallurgical process for the recycling of copper using anodic oxidation of cuprous ammine complexes[J]. Electrochimica Acta, 2008, 53: 2585-2592.
[17] LUNDSTR?M M, AROMAA J, FORS?N O. Redox potential characteristics of cupric chloride solutions[J]. Hydrometallurgy, 2009, 95: 285-289.
[18] XUE Juan-qin, YANG Juan-juan, DU Shi-yi, SHEN Bin-bin, MENG Ling-ai. Experimental research on waste water containing cuprous chloride disposal using ion exchange-resin[J]. Nonferrous Metals, 2008(5): 2-5. (in Chinese)
_______________________________
Foundation item: Projects(50874087; 50978212) supported by the National Natural Science Foundation of China; Project(2006E106) supported by Natural Science Foundation of Shaanxi Province, China; Project(07JK302) supported by Special Natural Science Foundation of Shaanxi Province Education Office, China
Corresponding author: XUE Juan-qin; Tel: +86-13186038833; E-mail: huagong1985@163.com
(Edited by YUAN Sai-qian)