Trans. Nonferrous Met. Soc. China 26(2016) 2672-2678
Effect of glass fibre (GF) addition on microstructure and tensile property of GF/Pb composites fabricated by powder metallurgy
Yao-hong GENG1,2, Peng-hu WANG1
1. Materials Science and Engineering College, Northeast Forestry University, Harbin 150040, China;
2. School of Naval Architecture and Ocean Engineering, Harbin Institute of Technology at Weihai, Weihai 264209, China
Received 16 May 2016; accepted 31 August 2016
Abstract: GF/Pb composites were fabricated by the method of powder metallurgy, and the density, microstructure and tensile property were characterized considering the size and content of glass fibre (GF). The results show that relative densities decrease with increasing GF fraction, and the 50 μm-GF reinforced specimens exhibit a better densification than the 300 μm-GF reinforced ones. The GF particles distribute quite uniformly in Pb matrix, and the composites fabricated at low sintering temperature (<200 °C) possess fine-grain microstructure. The addition of GF significantly improves the strength of the Pb composites, and the ultimate tensile strength of the Pb composite reinforced with the addition of 50 μm-0.5% GF (mass fraction) is about 30 MPa higher than that of GF-free sample. For all composites groups, increasing the reinforcement content from 0.5% to 2% (mass fraction) results in a decrease in both tensile strength and ductility.
Key words: GF/Pb composites; powder metallurgy; sintering microstructure; tensile property
1 Introduction
Pb-based alloys have been extensively investigated over the past few decades as a candidate material in electric vehicles, architecture field and nuclear power industry because of their excellent properties, such as plastic deformability, high conductance, corrosion, noise and radium resistance characteristics [1-4]. However, their insufficient strength and low hardness seriously restrict their practical applications [5].
The strength of the Pb alloy could be effectively improved by introducing the stiff and hard particle reinforcements, such as glass fibre (GF) [6], Ti3SiC2 [7] and MnO2 [8]. GF possesses useful properties such as good machinability, low density, excellent thermal shock and high-temperature oxidation resistance [9,10]. Moreover, it has been reported that the thermal expansion coefficient of GF is close to that of Pb matrix, which would largely avoid the phenomenon of segregation of the fibre particles and reduce the internal stress between fibre and Pb matrix during the fabrication of the composites [11]. The combination of GF and Pb metal can provide a superior class of the composites with high strength and other properties. SUN et al [12] fabricated GF/Pb composite wire by forward extrusion method and a side way extrusion method. The tensile strength of the composites was measured in the range of 62-180 MPa, and the conductivity was in the range of (31.6-32.1)×10-8 Ω·m. The addition of high volume fraction of the continuous GF should be the main reason for the high strength. However, the effect of non-continuous GF on the Pb matrix composite has less been investigated until now and it would be more complex than that in the continuous GF/Pb composite. Compared with extrusion method, powder metallurgy (PM) technique is a simple and low cost production method for producing near-net shape composites [13,14]. Moreover, the PM is feasible to add GF reinforcement within a wide range of volume fraction.
In the present work, the GF/Pb composites with good tensile properties through the addition of two kinds of short GF were prepared with the method of powder metallurgy processes including mixing and hot-pressing. The effect of the GF size and the content on the fabrication was investigated. Simultaneously, in order to provide a deeper insight into the intrinsic properties of the GF/Pb composite, the effect of GF addition on the tensile properties of the GF/Pb composites was evaluated. It is expected that the GF/Pb composites possess excellent strength without sacrificing ductility.
2 Experimental
2.1 Composite preparation
The raw materials were made from commercial powders of Pb (99.0% in purity, and about 47 μm) and two-sized GF (99.5% in purity, approximately 300 and 50 μm in length, and 12 μm in diameter). The morphology of the raw powders is shown in Fig. 1. Elemental powder blends corresponding to nominal Pb-(0.5, 1.0, 1.5, 2.0)%GF composites with two kinds of short GF particles were mixed mechanically at room temperature. The powder mixtures were subjected to double action axial compaction into square compacts using a stainless steel die under 150 MPa for 5 min. The green compact with a dimension of 50 mm × 50 mm × 10 mm was placed in a graphite crucible followed by sintering in a vacuum sintering furnace. Then, the compacts were sintered at a heating rate of 10 °C/min and with the exertion of a gradual increasing pressure in a vacuum furnace under a vacuum better than 1×10-3 Pa. The temperatures of 100, 150, 200, 250 °C and a pressure of 50 MPa were acquired nearly simultaneously, and the temperature and pressure were held constant for 2 h, followed by furnace cooling.
2.2 Material characterization
The densities of the GF/Pb composites were measured by the Archimedes method with a very thin film of vaseline on each specimen to avoid the permeation of water. The detailed microstructures were studied by scanning electron microscope (SEM, Quanta 200 FEG) using secondary electrons imaging. Tensile specimens with a gauge length of 18 mm and a section area of 4 mm × 1 mm were machined from the compacts. Tensile tests were carried out in air on American Instron 5569 testing machine at a strain rate of 1 mm/min at room temperature. All specimens for microstructure observation and tensile tests were taken along the middle of the fabricated samples. In tensile experiments, at least 2 specimens were tested for each composite.
3 Results and discussion
3.1 Relative density of GF/Pb composites
Figure 2 shows the effect of GF fraction and sintering temperature (200 °C) on the relative density of the GF/Pb composites after vacuum hot-press sintering. It is found that the highest relative density is 99.8% for Pb-0.5%GF (50 μm) composites, while the lowest relative density value is 97.3% for Pb-2%GF (300 μm) composites. The relative density decreases with increasing GF reinforcement content. In addition, the 50 μm-GF reinforced specimens exhibit a better densification than the 300 μm-GF reinforced ones, especially for the composites with high GF reinforcement fractions. This result indicates that decreasing the particle size of GF is an effective way to reduce the microvoids between the GF and Pb matrix. Since the pores mainly exist at the GF/Pb interface, the condition of interface bonding directly affects the composite density.
3.2 Microstructure of sintered GF/Pb composite
SEM observations of the typical microstructure and the statistics of the grain size distribution of Pb-1%GF (50 μm) composite produced by sintering at 150 °C for 2 h are shown in Fig. 3. The GF particles uniformly distribute in the Pb matrix, and, as indicated in the inset of Fig. 3(a), the GF particles are integrated tightly with Pb matrix and grow inside partially. As shown in Fig. 3(b), in the Pb matrix, the nearly equiax-shaped grains can be observed and the grain boundaries are relatively clear, indicating that dynamic recrystallization occurs during the sintering process. The histogram in Fig. 3(c) shows the grain size distribution of the Pb matrix, which is summarized from 200 grain diameters. After calculation, the mean grain size of Pb matrix grains is 14.47 μm, mostly scattering from 2 to 25 μm, and more than 80% of the grains are refined to less than 25 μm. We speculate that the grain refinement of the composite could be attributed to the optimal processing parameters for the powder materials.
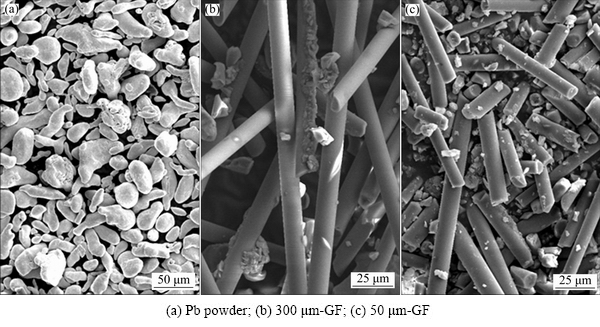
Fig. 1 Morphologies of starting powders
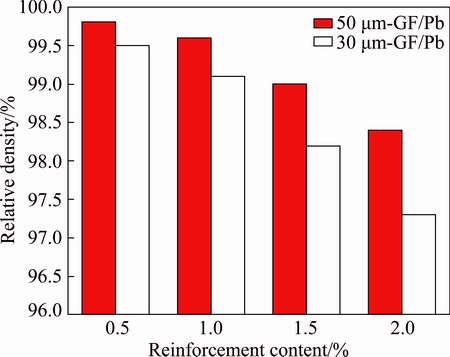
Fig. 2 Effect of reinforcement content on relative density of GF/Pb composites sintered at 200 °C for 2 h
Figure 4 shows the microstructure of the Pb-x%GF composites. As indicated, the GF particles have a rod-like shape and exhibit a uniform distribution in the Pb matrix. The GF reinforcement particles are dispersed within the Pb matrix in two ways in the sintered composite, as can be seen in Fig. 4. Part of the GF is perpendicular to the Pb substrate, while the others of the GF particles are dispersed parallel in the composites, especially with higher content of GF reinforcement particles. The mechanical properties of the Pb-x%GF composites could be improved by the cross type grating structure.
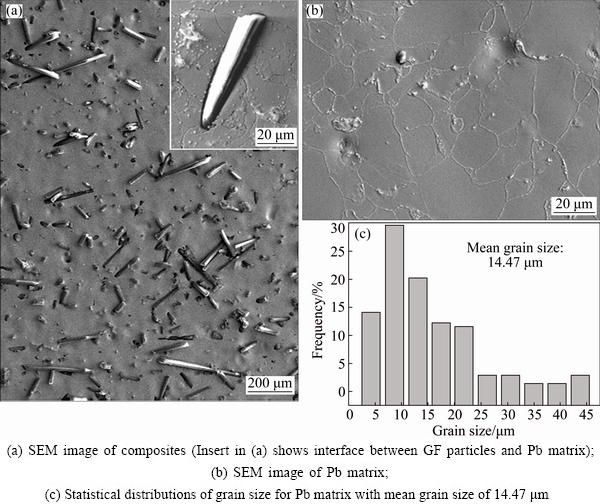
Fig. 3 Typical microstructure and grain size statistical distributions of Pb-1%GF (50 μm) composite sintered at 150 °C for 2 h
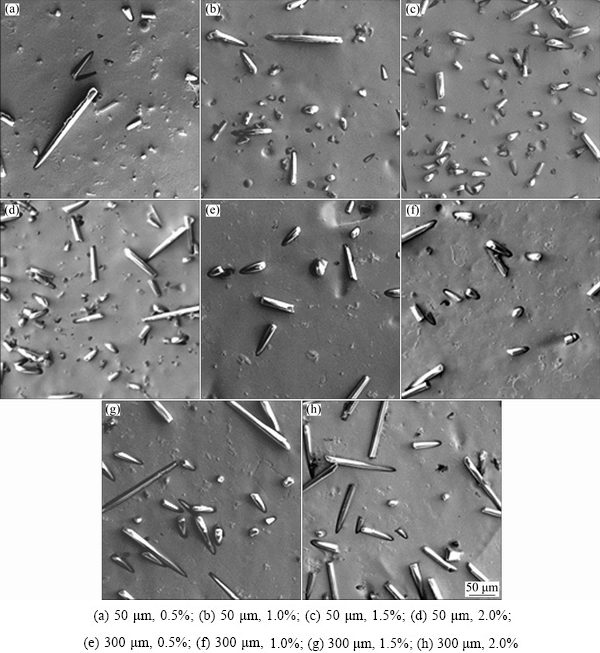
Fig. 4 Microstructures of GF/Pb composites with different GF (Pb matrix composites reinforced with GF were prepared by hot pressing at 200 °C for 2 h)
Figure 5 shows the SEM images of the Pb matrix in the Pb-1%GF composites sintered at 100, 150, 200 and 250 °C, respectively. The sintering temperature has a significant effect on the grain size of the Pb matrix, and the average grain size increases from 13.84 to 26.43 μm, as the sintering temperature rises from 100 to 250 °C. Moreover, at 200 °C and above, the individual grains grow significantly, up to 76 μm, as shown in Figs. 5(c) and (d). Such phenomenon can be attributed to the low-melting point of Pb, about 327.4 °C. It is testified that the recovery temperature and the recrystallization temperature of Pb are in the range from -130 to 100 °C and from -40 to 10 °C, respectively [15]. In the present composites, all the sintering temperatures are higher than the recrystallization temperature. As a result, some grains grow in the composites.
3.3 Tensile properties and fractography of composites
Figure 6 shows the effect of the reinforcement content and type of the GF on the tensile property of the GF/Pb composites sintered at 200 °C for 2 h. The average values of the tensile properties of the composites are summarized in Table 1. It can be seen that the tensile strength of the 50 μm-GF reinforced composites is larger than that of 300 μm-GF reinforced samples. The 50 μm-0.5%GF reinforced composite possesses the highest ultimate tensile strength (σb), which is about 15 MPa higher than that of the other GF reinforced composites in the present work and 30 MPa higher than that of pure Pb. As the better reinforcing effect can be achieved by GF with smaller sizes, the large enhancement of the σb of the 50 μm-GF composite could be mainly attributed to the significant refinement of the GF. Moreover, the fine-grain microstructure of the Pb matrix could also contribute to the enhancement of the σb. Nevertheless, the tensile strength and elongation of Pb matrix composites reinforced by the two kinds of GF show a decrease with increasing reinforcement content from 0.5% to 2%. The decreasing trend of tensile strength and strain with the reinforcement content in the GF reinforced composites can be attributed to the increase in the amount of porosity and the concentration of the GF. Generally, the discontinuity of the matrix phase increases with increasing porosities and this phenomenon causes low mechanical properties, such as tensile strength.
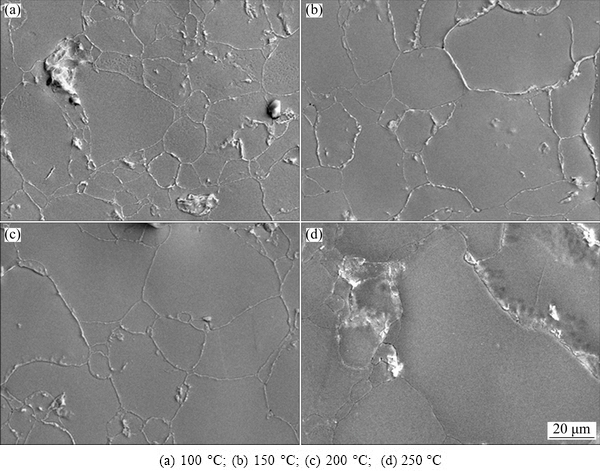
Fig. 5 SEM images of Pb matrix in Pb-1%GF composites sintered at different temperatures
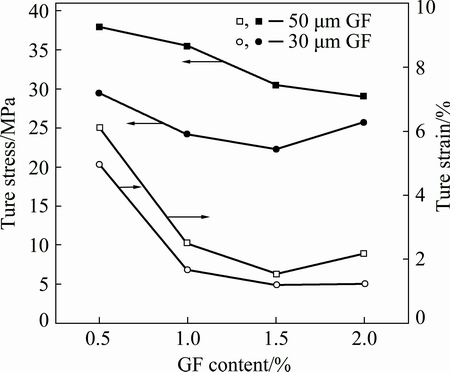
Fig. 6 Variation of tensile property in Pb-matrix composites with different types of GF content
Figure 7 shows the tensile true stress-strain curves of the composites with different contents of 50 μm-GF addition. The composites were prepared by hot pressing at 200 °C for 2 h. It can be seen that the yield strength (σ0.2) and ultimate tensile strength (σb) of the composites increase significantly, however, the fracture strain (εf) decreases, compared to that of pure Pb. Moreover, as the content of GF increases, the σb of the composites exhibits monotonous characteristics, decreasing from maximum 37.9 MPa with 0.5% GF to 24.9 MPa with 2% GF, and the εf decreases from 4.41% to 1.27%.
Table 1 Tensile property of GF/Pb composites at room temperature
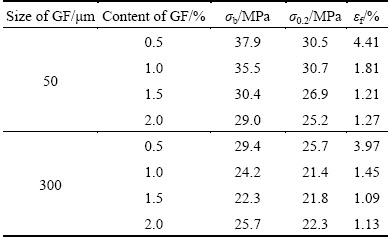
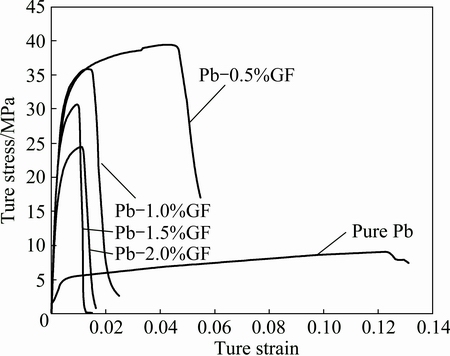
Fig. 7 True stress-true strain curves of tensile specimens having different amounts of 50 μm-GF content
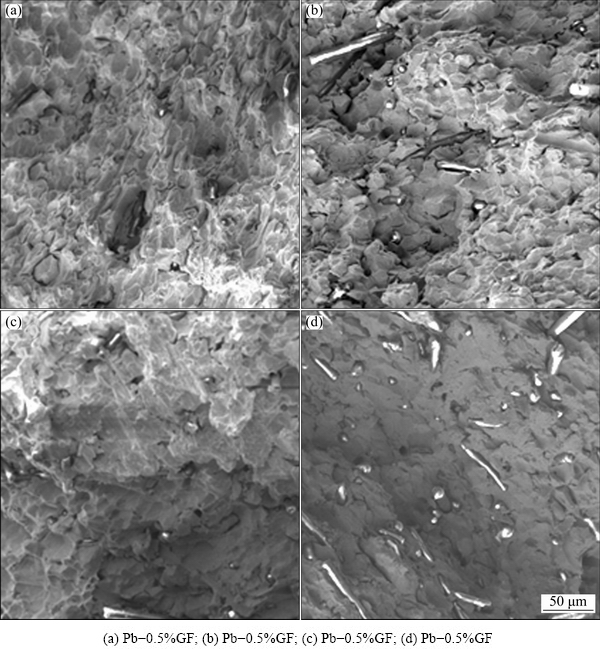
Fig. 8 SEM fractographics of GF/Pb composites
From the fracture surfaces of the composites shown in Fig. 8, the debonding between the GF particles and the Pb matrix is the main fracture mode in the composites. The poor interface between GF and Pb matrix plays the role in crack source during the deformation and facilitates the fracture of the composites. As shown in Fig. 8, the cracks between GF and Pb matrix become long and deep when the content of the additive GF increases from 0.5% to 2%.
4 Conclusions
1) The relative density decreases with increasing GF reinforcement content, and the 50 μm-GF reinforced specimens exhibit better densification than the 300 μm-GF reinforced ones, especially for the composites with high GF reinforcement fractions.
2) The average grain size increases from 13.84 μm to 26.43 μm, as the sintering temperature rises. At 150 °C and above, the individual grains grow significantly, up to 76 μm.
3) The size of the GF significantly influences the tensile properties of the composites. The composite with 50 μm-0.5%GF possesses the best tensile properties, i.e., the average tensile strength is 30 MPa higher than that of GF-free sample, but the tensile strength and elongation of GF/Pb composite show a decrease with reinforcement content from 0.5% to 2%, mainly due to increased porosities.
References
[1] ZHONG Xiao-cong, YU Xiao-ying, JIANG Liang-xing, LI Fei, LI Jie, LIU Ye-xiang. Electrochemical behavior of Pb-Ag-Nd alloy during pulse current polarization in H2SO4 solution [J]. Transactions of Nonferrous Metals Society of China, 2015, 25(5): 1692-1698.
[2] TAGUCHI M, TAKSHASHI H, NAGAI M, AICHI T, SATO R. Characteristics of Pb-based alloy prepared by powder rolling method as an insoluble anode for zinc electrowinning [J]. Hydrometallurgy, 2013, 136: 78-84.
[3] WANG Wei-guo, ZHOU Bang-xin, ROHRER G S, GUO Hong, CAI Zheng-xu. Textures and grain boundary character distributions in a cold rolled and annealed Pb-Ca based alloy [J]. Materials Science and Engineering A, 2010, 527(16-17): 3695-3706.
[4] LIU Xuan, ZHANG Jian-feng, LI Hao-yu, LE Qi-chi, ZHANG Zhi-qiang, HU Wen-yi, BAO Lei. Electrical resistivity behaviors of liquid Pb-Sn binary alloy in the presence of ultrasonic field [J]. Ultrasonics, 2015, 55: 6-9.
[5] WANG Xin, SUN Hong-fei, FANG Wen-bin. Effect of extrusion ratio on coating extrusion of Pb-GF composite wire by numerical simulation and experimental investigation [J]. Transactions of Nonferrous Metals Society of China, 2009, 19(S1): 453-457.
[6] SUN Hong-fei, WANG Er-de, FANG Wen-bin. The physical simulation on extrusion process of glass fiber composite wire [J]. Acta Metallurgica Sinica, 2004, 17(6): 940-944.
[7] ZHANG Rui, FENG Ke-qin, MENG Jun-hu, SU Bo, REN Shu-fang, HAI Wan-xiu. Synthesis and characterization of spark plasma sintered Ti3SiC2/Pb composites [J]. Ceramics International, 2015, 41(9): 10380-10386.
[8] MA Rui-xin, CHENG Shi-yao, ZHANG Xiao-yong, LI Shi-na, LIU Zi-lin, LI Xiang. Oxygen evolution and corrosion behavior of low-MnO2-content Pb-MnO2 composite anodes for metal electrowinning [J]. Hydrometallurgy, 2016, 159: 6-11.
[9] AYADI A, NOURI H, GUESSASMA S, ROGER F. An original approach to assess elastic properties of a short glass fibre reinforced thermoplastic combining X-ray tomography and finite element computation [J]. Composite Structures, 2015, 125: 277-286.
[10] MARANAN G B, MANALO A C, BENMOKRANE B, KARUNASENA W, MENDIS P. Evaluation of the flexural strength and serviceability of geopolymer concrete beams reinforced with glass-fibre-reinforced polymer (GFRP) bars [J] Engineering Structures, 2015, 101: 529-541.
[11] SUN Hong-fei, FANG Wen-bin, HAN Fei, HE Wen-xiong, WANG Er-de. Extrusion process of lead-clad glass fiber composite [J]. Transactions of Nonferrous Metals Society of China. 2003, 13(S1): s179-s182.
[12] SUN Hong-fei, FANG Wen-bin, HAN Fei, HE Wen-xiong, WANG Er-de. Fabrication of composite wire of lead coated on glass fiber by extrusion [J]. Transactions of Nonferrous Metals Society of China, 2003, 13(3): 609-612.
[13] FATHY A, EL-KADY O, MOHAMMED M M M. Effect of iron addition on microstructure, mechanical and magnetic properties of Al-matrix composite produced by powder metallurgy route [J]. Transactions of Nonferrous Metals Society of China, 2015, 25(1): 46-53.
[14] HE Wei-jun, LI Chun-hong, LUAN Bai-feng, QIU Ri-sheng, WANG Ke, LI Zhi-qiang, LIU Qing. Deformation behaviors and processing maps of CNTs/Al alloy composite fabricated by flake powder metallurgy [J]. Transactions of Nonferrous Metals Society of China, 2015, 25(11): 3578-3584.
[15] WORCESTER A W, SANKOVITCH M J. The recyclability of lead alloys [J]. Battery Conference on Application sand Advances, 1997, l: 79-83.
GF的添加对粉末冶金GF/Pb复合材料组织与拉伸性能的影响
耿耀宏1,2,王蓬瑚1
1. 东北林业大学 材料科学与工程学院,哈尔滨 150040;
2. 哈尔滨工业大学(威海) 船舶与海洋工程学院,威海 264209
摘 要:采用粉末冶金工艺制备了玻璃纤维(GF)强化的Pb基复合材料(GF/Pb),并分析了GF尺寸及含量对复合材料的密度、显微组织及拉伸力学性能的影响。结果表明,随着GF含量的增加,复合材料的相对密度降低, 50 μm-GF强化的复合材料的相对密度高于300 μm-GF强化的复合材料。低温(<200 °C时)烧结所得复合材料呈现出细小均匀的显微组织,且GF均匀分布于Pb基体中。GF的加入显著提高了Pb基复合材料的强度,添加GF尺寸为50 μm、质量分数为0.5%的Pb基复合材料比纯Pb的强度高约30 MPa。所有合成的复合材料中,随着玻璃纤维质量分数从0.5%增加到2%,复合材料的强度及拉伸应变均降低。
关键词:GF/Pb复合材料;粉末冶金;烧结显微组织;拉伸性能
(Edited by Sai-qian YUAN)
Corresponding author: Yao-hong GENG; Tel: +86-631-5687135; E-mail: yaohongg@hit.edu.cn
DOI: 10.1016/S1003-6326(16)64394-7