
Effect of Ca and Y additions on oxidation behavior of
AZ91 alloy at elevated temperatures
CHENG Su-ling(程素玲), YANG Gen-cang(杨根仓), FAN Jian-feng(樊建锋),
LI You-jie(李佑杰), ZHOU Yao-he(周尧和)
State Key Laboratory of Solidification Processing, Northwestern Polytechnical University,
Xi’an 710072, China
Received 23 October 2008; accepted 16 November 2008
Abstract: In order to develop the ignition-proof magnesium alloy, the effect of alloying elements, Ca and Y, on the oxidation behavior of AZ91 magnesium alloy at elevated temperatures was investigated. The ignition-proof performance, oxide products and oxidation kinetics of Ca- and Y-containing AZ91 alloys were studied. The results indicate that the proper addition of Ca can increase the ignition point of AZ91 alloy greatly. However, the oxide film of Ca-bearing AZ91 alloy formed at elevated temperature is thick and brittle, which is prone to crack in melting and cooling process. In addition, the oxide film of AZ91-xCa alloy is incompact and cannot inhibit the diffusion of reaction particles. The oxide film of AZ91-xCa alloy turns to thin and plastic one after Y is added, and the density of the oxide film increases greatly due to the formation of composite oxide film composed of MgO, CaO and Y2O3. ⊙
Key words: AZ91 alloy; calcium; yttrium; oxidation resistance; oxidation kinetics
1 Introduction
Magnesium alloys with low density even lighter than aluminum alloys have attracted more and more interest in automobile and electric industry. But the problem of oxidation and burning during melting and pouring process is still unsettled properly. Conventional fluxes or gases (CO2, SO2, and SF6 etc) protective methods would lead to some problems such as inclusion and environmental pollution. So, the extension application of magnesium alloys has been restricted.
The alloying ignition-proof methods, retarding the severe oxidation of magnesium alloy at elevated temperature by adding one or several active elements to promote the formation of protective oxide film, have been investigated for many years. Be and Ca were found to be effective elements to improve the oxidation resistance of magnesium alloy[1-8]. However, even a little content of Be will deteriorate the mechanical properties and the allowed content of Be is 0.01% (mass fraction) in permanent casting and 0.002% in sand casting[4, 9-10]. What’s more, Be has toxicity. Single addition of Ca cannot inhibit the ignition of magnesium alloy completely when melting and leads to the brittleness of the alloys[11]. FAN et al[12-13] reported that the addition of Y can increase the ignition point of pure Mg. But the addition dose should be larger than 10%, which will result in cost-raising. Research in Refs.[14-15] indicated that the addition of rare earth can improve the mechanical properties of Mg-Al alloys.
In the present research, the effect of single and simultaneous addition of Ca and Y on the oxidation behavior of AZ91 magnesium alloy at elevated temperature was studied. Generally, microcontent (about 0.008%) of Be is added in commercial AZ91D alloy to increase its oxidation resistance during melting and casting process. The oxidation resistance of Mg-9Al- 0.6Zn-xCa (AZ91-xCa) and Mg-9Al-0.6Zn-xCa-yY (AZ91-xCa-yY) alloys was compared with that of AZ91D alloy so as to abolish the usage of Be in the magnesium alloy in future because of its toxicity.
2 Experimental
AZ91-xCa, AZ91-xCa-yY alloys were prepared using commercial pure Mg (99.9%), Al (99.5%), Zn (99.9%), Ca (99.5%) and Mg-24.7%Y master alloy in an electric resistance furnace under the protection of CO2-0.5% (volume fraction) SF6 gases. The nominal and analyzed contents of Ca and Y in the alloys are listed in Table 1. Commercial AZ91D alloy was remelted and cast for comparative experiments under the same conditions. It should be pointed out that the analyzed compositions of Y in the alloys are deviate from the nominal value largely because Y is prone to reaction with Al to form coarse Al2Y phase, which frequently causes gravity segregation.
Table 1 Nominal and analyzed contents of Ca and Y in studied alloys (mass fraction, %)
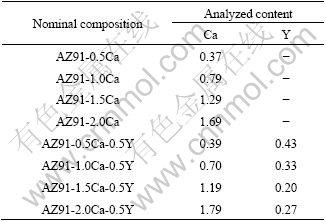
The samples to perform ignition point tests and oxide film analysis were cut to cylindrical shape with the size of d18 mm×7 mm from the central section of the ingots and polished. When testing the ignition points of the alloys, the samples were continuously heated in an electric resistance furnace exposed in air at a constant heating rate of 4 ℃/min until the ignition occurred, and the sample temperature was measured by the thermocouple and recorded. For each alloy, the measurements were carried out at least three times and the average values of ignition points were calculated. The analyses of phase compositions and microstructural morphologies were conducted by using X-ray diffractometer(XRD) and scanning electron microscope (SEM) equipped with energy dispersive spectroscope (EDS). The oxidation kinetics experiment was conducted in air at different temperatures using WRT-3P thermogravimetric analyzer (TGA), which is capable of accommodating a sample with the maximum mass of 2 g and has a measurement accuracy of 0.1 mg.
3 Results and discussion
3.1 Ignition-proof performance
A typical temperature vs time curve of ignition point testing is shown in Fig.1. The combustion heat of magnesium alloys is large enough to cause a significant rise of temperature. So, the curve would display a rapid oscillation of rising and falling in burning process and the first inflection was defined as ignition point of the sample in the present research. After all samples burned out, the temperature rising rate was restored to the setting value.
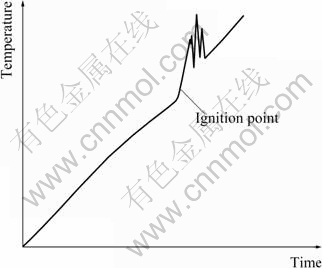
Fig.1 Typical temperature vs time curve of ignition point tests
The results of the ignition point tests are shown in Fig.2. The tested average ignition point of AZ91 alloy is 524 ℃, which is close to the result of SHIH et al[16] as 510 ℃, but larger than the result of CHOI et al[5] as 350-450 ℃ measured using a finely chipped powder specimen. The ignition point of AZ91D alloy was tested as 550 ℃, which is a little higher than that of AZ91 alloy because of Be addition. The ignition point of AZ91 alloy increases linearly with the increase of Ca content, as shown in Fig.2. The data of ignition points of Ca-bearing AZ91 alloys in Fig.2 can be fitted as a linear equation:
θ=179x+524, R=0.999 61 (1)
where θ is the ignition point (℃) and x is the analyzed content of Ca (mass fraction, %) in AZ91 alloy.
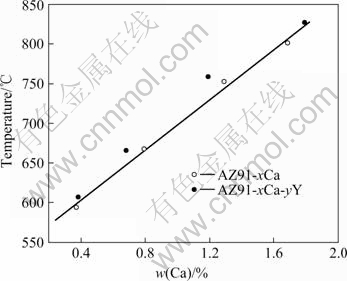
Fig.2 Ignition points of tested alloys with different contents of Ca
It is verified by further ignition point tests that the average ignition points of Ca-bearing AZ91 alloys are in agreement with Eq.(1) well when the content of Ca is lower than 1.3% (analyzed content). When the content is higher than 1.3%, the ignition temperature is unstable. However, the minimum ignition temperature is higher than 770 ℃ and some of the alloys do not burn up to 900 ℃. The testing results of ignition points of Ca-bearing AZ91 alloys imply that the proper addition of Ca is effective to improve the ignition point of AZ91 alloy up to 770 ℃, but at a higher temperature the ignition of AZ91 cannot entirely be inhibited by addition of Ca.
As can be seen in Fig.2, the ignition temperature of AZ91-xCa alloys increases generally by about 25 ℃ after about 0.5%Y is added. Further increasing of content of Y cannot improve the ignition point. By the microstructure and composition analysis[16], it was found that when Y is added into AZ91 alloy, it is prone to react with Al to form Al2Y intermetallic compound, so single-element Y is limited (less than 0.25%) in the alloy. Therefore, the ignition points are only increased by about 25 ℃ in the finite addition dose range of Y. What’s more, a large amount of Y addition will result in the formation of massive Al2Y phase, which will deteriorate the mechanical properties of the alloy. Whereas a little amount of Y will result in the formation of fine, dispersed Al2Y phase, which can increase the mechanical properties[17]. As a result, the addition of Y in AZ91 alloy should not exceed 0.25% simultaneously considering the ignition-proof performance and mechanical properties of the alloy.
3.2 Morphology and phase composition of oxide film
Fig.3 shows the surface of AZ91D, AZ91-1.5Ca and AZ91-1.5Ca-0.5Y alloys after being oxided for 100 min at 500, 700 and 700 ℃, respectively. As can be seen in Fig.3(a), a thin oxide film with black oxide product (i.e. discontinuous ignition point) in some region is formed on AZ91D sample at 500 ℃. It is obvious that it will burn if the temperature increases further. So, the ignition of AZ91D alloy occurs at solid state when being heated in air atmosphere. Fig.3(b) shows a smooth film with some fold (signed by arrow) formed on AZ91-1.5Ca alloy at 700 ℃, and the film is thick and brittle. A hole on the right bottom of the sample is a discontinuous ignition point. An uniform film with fine wrinkle without any discontinuous ignition point or crack is formed on AZ91-1.5Ca-0.5Y alloy at 700 ℃, as shown in Fig.3(c). From the shapes of AZ91-1.5Ca and AZ91-1.5Ca-0.5Y samples after oxidation at 700 ℃, it can be seen that the deforming of the former is slight whereas that of the latter is complete, resulting from the effect of gravitation during melting. So, it can be concluded that the oxide film of AZ91-xCa alloy had turn to thin and plastic one
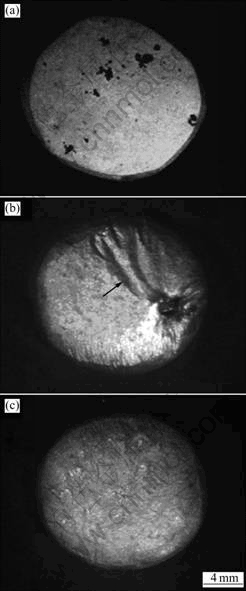
Fig.3 Surface appearances of AZ91D (a), AZ91-1.5Ca (b) and AZ91-1.5Ca-0.5Y (c) alloys held for 100 min at 500, 700 and 700 ℃, respectively
after a little content of Y was added.
XRD analysis (Fig.4) indicates that the oxide film formed on AZ91D at 500 ℃ is mainly composed of MgO, MgAl2O4 and Mg3N2. MgAl2O4 and Mg3N2 are the products of serious oxidation or ignition[18]. There are also Mg and Mg17Al12 peaks due to that the film is so thin that X-ray penetrates the film into the alloy substrate. After Ca is added, CaO is formed in the oxide film. When Y is added into AZ91-xCa alloy, Y2O3 is formed. The oxide film is mainly composed of MgO, CaO and Y2O3, no MgAl2O4 or Mg3N2 is found, which indicates that no serious oxidation or ignition occurs. In addition, intermetallic compound Al2Ca and Al2Y are detected which locate on the surface of the samples.
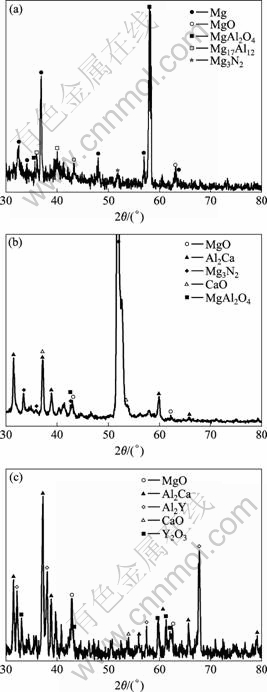
Fig.4 XRD patterns of oxide film formed on AZ91D alloy at 500 ℃ (a), AZ91-1.5Ca alloy at 700 ℃ (b) and AZ91-1.5Ca- 0.5Y alloy at 700 ℃ (c)
Fig.5 shows SEM images of oxidized surface of AZ91-1.5Ca and AZ91-1.5Ca-0.5Y alloys after oxidation at 700 ℃ for 30 min. As can be seen in Fig.5, all of the samples have an uniform oxide film, and severe oxidation occurs at grain boundary because the grain boundaries can serve as short-circuit paths for diffusion of reaction particles. The surface turns smoother and compacter after Y is added. The white oxide particles on grain boundaries in Fig.5(a) are serious oxidation products. The kind of particle is less in Fig.5(b). Energy dispersive spectroscopy analysis indicates that the contents of Ca and Y in oxide film are higher than those in matrix (by about 10 times), indicating that Ca and Y are surface active elements in AZ91 alloy. They will diffuse to the surface from the matrix continuously at elevated temperature along with the processing of reaction of Ca and Y on surface in air atmosphere.
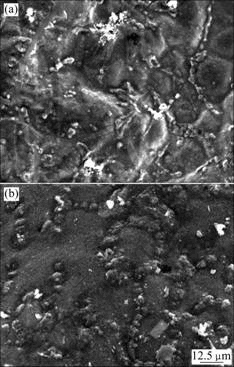
Fig.5 SEM images of oxide film formed on AZ91- 1.5Ca alloy (a) and AZ91-1.5Ca-0.5Y alloy (b) after oxide for 30 min at 700 ℃
3.3 Oxidation kinetics analysis
Fig.6 shows the oxidation dynamic curves of AZ91D, AZ91-1.5Ca and AZ91-1.5Ca-0.5Y alloys at a heating rate of 2.5 ℃/min. All the curves show three distinct stages of oxidation, namely, initial oxidation period, incubation period and non-protective oxidation period[18-19]. For AZ91D alloy, when the temperature exceeds 450 ℃, the oxidation transfers to non-protective oxidation followed by catastrophic oxidation with a significant increase of mass gain. After the addition of 1.5%Ca, the beginning temperature of the non-protective oxidation period increases to 500 ℃, and increases further to 550 ℃ for AZ91-1.5Ca-0.5Y alloy. It is obvious from Fig.6 that the mass gain of AZ91-1.5Ca alloy is less than that of AZ91D alloy and that of AZ91-1.5Ca-0.5Y alloy is the smallest.
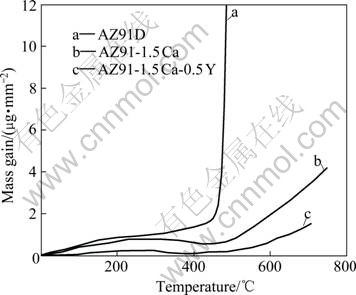
Fig.6 Oxidation dynamic curves of alloys under heating-up state
The isothermal oxidation experiments at 400 ℃ and 700 ℃ are shown in Fig.7. The mass gain of AZ91- 1.5Ca alloy is higher than that of AZ91D alloy and that of AZ91-1.5Ca-0.5Y alloy is the least at 400 ℃ (Fig.7(a)). So, after Ca is added, the oxide film formed on AZ91 alloy at elevated temperature is thick. Oxidation dynamic curves of AZ91-1.5Ca and AZ91-1.5Ca-0.5Y alloys held at 700 ℃ (Fig.7(b)) follow parabolic law and the mass gain of the latter is relatively small. The oxidation mass gain curves of AZ91-1.5Ca and AZ91- 1.5Ca-0.5Y alloys held at 700 ℃ can be fitted as follows:
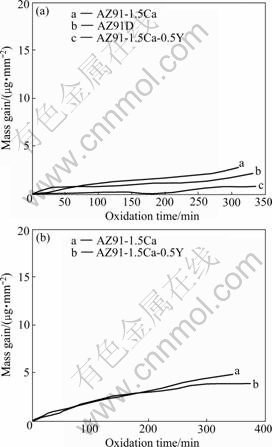
Fig.7 Oxidation dynamic curves of alloys at 400 ℃ (a) and at 700 ℃ (b)
For AZ91-1.5Ca,
y2=0.072 25t-3.406 27, R=0.983 83 (2)
For AZ91-1.5Ca-0.5Y,
y2=0.046 25t-0.911 67, R=0.986 80 (3)
where y and t are mass gain and oxidation time, respectively.
Compared Eq.(3) with Eq.(2), the addition of Y can decrease the oxidation rate of Ca-bearing AZ91 alloy effectively, indicating that the oxidation resistance can be increased by the addition of Y.
General observation of the samples of AZ91-1.5Ca and AZ91-1.5Ca-0.5Y alloys after heating at 700 ℃ for 400 min found that pulverous oxide is formed on the former, whereas the oxide formed on the later is hard and compact, confirming that the oxide film comprised by MgO and CaO is incompact and Y addition can increase the density and adhesion capacity of oxide film of Ca-bearing AZ91 alloy.
Based on the above analysis, it can be concluded that the effect of Ca on the oxidation resistance of AZ91 alloy is significant. But the mass gain of Ca-containing AZ91 alloy oxidized at elevated temperature is large, indicating that the oxide film is thick. The mass gain will be reduced significantly after Y is added. The Pilling-Bed worth(P-B) rate of Ca element is 0.65 even lower than that of Mg element (0.81)[19], so the oxide film composed of MgO and CaO will not be compact. The increasing of ignition temperature may be interpreted by the special structure of composite oxide film. When Y is added, the oxidation mass gain of AZ91-xCa-yY is lower than that of AZ91-xCa or AZ91D alloy, which indicates that a thinner film is formed. It can be interpreted that the P-B rate of Y is greater than 1 (1.39)[19], and the oxide film will be compact due to the formation of composite oxide film containing Y2O3. However, the mechanism of ignition, such as the effect of Ca and Y on the oxidation process, the structures of MgO, CaO and Y2O3 composite oxides should be further investigated.
4 Conclusions
1) The addition of Ca can increase the ignition temperature of AZ91 alloy significantly. The correlation of ignition point θ of AZ91-xCa alloy vs Ca-content x is as follows: θ=179x+524 (x<1.3). The ignition tempera- ture of AZ91-xCa alloys only increases by 25 ℃ when Y is added because the single-element Y in the alloy is limited for the reaction of Al and Y.
2) After Ca is added, the oxide film formed on AZ91 alloy at elevated temperature is thick and brittle and prone to crack during deformation. The density and plastic properties of the oxide film can be increased greatly after microcontent Y is added due to the formation of composite oxide film containing Y2O3.
References
[1] SAKAMOTO M, AKIYAMA S. Suppression of ignition and burning of molten Mg alloys by Ca bearing stable oxide film [J]. J Mater Sci Lett, 1997, 16: 1048-1050.
[2] ZENG X Q, WANG Q D, LU Y Z, DING W J, ZHU Y P, ZHAI C Q, LU C, XU X P. Behavior of surface oxidation on molten Mg-9Al-0.5Zn-0.3Be alloy [J]. Mater Sci Eng A, 2001, 301A: 154-161.
[3] YOU B S, PARK W W, CHUNG I S. The effect of calcium additions on the oxidation behavior in magnesium alloys [J]. Scripta Mater, 2000, 42: 1089-1094.
[4] ZENG X Q, WANG Q D, LU Y Z, DING W J, LU C, ZHU Y P, ZHAI C Q, XU X P. Study on ignition proof magnesium alloy with beryllium and rare earth additions [J]. Scripta Mater, 2000, 43: 403-409.
[5] CHOI B H, PARK I M, YOU B S, PARK W W. Effects of Ca and Be additions on high temperature oxidation behavior of AZ91 alloys [J]. Mater Sci Forum, 2003, 419/422: 639-644.
[6] HUANG Y B, YOU B S, CHOI B H, PARK W W, CHUNG I, SUH S K. Model for the protective oxidation of Mg-Ca alloys containing Be at elevated temperature [J]. Mater Sci Forum, 2004, 449/452: 637-641.
[7] ZHAO Y H, ZENG X Q, DING W J, WANG Q D, ZHU Y P. Effects of Be and Ca on surface oxidation behaviors of Mg-9Al-0.5Zn alloy [J]. The Chinese Journal of Nonferrous Metals, 2000, 10(6): 847-852. (in Chinese)
[8] FAN J F, YANG G C, CHENG S L, XIE H, HAO W X, WANG M, ZHOU Y H. Oxidation behavior of ignition-proof magnesium- calcium alloys [J]. The Chinese Journal of Nonferrous Metals, 2004, 14(10): 1666-1670. (in Chinese)
[9] ZENG X Q, WANG Q D, LU Y Z, ZHU Y P, DING W J, ZHAO Y H. Influence of beryllium and rare earth additions on ignition-proof magnesium alloys [J]. J Mater Proc Techn, 2001, 112: 17-23.
[10] HU Z. Casting technology and quality control of aluminum and magnesium alloy [M]. Beijing: Aerospace Industry Press, 1990.
[11] LI P J, TANG B, KANDALOVA E G. Microstructure and properties of AZ91D alloy with Ca additions [J]. Mater Lett, 2005, 59: 671-675.
[12] FAN J F, YANG G C, CHENG S L, XIE H, WANG M, ZHOU Y H. Effect of rare earths (Y, Ce) additions on the ignition points of magnesium alloys [J]. J Mater Sci, 2004, 39: 6375-6377.
[13] FAN J F, YANG G C, CHENG S L, XIE H, HAO W X, WANG M, XHOU Y H. Surface oxidation behavior of Mg-Y-Ce alloys at high temperature [J]. Metal Mater Trans A, 2005, 36: 235-239.
[14] QI Q J, LIU Y B, YANG X H. Effects of rare earths on friction and wear characteristics of magnesium alloy AZ91D [J]. Trans Nonferrous Met Soc China, 2003, 13(1): 111-115.
[15] ZHANG S C, WEI B K, CAI Q Z, WANG L S. Effect of mischmetal and yttrium on microstructures and mechanical properties of Mg-Al alloy [J]. Trans Nonferrous Met Soc China, 2003, 13(1): 83-87.
[16] SHIH T S, WANG J H, CHONG K Z. Combustion of magnesium alloys in air [J]. Mater Chem Phys, 2004, 85: 302-309.
[17] CHENG S L, YANG G C, FAN J F, LI Y J, ZHOU Y H. Effect of Ca and Y on the microstructure and mechanical properties of AZ91 alloy [J]. Rare Metal Mater Eng, 2006, 35(9): 1400-1403.
[18] CZERWINSKI F. The oxidation behaviour of an AZ91D magnesium alloy at high temperatures [J]. Acta Mater, 2002, 50: 2639-2654.
[19] LI M S. High temperature corrosion of metals [M]. Beijing: Metallurgical Industry Press, 2001.
Foundation item: Project(50271055) supported by the National Natural Science Foundation of China; Project(01G53048) supported by the Aviation Foundation of China; Project(2002699018) supported by the Doctorate Foundation of the Education Ministry of China
Corresponding author: CHENG Su-ling; Tel: +86-29-88491484; E-mail: chengsuling@163.com
DOI: 10.1016/S1003-6326(08)60268-X
(Edited by YANG Hua)