
Influence of powder characteristics on structure and properties of Ni3Al fabricated by spark plasma sintering
MENG Jie(孟 杰), JIA Cheng-chang(贾成厂), HE Qing(何 箐)
School of Materials Science and Engineering, University of Science and Technology Beijing, Beijing 100083, China
Received 20 April 2006; accepted 30 June 2006
Abstract: Three kinds of Ni and Al powder mixtures with nominal composition Ni75Al25 were employed to prepare Ni3Al alloys by spark plasma sintering(SPS) process. The raw powders include fine powder, coarse powder and mechanically-alloyed fine powder. The effects of powder characteristics and mechanical alloying on structure and properties of sintered body were investigated by scanning electron microscopy(SEM), X-ray diffraction(XRD), bending test and Vickers hardness measurements. For all mixture powders near fully dense Ni3Al alloys (relative density>99.5%) are obtained after sintering at 1 150 ℃ for 5 min under 40 MPa. However a small fraction of Ni can be reserved for alloy from coarse powders. The results reveal that grain size is correlated with particle character of raw powder. Ni3Al alloy made from mechanically-alloyed fine powder has finer and more homogenous microstructure. The hardness of all alloys is similar varying from HV470 to 490. Ni3Al alloy made from mechanically-alloyed fine powder exhibites higher bending strength (1 070 MPa) than others.
Key words: Ni3Al; powder; spark plasma sintering; mechanical alloying
1 Introduction
The ordered intermetallic compound Ni3Al is considered candidate materials for technical applications because of their high mechanical strength at elevated temperatures and their low density as compared with that of nickel based superalloys[1]. But brittleness of Ni3Al at ambient temperatures had been believed to be the most serious problem preventing its application[2,3]. Ni3Al is conventionally produced by melting followed by hot or cold working to their final dimensions. However, these processes require multiple remelting to ensure sufficient homogeneity, and have drawbacks of crucible and oxygen contamination as well as difficulty in working or mechanically machining. Powder metallurgy (PM) process can avoid the problems associated with casting, like segregation or extensive grain growth[4], and has the added advantages of precise control composition and easy realization of complex partshapes[5]. However, the difficulties for the PM process include ease of oxidation, high porosity, and low relative density. Bulk Ni3Al based alloy has been fabricated using various PM processes, such as combustion synthesis with self-propagating wave (SHS), metal injection molding, hot isostatic pressing (HIP), and hot extrusion[6-9].
Spark plasma sintering(SPS) method has been developed as a new versatile technique to rapidly sinter various materials including metals, ceramics, and composites[10,11]. In SPS method, raw powders in a graphite die are pressed uniaxially and a low voltage pulsed current is applied. Therefore, SPS enables compacted powder to be sintered under uniform heating to high density at relatively lower temperatures, and in a much shorter sintering time (typically a few minutes) compared to conventional sintering[12]. The short time consolidation is useful to maintain the grain size and control the microstructure. But effects of raw powders on microstructure and properties of Ni3Al alloys made by SPS were important due to short time sintering. The report on this problem was rarely seen.
In the present paper, Ni3Al alloys were prepared by spark plasma sintering from three kinds of Ni and Al powder mixtures. Raw Ni powders included fine powder, coarse powder. And mechanical alloying was used for modification of powders. The effects of powder characteristics and mechanical alloying on structure and property of sintered body were initially discussed.
2 Experimental
Three types of elemental powders (with nominal composition 75/25 nickel/aluminum, mole ratio) were used as raw powders. The detailed characters of powders were shown in Table 1(For convenience, the number will be used in this paper). We selected two kinds of nickel powders as raw powders, including fine and coarse powder (see Table 1, Powder A and C). Powder B was gained by mechanical alloying from powder A for 15 min with ratio of 10∶1 of ball to powder using a vibratory ball mill. The densifications of all powders were then performed using SPS 1050 (Sumitomo Coal Mining Co., Japan). The powders were loaded in a graphite die (20 mm in diameter) and were sintered in vacuum. A pressure of 40 MPa was applied. The sintering temperature was 1 150 ℃ by heating rate of 100 ℃/min with a holding time of 5 min. All of the sintered specimens were then polished to remove any surface carbon contamination.
Table 1 Characters of raw powders
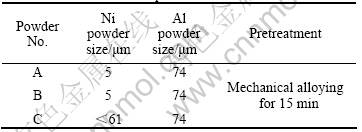
The microstructures of both the powders and sintered specimens were characterized using a scanning electron microscope (SEM). The phase identification was evaluated by X-ray diffraction (XRD) analysis using
CuKα radiation. The densities were determined by Archimedes method using water immersion. Microvickers hardness (0.98 N, 15 s) measurements were carried out. In addition, samples with sizes of 3 mm×3 mm×18 mm were cut from compacts and bended at a strain rate of 0.01 s-1 at room temperature to examine their mechanical behavior.
3 Results and discussion
3.1 Analysis of raw powders
X-ray diffraction patterns of three types of raw powders are presented in Fig.1. Ni peaks and Al peaks appear for all powders. But the grain size and strain of particles were different, the peak relative intensity is slightly discriminating. For powder B fine grain size and large strain were obtained by mechanical alloying in comparison with that of powder A. The morphologies of particles before sintering is shown in Fig.2. Spherical Ni
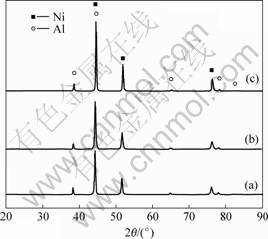
Fig.1 XRD patterns of raw powders: (a) powder A; (b) powder B; (c) powder C
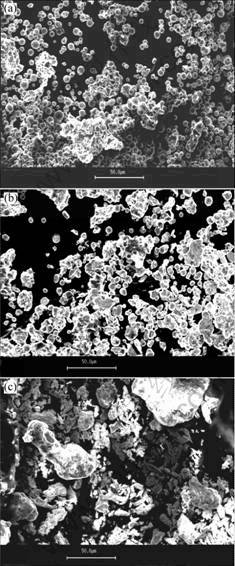
Fig.2 Morphologies of raw powders: (a) powder A; (b) powder B; (c) powder C
particles and irregular Al particles are seen in powder A (Fig.2(a)). After mechanical alloying elliptical Ni particles and flaky Al particles are formed in powder B (Fig.2(b)), for severe plastic deformation occurs by impactting between ball and ball (or ball and vial) during mechanical alloying. Irregular and coarse size particles are observed in powder C (Fig.2(c)).
3.2 Microstructure and properties of Ni3Al based alloys by SPS
Fig.3 shows the X-ray diffraction patterns of Ni3Al alloys synthesized by SPS from various raw powders. The ordered DO3 structure peak can be seen for all cases, which reveals that Ni reacts with Al to form Ni3Al during SPS. However a small fraction of Ni peak can be observed for alloy from powder C. For powder C Ni and Al powder are coarse in size, which makes it difficult to sufficiently contact between Ni powder and Al powder. In addition, the reaction needs more dynamic for powder C because it has less surface area and less energy. So the entire reaction has been realized from fine powders for powder A and B. It is suggested that Ni and Al elemental powder can react to form Ni3Al when sintering condition is enough. However coarse powder having less active energy (for powder C) will need more high sintering condition, such as increasing sintering temperature, pressure and extension of sintering time.
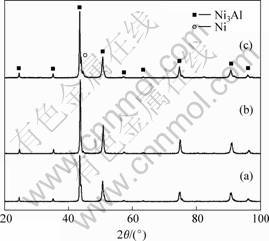
Fig.3 XRD patterns of Ni3Al alloys synthesized by SPS:(a) powder A; (b) powder B; (c) powder C
Table 2 shows the density and microhardness of alloys by SPS from various powders. The relative densities of alloys by SPS are higher than 99.5% for all cases. Therefore, the conditions of SPS we used in this paper are enough to make the powder consolidated. All samples exhibit nearly equivalent value of microhard-
ness (470-490). It is reported that Vickers hardness values for hot pressing materials with powders by vacuum gas atomization vary from 311 to 392[13], lower than those of Ni3Al in this paper.
Table 2 Density and hardness of alloys synthesized by SPS from various raw powders
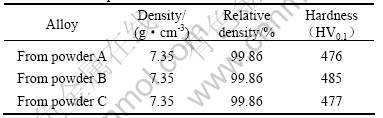
Fig.4 shows the morphologies of Ni3Al alloys prepared by SPS from various powders. It can be seen that Ni3Al from powder B has fine and homogenous microstructure (see Fig.4(b)). However Ni3Al from powder C has asymmetric grain (see Fig.4(c)) because time of SPS was so short that unequigranular particles were consolidated but not homogenized. And Ni3Al from
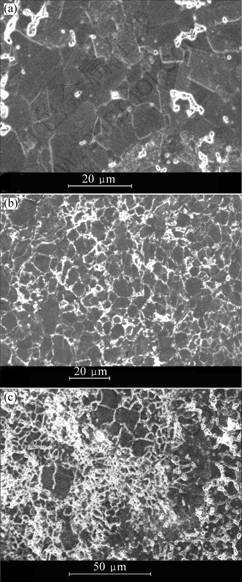
Fig.4 Morphologies of Ni3Al alloys prepared by SPS: (a) powder A; (b) powder B; (c) powder C
powder A has relative coarse grain (average size is 15 μm) (Fig.4(a)). For fine powders have more surface area energy than coarse powders, its grain growth is rapid during SPS even though raw powder is fine. While powder grain gets fine after mechanical alloying, which can be known by X-ray diffraction results (see Fig.1), so alloy from powder B has fine grain. Furthermore a large amount of drawbacks in particles formed during mechanical alloying hindered grain growth for powder B. The bending stress—strain curves of Ni3Al alloys synthesized by SPS from various powders are shown in Fig.5. All curves reveal evidently brittle fracture characteristics. Compared with others, Ni3Al prepared from powder B has highest bending strength (1 070 MPa) and large strain, as seen curve 2 in Fig.5. Property of alloy is tight correlate with its microstructure. According to analysis of Fig.4, Ni3Al from powder B has fine and homogenous grain so it has excellent combination property. Coarse grain Ni3Al from powder A has lowest strain (curve 1 in Fig.5) and irregular grain Ni3Al from powder C has lowest fracture strength (curve 3 in Fig.5). For alloy made with powder C a small fraction of Ni existed improve its ductility but decrease its fracture strength.
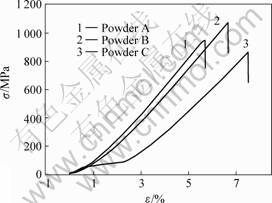
Fig.5 Bending stress—strain curves of Ni3Al alloys by SPS
Fig.6 shows the scanning electronic micrographs of the fracture surfaces of Ni3Al alloys by SPS from various powders after bending test at room temperature. Intergranular failure can be clearly seen in Fig.6(a) for the Ni3Al alloy made with powder A, not only due to the clearly shown individual grains on the fracture surface, but also many secondary grain boundary cracks. However, the fracture surface features in the Ni3Al alloy with powder B and C are different, as seen in Figs.6(b) and (c), where intergranular failure coexists with a portion of transgranular failure. The difference in the grain size of the three alloys can also be clearly seen by comparing the micrograph of Fig.6, which agrees with that of Fig.4. This result indicates that excellent microstructure by mechanical alloying improve the property of alloy. However, in all of the alloys, either by the mode of intergranular or transgranular failure, the alloy shows brittle failure, consistent with the stress—strain behavior during deformation, which can be improved by adding alloy elements (such as boron and chromium ) or other methods in the future study.
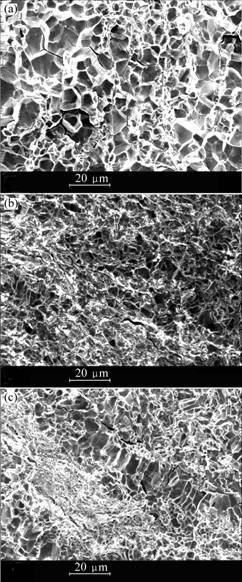
Fig.6 Micrographs of fracture surfaces of Ni3Al alloys by SPS: (a) powder A; (b) powder B; (c) powder C
4 Conclusions
1) Near fully dense Ni3Al based alloys (relative density>99.5%) were obtained by spark plasma sintering at 1 150 ℃ for 5 min under 40 MPa for all mixture powders.
2) A small fraction of Ni could be reserved for SPS alloy from coarse powders having less surface area energy. However pure Ni3Al were gained by SPS from fine powders.
3) Fine and homogenous crystalline alloys were obtained by SPS with mechanically-alloyed fine powders.
4) Alloy prepared by SPS with mechanically- alloyed fine powders exhibit highest bending strength (1070 MPa) and large strain (6.7%).
References
[1] YE H Q. Recent developments in high temperature intermetallics research in China [J]. Intermetallics, 2000, 8 (5-6): 503-509.
[2] AOKI K, ISHIKAWA K, MASUMOTO T. Ductilization of Ni3Al by alloying with boron and substitutional elements[J]. Mater Sci Eng A, 1995, 192/193: 316-323.
[3] GEORGE E P, LIU C T, LIN H. Environmental embrittlement and other causes of brittle grain boundary fracture in Ni3Al[J]. Mater Sci Eng A, 1995, 192/193(1): 277-288.
[4] PEREZ P, EDDAHBI M, GONZALEZ-CARRASCO J L. Effect of the processing route on the oxidation behaviour of a Ni3Al powder metallurgy alloy[J]. Intermetallics, 1999, 7(6): 679-87.
[5] HIBINO A, MATSUOKA S, KIUCHI M. Synthesis and sintering of Ni3Al intermetallic compound by SHS[J]. J Mater Process Tech, 2001, 112: 127-135.
[6] BOZIC D, ILIC N, MITKOV M. Comparative study of microstructure,mechanical and fracture properties of Ni3Al-based intermetallics produced by powder metallurgy and standard melting and casting processes[J]. J Mater Sci, 1996, 31(21): 3213-3221.
[7] WEGMANN M R, MISIOLEK W Z, GERMAN R M. Injection molding and reactive sintering of Ni3Al[A]. Proceedings of the 1991 Powder Metallurgy Conference and Exhibition: Advances in Powder Metallurgy[C]. Chicago: Metal Powder Industries Federation, 1991. 75-180.
[8] PEREZ P, RUIZ-CHICA J, GARCES G. Effect of chromium addition on the properties of a fine-grained PM Ni3Al alloy[J]. Mater Sci Forum, 2003, 426-432(3): 1909-1914.
[9] MINAY E J, Mcshane H B, RAWLINGS R D. The hot extrusion reaction synthesis of nickel aluminide alloys[J]. Intermetallics, 2004, 12(1): 75-84.
[10] GAO L, HONG J S, MIYAMOTO H. Bending strength and microstructure of Al2O3 ceramics densified by spark plasma sintering[J]. J Euro Ceram Soc, 2000, 20(12): 2149-2152.
[11] TAKIDA T, MABUCHI M, NAKAMURA M. Mechanical properties of a ZrC-dispersed Mo alloy processed by mechanical alloying and spark plasma sintering[J]. Mater Sci Eng A, 2000, 276(1-2): 269-272.
[12] NYGREN M, SHEN Z J. On the preparation of bio-, nano- and structural ceramics and composites by spark plasma sintering[J]. Solid State Sci, 2003, 5(1): 125-131.
[13] SHEE S K, PRADHAN S K, DE M. Effect of alloying, on the microstructure and mechanical properties of Ni3Al[J]. J Alloys Comp, 1998, 265: 249-256.
(Edited by CHEN Ai-hua)
Corresponding author: MENG Jie; Tel: +86-10-62334271; Fax:+86-10-62325983; E-mail: mjmengjie@126.com.