
Microstructure and properties of β-NiAl and its eutectic alloy with Cr and Mo additions
ZHANG Zhi-gang(张志刚), LIU Xian-bin(刘先斌), GONG Sheng-kai(宫声凯), XU Hui-bin(徐惠彬)
School of Materials Science and Engineering, Beijing University of Aeronautics and Astronautics,
Beijing 100083, China
Received 28 July 2006; accepted 15 September 2006
Abstract: NiAl-0.3Ru and NiAl-30Cr-4Mo alloys were fabricated by arc melting method and then annealed at 1 423 K for 12 h. It has been revealed that NiAl-0.3Ru was in a single-phase with large grain size and NiAl-30Cr-4Mo consisted of multiple eutectic cells. Each cell consisted of alternating plates NiAl and Cr(Mo). The compressive properties including the brittle-ductile transition temperature (BDTT) were tested. NiAl-0.3Ru and NiAl-30Cr-4Mo fractured with a little plastic deformation after yielding below the BDTT, and almost no fracture was found after large deformation up to 60% above the BDTT. Fractograph showed that at the room temperature, the fracture in NiAl-0.3Ru was intergranular and the NiAl-30Cr-4Mo in transgranular along the interface between b-NiAl and Cr (Mo). The compressive properties of NiAl were obviously improved by eutectic alloying with Mo and Cr additions.
Key words: β-nickel aluminide; eutectic alloy; compressive properties; brittleness; ductility; fracture morphology
1 Introduction
NiAl intermetallic alloys have been recognized as promising candidates for high temperature structural applications[1-5]. These alloys possess several attractive properties such as low density (5-6 g/cm3), about 2/3 of the density of nickel-based superalloys, high modulus ( 189 GPa), and excellent oxidation resistance up to
1300℃[6-10]. Furthermore, polycrystalline NiAl exhibits a brittle-to-ductile transition in the temperature range from 300 ℃ to 600 ℃ which is significantly lower than that of the other intermetallic compounds. The exact temperature depends on the stoichiometry, impurity content and grain size[11-13]. However, to make NiAl a viable structural material, it is necessary to overcome some of its inherent problems. These include low ductility and fracture toughness at ambient temperatures and inadequate strength at elevated temperatures. Accordingly, significant efforts have been centered on enhancing the mechanical properties of NiAl through micro- and macro-alloying as well as incorporating second phase reinforcements. It has been shown that small additions of Fe, Ga, Mo (0.3%, mole fraction) to NiAl can improve its mechanical properties[14-16]. On the other hand, macro alloying element addition of ductile refractory metals such as Cr, W, Mo, Re and V to brittle NiAl intermetallic matrix to improve its mechanical properties has become a focus of research[17-19]. In this paper, a single-phase alloy (NiAl-0.3Ru) and a quasi-eutectic alloy (NiAl-30Cr-4Mo) were fabricated and their microstructures, room temperature and elevated temperature compressive properties were characterized.
2 Experimental
The alloys used in this study were prepared from starting materials of 99.97 % Ni, 99.99 % Al, 99.9 % Cr, 99.9 % Mo, and 99.9% Ru(mass fraction). Button ingots, approximately 45 g each, were prepared by vacuum arc melting under an argon atmosphere using a non-consumable tungsten electrode and then remelted more than three times to ensure homogeneity. Since the arc-melted ingots had a high inner stress and an extremely fine microstructure, an annealing heat treatment at 1 200 ℃ for 12 h was carried out to relieve stress and better characterize the microstructure.
Compression specimens were taken from annealed ingots by wire electro-discharge machining (EDM). The compression specimens with d6 mm×9 mm in size were tested in the as-EDMed surface condition. The compression testing was conducted in a universal testing machine at different temperatures, and all the tests were conducted in air at constant strain rate of 9.26×10-4 s-1. The crystal structure of different alloys was identified by a D/MAX2000 X-ray diffractometer (XRD) with Cu Ka radiation. Scanning electron microscope(SEM) with EDS was used to study their microstructure, fractography and composition distribution.
3 Results and discussion
The microstructure of NiAl-0.3Ru is shown in Fig.1. It can be seen that the alloy is in single phase with large grain size. XRD analysis shows that the phase is b-NiAl and EDS analysis (Fig.2) indicates that the composition (mole fraction) of Ni, Al and Ru is about 53.25%, 46.43% and 0.33%, respectively.
Fig.3 shows the microstructure of NiAl-30Cr-4Mo. It can be seen that this alloy consists of multiple eutectic cells. The eutectic cells are enclosed by relatively thick intercellular regions. Each cell consists of alternating plates NiAl (dark contrast) and Cr alloyed with Mo (light contrast), which form a radial pattern extending from the center outward toward the cell boundary. The major difference between the cell interior and the intercellular region lies in the thickness of the lamellae, where coarser alternating layers of NiAl and Cr(Mo) exist in the intercellular regions, while much thinner lamellae are found within the cells. EDS analysis (Fig.4 and Fig.5) and XRD analysis (Fig.6) reveal that the dark contrast phase is b-NiAl with Ni, Al, Cr and Mo compositions of about 30.65%, 30.16%, 35.57% and 3.62%(mole fraction), respectively. The light one is Cr (Mo) phase with Cr, Ni, Al and Mo compositions of about 69.53%, 9.47%, 10.29%, and10.71%(mole fraction), respectively.
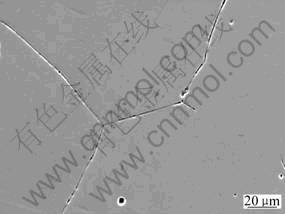
Fig.1 SEM image of NiAl-0.3Ru
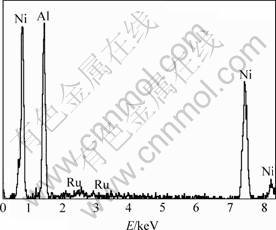
Fig.2 EDS analysis of NiAl-0.3Ru
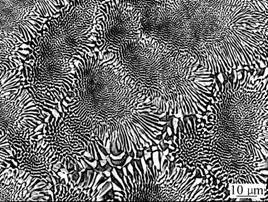
Fig.3 SEM image of NiAl-30Cr-4Mo
Fig.7(a) shows the change of compressive stress—strain curves of NiAl-0.3Ru with temperature at a strain rate of 9.26×10-4 s-1. Observed stress—strain curves can be divided into two temperature regions. At the temperatures below 673 K, the alloy fractures with a little plastic deformation after yielding, indicating that the alloy is brittle. At the temperature above 873 K, obvious plastic deformation is observed and no fracture is found after large deformation (compressive strain up to 60%), showing that the alloy is ductile at high temperatures. Thus, at the compressive strain rate of 9.26×10-4 s-1, the brittle-to-ductile transition temperature (BDTT) of NiAl-0.3Ru is between 673 K and 873 K. The change of compressive stress—strain curves with temperature at 9.26×10-4 s-1 of NiAl-30Cr-4Mo (Fig.7(b)) is similar to NiAl-0.3Ru. But the BDTT of NiAl-30Cr-4Mo is between 473 K and 673 K and the plastic strain to fracture after yielding is obviously higher than NiAl-0.3Ru.
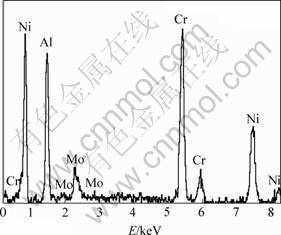
Fig.4 EDS analysis of NiAl-30Cr-4Mo (dark contrast)
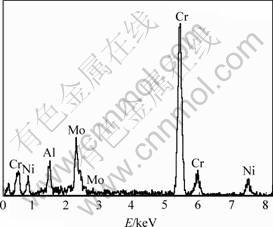
Fig.5 EDS analysis of NiAl-30Cr-4Mo (light contrast)
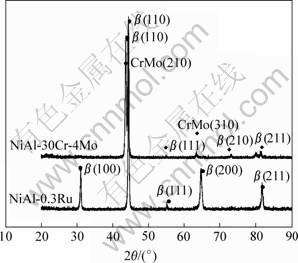
Fig.6 XRD patterns of NiAl-0.3Ru and NiAl-30Cr-4Mo
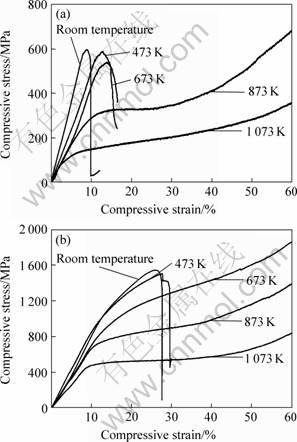
Fig.7 Compressive stress—strain curves for alloys at different temperatures: (a) NiAl-0.3Ru; (b) NiAl-30Cr-4Mo
Fig.7 shows the stress—strain curves of two alloys. Below the BDTT, significant strain-hardening can be seen after yielding, whereas slight strain-hardening is observed above BDTT, which means that the resistance to plastic deformation decreases with temperature increasing, especially above BDTT.
Temperature dependence of the compressive yield stress and compressive strain to fracture of NiAl-0.3Ru and NiAl-30Cr-4Mo are shown in Fig.8. It is seen that at room temperature, NiAl-30Cr-4Mo exhibits yield strength of 1 035 MPa and strain to fracture of 27.5%, while its counterparts in NiAl-0.3Ru are only 576 MPa and 9.8%. With temperature increasing, the strain to fracture increases and the yield strength decreases. However, at high temperatures, the compressive yield strength of NiAl-30Cr-4Mo is still several times as high as that of the NiAl-0.3Ru, indicating that the compressive properties of NiAl alloys can be improved by eutectic alloying with chromium and molybdenum addition.
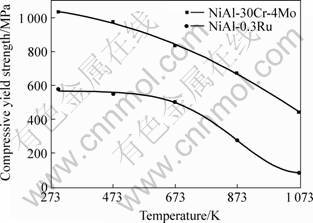
Fig.8 Temperature dependence of compressive yield stress
Besides diversity in compressive properties, a significant diversity in fracture morphology is observed in NiAl-30Cr-4Mo and NiAl-0.3Ru (see Fig.9). The fracture of NiAl-0.3Ru is intergranular (Fig.9(a)), which agrees with the previous observations. However, the eutectic alloy fractures transgranularly. Its fracture surface gives a layered tearing and cleavage appearance (Fig.9(b)) as opposed to the sharp faceted appearance in the NiAl-0.3Ru, as a result of the grain boundary decohesion. Moreover, the compressive fracture behavior of the eutectic alloy indicates predominant debonding along the b/Cr(Mo) interface at room temperature.
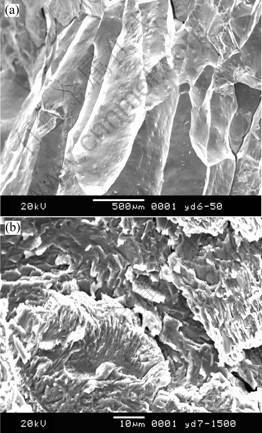
Fig.9 Fractographs of alloys tested in compression at room temperature: (a) NiAl-0.3Ru; (b) NiAl-30Cr-4Mo
4 Conclusions
1) The microstructure of NiAl-0.3Ru is in single phase with large grain size. But NiAl-30Cr-4Mo consists of multiple eutectic cells and each cell consists of alternating plates NiAl and Cr alloyed with Mo.
2) Below the BDTT of the alloys, NiAl-0.3Ru and NiAl-30Cr-4Mo fracture with a little plastic deformation after yielding, showing that the alloy is brittle in the low temperature region. Above the BDTT, obvious plastic deformation is observed while no fracture is found after large deformation (compressive strain up to 60%), showing that the alloy is ductile.
3) At room temperature, the fracture in NiAl-0.3Ru is intergranular. But the NiAl-30Cr-4Mo fractures transgranularly. A layered tearing and cleavage appearance of fracture surface indicates debonding along the b/Cr (Mo) interface.
4) The compressive properties of NiAl-30Cr-4Mo are obviously higher than those of NiAl-0.3Ru. At room temperature, the NiAl-30Cr-4Mo exhibits yield strength of 1 035 MPa and strain to fracture of 27.5 %, indicating that the compressive properties of NiAl alloys can be improved by eutectic alloying with chromium and molybdenum additions.
References
[1] ALAIN L. Intermetallics: Why is it so difficult to introduce them in gas turbine engines? [J]. Intermetallics, 2006, 14(10-11): 1123-1129.
[2] VJUNITSKY I, SCHONFELD E, KAISER T, STEURER W, SHKLOVER V. Study of phase states and oxidation of B2-structure based Al-Ni-Ru-M alloys[J]. Intermetallics, 2005, 13(1): 35-45.
[3] CAMMAROTA G P, CASAGRANDE A. Effect of ternary additions of iron on microstructure and microhardness of the intermetallic NiAl in reactive sintering[J]. J Alloys Compd, 2004, 381(1-2): 208-214.
[4] SRDJAN M, RUBENS C. Growth morphology of the NiAl-V in situ composites[J]. J Mater Processing Tech, 2003, 143-144: 629-635.
[5] SCHEPPE F, SAHM P R, HERMANN W, PAUL U, PREUHS J. Nickel aluminides: a step toward industrial application[J]. Materials Science and Engineering A, 2002, 329-331: 596-601.
[6] SSUTHOFF G. Multiphase intermetallic alloys for structural applications[J]. Intermetallics, 2000, 8(9-11): 1101-1109.
[7] LI Hui-tian, GUO Jian-ting, HUAI Kai-wen, YE Heng-qiang. Microstructure characterization and room temperature deformation of a rapidly solidified NiAl-based eutectic alloy containing trace Dy [J]. J Crystal Growth, 2006, 290(1): 258-265.
[8] GAO Q, GUO J T, HUAI K W, ZHANG J S. The microstructure and compressive properties of as-cast NiAl-28Cr-5.8Mo-0.2Hf containing minor Dy [J]. Mater Lett, 2005, 59(23): 2859-2862.
[9] WANG Y, LIU Z, CHEN L Q. Thermodynamic properties of Al, Ni, NiAl and Ni3Al from first-principles calculations[J]. Acta Mater, 2004, 52(9): 2665-2671.
[10] ZHANG Guang-ye, HUANG Hua, GUO Jian-ting, YE Heng-qiang. Oxidation Behavior of NiAl-30.75Cr-3Mo-0.25Ho alloy at high temperature[J]. J Rare Earth, 2006, 24(1): 97-102.
[11] CHEN R S, GUO J T, ZHOU W L, ZHOU J Y. Brittle-to-ductile transition of a multiphase intermetallic alloy based on NiAl[J]. Intermetallics, 2000, 8(5-6): 663-667.
[12] CUI C Y, CHEN Y X, GUO J T, QI H Y, YE H Q. Brittle-to-ductile transition in multiphase NiAl alloy[J]. Materials Science and Engineering A, 2002, 325(1-2): 186-193.
[13] GUO J T, QI Y H, LI G S, WU W T. Tensile properties and microstructure of in situ NiAl-Cr(Zr) eutectic composite[J]. Composite Structure, 2003, 62(3-4): 323-327.
[14] ALIBTER A, SALAZAR M, BEDOLLA E, DREW R A L, PEREZ R. Improvement of the mechanical properties in a nanocrystalline NiAl intermetallic alloy with Fe, Ga and Mo additions[J]. Materials Science and Engineering A, 2003, 347(1-2): 154-164.
[15] TOMASZ C, STANISLAW W. Structure and mechanical properties of NiAl and Ni3Al-based alloys[J]. International Journal of Mechanical Sciences, 2000, 42(8): 1499-1518.
[16] FROMMEYER G, FISCHER R, DEGES J, RABLBAUER R, SCHNEIDER A. APFIM investigations on site occupancies of the ternary alloying elements Cr, Fe, and Re in NiAl[J]. Ultramicroscopy, 2004, 101(2-4): 139-148.
[17] LAPIN J. Effect of directional solidification and heat treatments on the microstructure and mechanical properties of multiphase intermetallic Zr-doped Ni-Al-Cr-Ta-Mo alloy[J]. Intermetallics, 2006, 14(12): 1417-1427.
[18] LI Hui-tian, GUO Jian-ting, HUAI Kai-wen, YE Heng-qiang. Microstructure characteristics and thermal stability of NiAl-based near-eutectic alloy fabricated by water-cooled copper mold method[J]. Materials Characterization, 2006, 14: 269-275.
[19] REN W L, GUO J T, LI G S, WU J S. On high creep activation energy for cast NiAl-9Mo eutectic alloy[J]. Materials Letters, 2005, 59(12): 1533-1537.
(Edited by PENG Chao-qun)
Foundation item: Project(50571005) supported by the National Natural Science Foundation of China
Corresponding author: ZHANG Zhi-gang; Tel: +86-10-82338173; E-mail: zhangzhigang@mse.buaa.edu.cn