Trans. Nonferrous Met. Soc. China 28(2018) 1626-1631
Washing effect on properties of LiNi0.8Co0.15Al0.05O2 cathode material by ethanol solvent
Wan-min LIU1, Mu-lan QIN1, Lü XU1, Su YI1, Ji-yong DENG1, Zhong-hua HUANG2
1. School of Chemistry and Chemical Engineering, Hunan Institute of Engineering, Xiangtan 411104, China;
2. School of Mechanical Engineering, Hunan Institute of Engineering, Xiangtan 411104, China
Received 7 March 2017; accepted 10 October 2017
Abstract: Different LiNi0.8Co0.15Al0.05O2 cathode materials were washed by ethanol solvent. Inductively coupled plasma atomic emission spectroscopy (ICP-AES), Fourier transformed infrared (FTIR) spectrum, X-ray diffraction (XRD), scanning electron microscopy (SEM), charge-discharge test and electrochemical impedance spectroscopy (EIS) were used to evaluate the elemental contents, structures, morphologies and electrochemical properties of samples. The results show that ethanol washing can remove effectively the synthetic residues LiOH/Li2O on the freshly-prepared LiNi0.8Co0.15Al0.05O2 and make the sample much more resistant to H2O and CO2, without destroying its bulk structure, surface morphology and electrochemical performances. Moreover, the discharge specific capacity and cycle performance of LiNi0.8Co0.15Al0.05O2 after storage in air with a relative humidity of 80% for three months are improved by immediate ethanol washing.
Key words: lithium-ion battery; LiNi0.8Co0.15Al0.05O2; ethanol washing; storage property; electrochemical performance
1 Introduction
Layered Ni-rich materials LiNi1-x-yCoxMyO2 (0.8≤1-x-y<1, M=Al, Mn, Mg, etc) have attracted much attention for power sources due to their large capacity, excellent rate capability and low cost [1-5]. However, their storage property has been considered to be very poor on account of the formation of Li2CO3 on the surface [6-9]. Li2CO3 is electrochemically inactive and easily causes gas evolution during the battery operation [6].
It is not denied that lithium ions in Li2CO3 derive from the as-prepared materials. During the preparation process of Ni-rich materials, excess LiOH·H2O or other lithium compounds were added. Unreacted lithium compounds present in the form of LiOH/Li2O on the fresh powder account for the very rapid appearance of Li2CO3 on the surface of the fresh material exposed to air. Thus, two approaches have been used to remove the lithium residues on the particle surface. One is to coat the material with oxides or phosphates which can react with lithium residues to form new compounds, such as LiVO2/LiV2O5 [10,11], Li2O-2B2O3 [12], LixCoPO4 [13], LiMnPO4 [14], LiCoO2 [15], FeF3 [16] and Li2TiO3 [17]. The storage performance and electrochemical properties of LiNi1-x-yCoxMyO2 materials can be enhanced due to the elimination of LiOH/Li2CO3 and the formation of the lithiated compounds on its surface. The other is to wash the material by water to remove LiOH/Li2CO3 owing to its solubility in water [7,8,18]. However, this method needs subsequent heat treatment to recover the electrochemical properties of the material because the washing process can create more NiO-like cubic phase [7].
In this work, a facile process is reported for the elimination of lithium residues based on the different solubility characteristics of LiOH and Li2CO3 in ethanol solvent, which is expected to enhance the storage performance of LiNi0.8Co0.15Al0.05O2 without destroying its bulk structure and surface morphology.
2 Experimental
LiNi0.8Co0.15Al0.05O2 was synthesized from Ni0.8Co0.15Al0.05OOH according to our previous report [1].
The freshly-prepared powders were marked as “F” and used for further experiments. At 25 °C, 20 g F-powders were dispersed in 50 mL ethanol under constant stirring with a magnetic stirrer for 15 min. The powders recovered by filtration were dried at 150 °C. This procedure was repeated 1-3 times. Correspondingly, the powders treated with ethanol 1-3 times were marked as “EFn” (n=1, 2 or 3), respectively. In addition, 20 g LiNi0.8Co0.15Al0.05O2 powders placed in air for 1 d underwent the same treatment as the freshly-prepared powders. And the obtained samples were marked as “ESn” (n=1, 2 or 3), respectively. According to our previous investigation [15], the condition of 80% relative humidity was the most terrible for the storage property of LiNi0.8Co0.15Al0.05O2, which led to the rapid decrease of the discharge capacity of the material. Therefore, in this work, the storage process was carried out at room temperature by storing the sample in air with a relative humidity of 80% for three months. The mass increasing rate (Δm) and average nickel oxidation state (OS) of the sample were calculated according to our previous report [15].
The contents of lithium, nickel, cobalt and aluminum of the samples were measured by inductively coupled plasma atomic emission spectroscopy (ICP-AES, Optima 4300DV). The moisture content (OH-) was determined by a Karl-Fisher moisture titrator at 250 °C. The carbon content in Li2CO3 was measured by a CHS element analyzer at 1000 °C in an oxygen atmosphere. Fourier transformed infrared (FTIR) spectrum was tested on Shimadzu-FTIR-8400 spectrometer. The morphology of the sample was observed by a scanning electron microscopy (SEM, SU 3500). The structure of the sample was characterized by a powder X-ray diffraction (XRD, D/max-r A type, Cu Kα).
CR2032 coin-type cells were assembled with metallic lithium anodes for electrochemical tests. The positive electrode consisted of 80% active material, 10% acetylene black and 10% PVDF binder (mass fraction). The cells were assembled in an argon filled glove box. The charge-discharge measurement was performed at a current density of 0.2C within the voltage range of 2.8-4.3 V (vs Li/Li+) at room temperature on a LAND CT2001A test system. Electrochemical impedance spectroscopy (EIS) experiments were performed at full discharge state of desired cycle using a CHI660B electrochemical workstation over the frequency range of 1×105-1×10-3 Hz with the amplitude of ±5 mV.
3 Results and discussion
3.1 Changes of chemical composition, oxidation state, moisture and carbon contents
Table 1 gives the chemical composition of LiNi0.8Co0.15Al0.05O2 before and after being washed in ethanol solvent. It is obvious that the lithium content of sample F is the highest owing to the addition of excess LiOH·H2O in its preparation. Once sample F is washed in ethanol solvent for 1-3 times, the contents of lithium, nickel, cobalt and aluminum are close to theoretical values, as shown in sample EF1, EF2 and EF3. However, after sample F placed in air for 1 d undergoes the same treatment, the contents of all elements almost does not change, as shown in sample ES1, ES2 and ES3. This may be attributed to the terrible storage condition of 80% relative humidity in air, which leads to the rapid reaction between residual LiOH/Li2O and CO2 to form Li2CO3 [7]. It is well-known that LiOH can be dissolved by appropriate amount of ethanol, while Li2CO3 is insoluble in ethanol. Therefore, ethanol washing is only used for the freshly-prepared LiNi0.8Co0.15Al0.05O2.
Table 1 Chemical compositions of samples F, EFn and ESn (n=1, 2 or 3), nickel oxidation state (OS), moisture and carbon contents in samples F, EF2 and ES2 before and after storage in air for three months
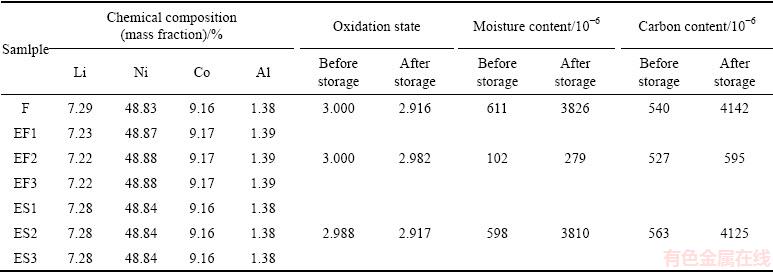
Table 1 also presents the nickel oxidation state (OS), moisture and carbon content in sample F, EF2 and ES2 before and after storage in air for three months. Before storage, OS values of sample F and EF2 are 3.000 and the carbon contents are also close, while the moisture of sample EF2 is only one-sixth that of sample F, which indicates that ethonal washing can remove effectively residual LiOH on the fresh LiNi0.8Co0.15Al0.05O2 without structural degradation. After storage, for sample F, OS decreases to 2.916, and moisture and carbon contents increase by about 6 and 7 times, respectively. However, sample EF2 shows a few changes. On the other hand, compared with sample F, sample ES2 shows the similar changes in the aspects of OS, moisture and carbon contents. These results indicate that during the course of storage, a certain amount of LiOH, Li2CO3 and NiO are formed on the surfaces of the fresh and placed powders. However, the removal of residues on the surface of the freshly-prepared powder can effectively reduce the forming amounts of LiOH, Li2CO3 and NiO. This may also show that the synthetic residue is the main source of deteriorating the storage property of LiNi0.8Co0.15Al0.05O2 and should be eliminated in time.
3.2 SEM and XRD studies
Figure 1 depicts the SEM images of samples F, EF2 and ES2. It is obvious that the surface morphology of LiNi0.8Co0.15Al0.05O2 is not affected by ethanol washing. Samples F, EF2 and ES2 all have spherical morphology in secondary particles and the estimated particle size is about 10 μm in diameter, while the primary particles are cylinder-shaped and they are densely agglomerated in secondary forms.
XRD patterns of samples F, EF2 and ES2 are shown in Fig. 2. It is observed that the three samples fit well with α-NaFeO2 structure with no minor phases [1]. Distinct splitting of the (006)/(102) and (108)/(110) peaks is seen in these patterns. The obtained lattice parameters are presented in Fig. 2. Compared with sample F, the lattice parameters of the EF2 and ES2 show no obvious changes. This indicates that the samples possess typical layered characteristics before and after ethanol washing, and the washing process does not change the structure of LiNi0.8Co0.15Al0.05O2.
3.3 Change of mass
Figure 3 shows the mass increasing rate curves of samples F, EF2 and ES2 during the course of storage. In the first 10 d, the masses of three samples all show increasing trend. However, the mass of sample F and ES2 increases more rapidly than that of sample EF2. After two weeks, the masses of samples F, EF2 and ES2 all stabilize and increase by 1.433%, 0.479% and 1.329%, respectively. These results indicate that ethanol washing can effectively enhance the storage property of fresh LiNi0.8Co0.15Al0.05O2, but it is useless for the stale powders.
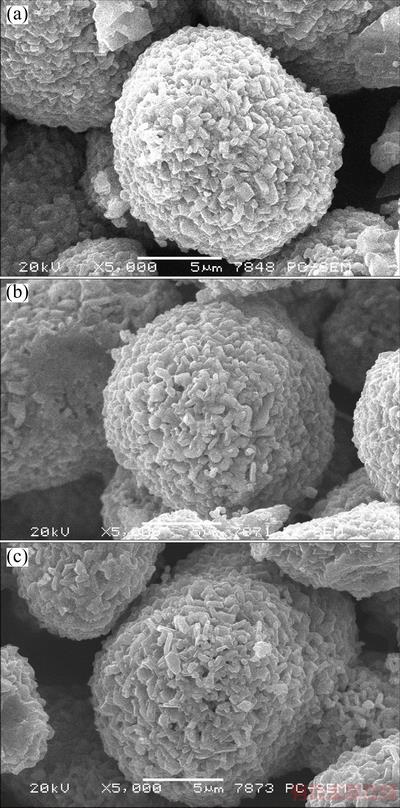
Fig. 1 SEM images of samples F (a), EF2 (b) and ES2 (c)
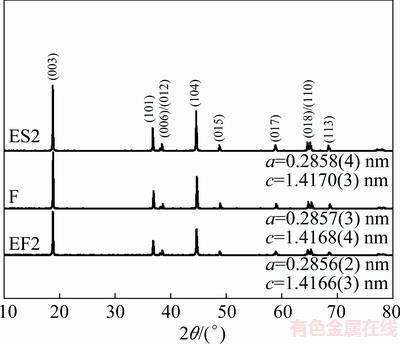
Fig. 2 XRD patterns of samples F, EF2 and ES2
3.4 FTIR study
Figure 4 shows FTIR spectra of fresh sample F, stored samples F, EF2 and ES2. Compared with fresh sample F, the FTIR spectra of the stored samples show significantly increased peaks of LiOH and Li2CO3. The band at 3600-3000 cm-1 is assigned to the O—H stretching vibration from LiOH, and two peaks at 868 and 1430 cm-1 are assigned to the
vibration from Li2CO3 [15]. The peaks of these bands for the stored sample F and stored ES2 are more prominent than those of the stored sample EF2. This means that the amounts of LiOH and Li2CO3 on the surface of sample EF2 are less than those of sample F and ES2 after exposure to air. Therefore, it is proven again that the removal of residual LiOH/Li2O with ethanol washing can effectively reduce the forming amounts of LiOH/Li2CO3 on the surface of LiNi0.8Co0.15Al0.05O2 during the storage.
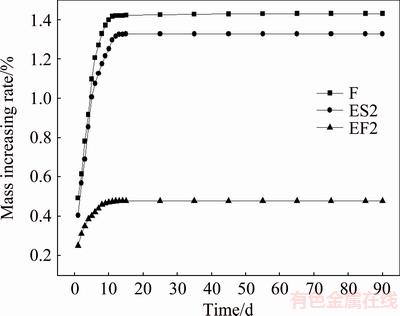
Fig. 3 Mass increasing rate curves of samples F, EF2 and ES2
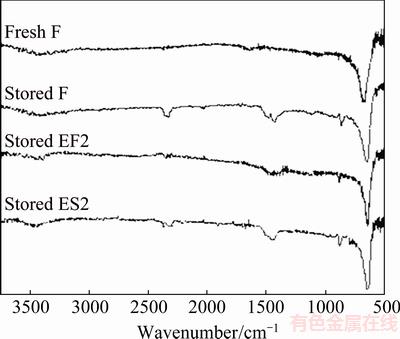
Fig. 4 FTIR spectra of fresh sample F, stored samples F, EF2 and ES2
3.5 Analysis of discharge capacity
Figure 5 compares the first and thirtieth discharge curves of sample F, EF2 and ES2 before and after storage in air for 90 d. As shown in Fig. 5, for the freshly- synthesized sample F, the first and thirtieth discharge specific capacities are 196.8 and 190.9 mA·h/g, respectively, with the capacity retention of 97.0% after 30 cycles. However, after storage in air for 90 d, its first, thirtieth discharge specific capacities and capacity retention decrease to 127.5 mA·h/g, 84.8 mA·h/g and 66.5%, respectively. Contrastively, it is found from Fig. 5 that the fresh sample EF2 has almost the same electrochemical properties as the fresh sample F, but the stored sample EF2 has higher discharge capacity and better cycle performance than the stored sample F. After storage for 90 d, the stored sample EF2 has still the initial discharge capacity of 158.6 mA·h/g and the capacity retention of 82.1% after 30 cycles. On the other hand, as shown in Fig. 5, the fresh sample ES2 displays inferior electrochemical performance to the fresh sample F and EF2. Its initial discharge capacity and capacity retention after 30 cycles are 185.5 mA·h/g and 90.5%, respectively. In addition, compared with the stored sample F, electrochemical properties of the stored sample ES2 are not improved at all.
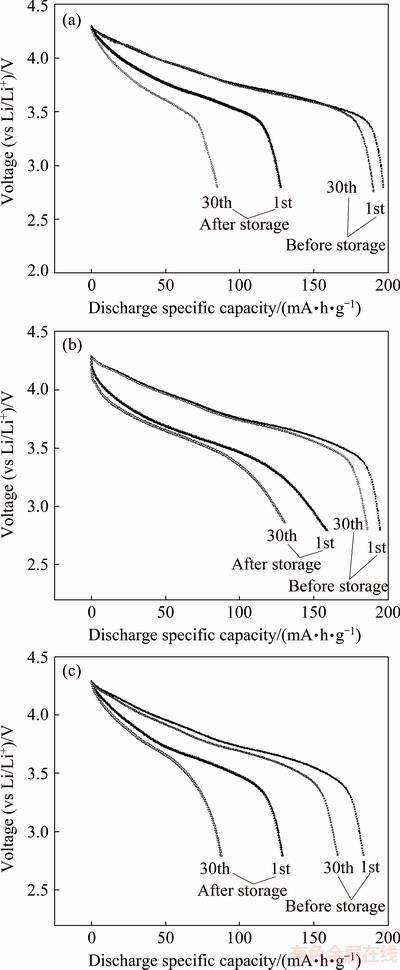
Fig. 5 First and thirtieth discharge curves of samples F (a), EF2 (b) and ES2 (c) before and after storage in air for three months
3.6 EIS analysis
In order to elucidate the effect of ethanol washing on the electrochemical properties of the material, EIS experiments were performed for samples F, EF2 and ES2 before and after storage in air with a relative humidity of 80% for three months. The impedance spectra of samples are represented in Fig. 6.
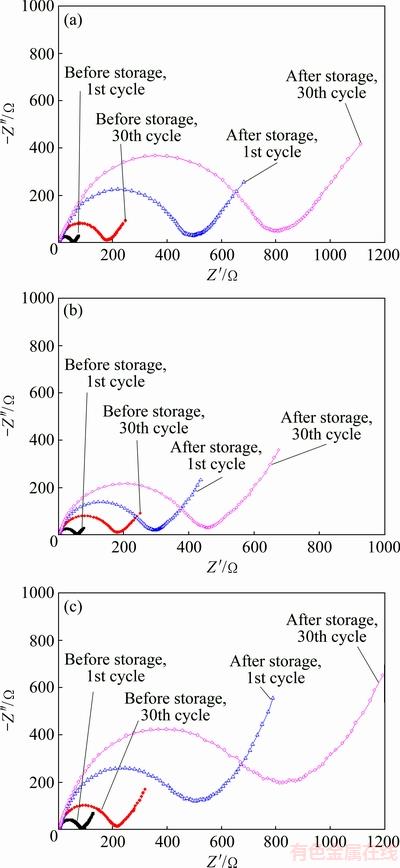
Fig. 6 EIS plots of samples F (a), EF2 (b) and ES2 (c) before and after storage in air for three months
Usually, an intercept in the high frequency region of the Z′ real axis corresponds to the ohmic resistance (Rs), which represents the resistance of the electrolyte and electrode material. The semicircle in the middle frequency range indicates the charge transfer resistance (Rct). The inclined line in the low frequency represents the Warburg impedance (Zw), which is associated with lithium-ion diffusion in the material particles [19,20]. As shown in Fig. 6, compared with Rct values, Rs values may be nearly neglected. Thus, Rct values can be used to evaluate the electrochemical performance of the active material. Table 2 lists the Rct values of samples after cycling for different times based on the EIS plots. For the sample F before storage, when the cycling times is increased from 1 to 30, its Rct value increases from 55 to 171 Ω, equal to the increment rate of 3.87 Ω per cycle. However, after the sample F is stored in air with a relative humidity of 80% for three months, its Rct value increases rapidly to 478 Ω after the 1st cycle. And the Rct value of 30th cycle is 792 Ω, with the increment rate of 10.47 Ω per cycle. This indicates that the stored sample F suffers more resistance during the course of charge-discharge, which may be attributed to the formation of a large number of electrochemically inactive materials, including NiO-like species and absorbed hydroxyl and carbonate species (shown in Table 1) [7,15]. On the other hand, the fresh sample EF2 shows the similar Rct values with the fresh sample F after charging/discharging for 1 cycle and 30 cycles. However, after the sample EF2 is stored for three months, its Rct value increases only to 292 Ω after the 1st cycle. And the Rct value increases by 159 Ω after the 30th cycle, with the increment rate of 5.3 Ω per cycle, nearly half of the corresponding value of the stored sample F. The smaller Rct is in favor of rapid Li+ diffusion and may result in better electrochemical performance of the active material (shown in Fig. 5). These data also suggest that the sample EF2 has better storage performance than the sample F, which has to ascribe to the removal of residual LiOH/Li2O with ethanol washing. As far as the sample ES2 is concerned, its Rct values before and after storage display the similar increasing tendency to the sample F.
Table 2 Rct values of samples F, EF2 and ES2 after different cycles
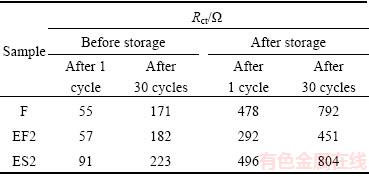
4 Conclusions
1) Ethanol washing can remove effectively the synthetic residues of LiOH/Li2O on the freshly- synthesized LiNi0.8Co0.15Al0.05O2 and make the powders much more resistant to H2O and CO2, without showing any bulk structural degradation and weakening the electrochemical performance.
2) The discharge specific capacity and cycle life of LiNi0.8Co0.15Al0.05O2 after storage in air with a relative humidity of 80% are improved by ethanol washing.
3) Ethanol washing without subsequent heat treatment is only used for the freshly-synthesized LiNi0.8Co0.15Al0.05O2, which is different from water washing. And this method is also effective for other nickel-rich cathode materials.
References
[1] HU Guo-rong, LIU Wan-min, PENG Zhong-dong, DU Ke, CAO Yan-bing. Synthesis and electrochemical properties of LiNi0.8Co0.15Al0.05O2 prepared from the precursor Ni0.8Co0.15Al0.05OOH [J]. Journal of Power Sources, 2012, 198: 258-263.
[2] XIE Hong-bin, DU Ke, HU Guo-rong, DUAN Jian-guo, PENG Zhong-dong, ZHANG Zhi-jiang, CAO Yan-bing. Synthesis of LiNi0.8Co0.15Al0.05O2 with 5-sulfosalicylic acid as a chelating agent and its electrochemical properties [J]. Journal of Material Chemistry A, 2015, 3: 20236-20243.
[3] SHIM J H, KIM C Y, CHO S W, MISSIUL A, KIM J K, AHN Y J, LEE S. Effects of heat-treatment atmosphere on electrochemical performances of Ni-rich mixed-metal oxide (LiNi0.80Co0.15Mn0.05O2) as a cathode material for lithium ion battery [J]. Electrochimica Acta, 2014, 138: 15-21.
[4] XIAO Zheng-wei, ZHANG Ying-jie, WANG Yi-fan. Synthesis of high-capacity LiNi0.8Co0.1Mn0.1O2 cathode by transition metal acetates [J]. Transactions of Nonferrous Metals Society of China, 2015, 25: 1568-1574.
[5] DENG Long-zheng, WU Feng, GAO Xu-guang, LIU Zhen-tian, XIE Hai-ming. Synthesis and electrochemical properties of LiNi0.87Co0.10Mg0.03O2 cathode materials [J]. Transactions of Nonferrous Metals Society of China, 2015, 25: 527-532.
[6] ZHUANG G V, CHEN G, SHIM J, SONG X, ROSS P N, RICHARDSON T J. Li2CO3in LiNi0.8Co0.15Al0.05O2cathodes and its effects on capacity and power [J]. Journal of Power Sources, 2004, 134: 293-297.
[7] LIU Han-san, YANG Yong, ZHANG Jiu-jun. Investigation and improvement on the storage property of LiNi0.8Co0.2O2as a cathode material for lithium-ion batteries [J]. Journal of Power Sources, 2006, 162: 644-650.
[8] KIM J, HONG Y, RYU K S, KIM M G, CHO J. Washing effect of a LiNi0.83Co0.15Al0.02O2 cathode in water [J]. Electrochemical and Solid-State Letters, 2006, 9(1): A19-A23.
[9] SHIZUKA K, KIYOHARA C, SHIMA K, TAKEDA Y. Effect of CO2on layered Li1+zNi1-x-yCoxMyO2(M=Al, Mn) cathode materials for lithium ion batteries [J]. Journal of Power Sources, 2007, 166: 233-238.
[10] LEE M J, NOH M, PARK M, JO M, KIM H, NAM H, CHO J. The role of nanoscale-range vanadium treatment in LiNi0.8Co0.15Al0.05O2 cathode materials for Li-ion batteries at elevated temperatures [J]. Journal of Materials Chemistry A, 2015, 3: 13453-13460._nf394950e0aee64bf4950f6c04152ecbf6
[11] XIONG Xun-hui, WANG Zhi-xing, YAN Guo-chun, GUO Hua-jun, LI Xin-hai. Role of V2O5coating on LiNiO2-based materials for lithium ion battery [J]. Journal of Power Sources, 2014, 245: 183-193.
[12] LIM S N, AHN W, YEON S H, PARK S B. Enhanced elevated- temperature performance of Li(Ni0.8Co0.15Al0.05)O2electrodes coated with Li2O-2B2O3glass [J]. Electrochimica Acta, 2014, 136: 1-9.
[13] KIM Y, CHO J. Lithium-reactive Co3(PO4)2 nanoparticle coating on high-capacity LiNi0.8Co0.16Al0.04O2 cathode material for lithium rechargeable batteries [J]. Journal of the Electrochemical Society, 2007, 154(6): A495-A499.
[14] DUAN Jian-guo, WU Ceng, CAO Yan-bing, DU Ke, PENG Zhong-dong, HU Guo-rong. Enhanced electrochemical performance and thermal stability of LiNi0.80Co0.15Al0.05O2 via nano-sized LiMnPO4 coating [J]. Electrochimica Acta, 2016, 221: 14-22.
[15] LIU Wan-min, HU Guo-rong, DU Ke, PENG Zhong-dong, CAO Yan-bing. Enhanced storage property of LiNi0.8Co0.15Al0.05O2 coated with LiCoO2 [J]. Journal of Power Sources, 2013, 230: 201-206.
[16] LIU Wan-min, TANG Xian, QIN Mu-lan, LI Gui-lin, DENG Ji-yong, HUANG Xian-wei. FeF3-coated LiNi0.8Co0.15Al0.05O2 cathode materials with improved electrochemical properties [J]. Materials Letters, 2016, 185: 96-99.
[17] WU Nai-teng, WU Hao, LIU Heng, ZHANG Yun. Solvothermal coating LiNi0.8Co0.15Al0.05O2microspheres with nanoscale Li2TiO3 shell for long lifespan Li-ion battery cathode materials [J]. Journal of Alloys and Compounds, 2016, 665: 48-56.
[18] XIONG Xun-hui, WANG Zhi-xing, YUE Peng, GUO Hua-jun, WU Fei-xiang, WANG Jie-xi, LI Xin-hai. Washing effects on electrochemical performance and storage characteristics of LiNi0.8Co0.1Mn0.1O2as cathode material for lithium-ion batteries [J]. Journal of Power Sources, 2013, 222: 318-325.
[19] LAI Yan-qing, XU Ming, ZHANG Zhi-an, GAO Chun-hui, WANG Peng, YU Zi-yang. Optimized structure stability and electrochemical performance of LiNi0.8Co0.15Al0.05O2 by sputtering nanoscale ZnO film [J]. Journal of Power Sources, 2016, 309: 20-26.
[20] HUANG Ya-qun, HUANG Yun-hui, HU Xian-luo. Enhanced electrochemical performance of LiNi0.8Co0.15Al0.05O2 by nanoscale surface modification with Co3O4 [J]. Electrochimica Acta, 2017, 231: 294-299.
乙醇洗涤对LiNi0.8Co0.15Al0.05O2正极材料性能的影响
刘万民1,秦牡兰1,许 律1,易 苏1,邓继勇1,黄中华2
1. 湖南工程学院 化学化工学院,湘潭 411104;2. 湖南工程学院 机械工程学院,湘潭 411104
摘 要:采用乙醇溶剂对不同LiNi0.8Co0.15Al0.05O2正极材料进行洗涤。采用电感耦合等离子体原子发射光谱(ICP-AES)、傅里叶变换红外光谱(FTIR)、X射线衍射(XRD)、扫描电子显微术(SEM)、充放电测试和电化学阻抗谱(EIS)对样品的元素含量、结构、形貌和电化学性能进行表征。结果表明,乙醇洗涤能够有效去除新制备的LiNi0.8Co0.15Al0.05O2表面残留的LiOH/Li2O杂质,不会破坏材料的本体结构、表面形貌和电化学性能,使得材料具有更好的耐H2O和CO2腐蚀性。并且,使用乙醇立即对新制备的材料进行洗涤,再置于相对湿度80%的空气中储存3个月后,其放电比容量和循环性能相对于未洗涤样品得到大幅提升。
关键词:锂离子电池;LiNi0.8Co0.15Al0.05O2;乙醇洗涤;储存性能;电化学性能
(Edited by Bing YANG)
Foundation item: Projects (15B054, 17C0400) supported by the Scientific Research Fund of Hunan Provincial Education Department, China; Projects (2017JJ2060, 2015JJ2042) supported by the Natural Science Foundation of Hunan Province, China; Project (2014-207) supported by the Aid Program for Science and Technology Innovative Research Team in Higher Educational Instituions of Hunan Province, China
Corresponding author: Wan-min LIU; Tel: +86-13787101796; E-mail: william@hnie.edu.cn
DOI: 10.1016/S1003-6326(18)64805-8